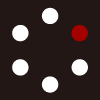
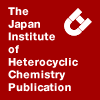
HETEROCYCLES
An International Journal for Reviews and Communications in Heterocyclic ChemistryWeb Edition ISSN: 1881-0942
Published online by The Japan Institute of Heterocyclic Chemistry
e-Journal
Full Text HTML
Received, 17th February, 2009, Accepted, 13th April, 2009, Published online, 14th April, 2009.
DOI: 10.3987/COM-09-11684
■ Dihydrochalcone Designed from Methylophiopogonanone B Strongly Inhibits Hypoxia-inducible Factor (HIF)-1α Activity
Mikio Fujii,* Kiyoshi Egawa, Yasuaki Hirai, Masato Kondo, Kotaro Fujii, Hidehiro Uekusa, Hiroyuki Akita, Kiyoshi Nose, Kazuo Toriizuka, and Yoshiteru Ida
School of Pharmaceutical Sciences, Toho University, 2-2-1, Miyama, Funabashi, Chiba 274-8510, Japan
Abstract
Inhibition of hypoxia-inducible factor (HIF)-1α activity of methylophiopogonanone B (1) and its derivatives were investigated. As the modification of the structure of 1, dihydrochalcone (11) was leaded and showed one order higher activity (IC50: 0.2 μg/mL) than methylophiopogonanone B (1) (IC50: 2.2 μg/mL).Angiogenesis is a key step during tumor progression, and the production of vascular endothelial growth factor (VEGF) plays central role during this step.1 Transcription of the VEGF gene is enhanced under hypoxic conditions and controlled primarily by hypoxia response factor-1 (HRF-1).2,3 Hypoxia-inducible factor (HIF)-1 is a heterodimeric protein comprising HIF-1α and HIF-1β, both of which are basic helix-loop-helix transcription factors.4 Whereas HIF-1β is constitutively expressed, HIF-1α is stabilized under condition of hypoxia and activates hypoxia-inducible genes such as those for erythropoietin5,6 and VEGF.2,7 In the presence of oxygen, HIF-1α is hydroxylated at specific proline residues and targeted for ubiquitination.8 Thus the level of HIF-1α is critical to the expression of VEGF. Inhibitor of VEGF production are expected to be chemotherapeutic agents for cancer.9,10 In previous studies, we established a high through-put assay system using a stable transformant of mammalian cells that has incorporated the luciferase gene under the control of hypoxia-response element.11 Over 800 compounds including natural and synthetic flavonoids were applied to the reporter assay and methylophiopogonanone B (1) (Figure 1) from Ophiopogonis tubers (Ophiopogon japonicus KER-GAWLER) was found to be a strong inhibitor of HIF-1α activity in hypoxia conditions.12,13 On the other hand, we established synthetic route for racemic 1 (rac-1) and found inhibition of the HIF-1α activity of synthetic rac-1 was as strong as natural 1.14 In this study, we report developing stronger inhibitor of HIF-1α activity by modification of the structure of 1.
Previously, we reported the synthesis of methylophiopogonanone B (rac-1) from acetophenone derivative 2.14 In this procedure, chalcone 3, dihydrochalcone 4 and homoisoflavanones (rac-5, rac-6 and rac-7) were also obtained as intermediates or by-products (Scheme 1).
The compounds, 2, 3, 4, rac-5, rac-6 and rac-7, were applied to the reporter-assay, and IC50 for the inhibition of HIF-1α activity and cell viability were estimated as shown in Table (entry 1-8). Among them, inhibition of the HIF-1α activity of rac-7 (IC50: 0.8 μg/mL) and 4 (IC50: 1.1 μg/mL) were comparatively stronger than that of rac-1 (IC50: 2.2 μg/mL). This suggests that dihydrochalcone 4 is a better leading compound for inhibition of the HIF-1α activity since the dihydrochalcone is synthesized much easier than homoisoflavanone.
Since dihydrochalcone 4 showed strong inhibition of the HIF-1α activity, next four dihydrochlacones shown in Scheme 2 were synthesized. In order to investigate the effects of C-methyl group on the A-ring to the activity, dihydrochalcones 8 and 9, which were replaced one or two C-methyl moieties on A-ring of 4 to hydrogen, respectively, were tested. However the activities of these compounds were much weaker than that of 4 (IC50 for 8: 5.8 μg/mL; IC50 for 9: >10.0 μg/mL). These results showed the importance of both of two C-methyl groups on A-ring of 4. On the other hand, dihydrochalcones 10 and 11 were tested to the reporter assay to investigate the effect of substituent at 4-position of B-ring of 4. While compound 10 (IC50: 7.0 μg/mL) showed weaker activity than 4, compound 11 was found to be effective to inhibit the HIF-1α activity. Inhibition of the HIF-1α activity of 11 (IC50: 0.2 μg/mL) showed one order higher than that of rac-1 (IC50: 2.2 μg/mL). Meanwhile, IC50 for structurally related chalcone 12 was >10.0 μg/mL.
Several information for structure–activity relationship of 1 were obtained from above results. Firstly, C-ring of 1 is supposed not to be necessary for the strong activity, since dihydrochalcones 4 show much higher activity than structurally similar homoisoflavanone rac-5. Next, two C-methyl groups on A-ring might be important considering from weaker activity of 8 and 9 than 4. Substituent on B-ring might be preferred smaller group since unsubstituted 10 (IC50: 0.2 μg/mL) was stronger inhibitor than 4 (IC50: 1.1 μg/mL) possessing methoxy group and 9 (IC50: >10.0 μg/mL) possessing dimethylamino group and rac-7 (IC50: 0.8 μg/mL) possessing hydroxyl group was weaker than rac-1 (IC50: 2.2 μg/mL) possessing methoxy group. Then B-ring is considered to be important to inhibit HIF-1α activity, since 2 (Scheme 1) did not show high activity.
In summary, reporter assay for inhibition of the HIF-1α activity was applied to methylophiopogonanone B (rac-1) and its derivatives and the activity of dihydrochalcone 11 possessing simple structure showed one order higher than that of rac-1. Considering from the results of the assay for dihydrochalcones 4, 10, and 11, HIF-1α inhibitory activity and cyototoxicity were strongly affected by kind of substituent on B ring. Further modification of 11, especially on B ring, for the purpose of reducing cytotoxicity and enhancing the inhibition of HIF-1α activity were underwent for discovery of new anticancer agent.
EXPERIMENTAL
Materials
Compound 8, 9, 10 and 11: These compounds were synthesized by condensation of the corresponding acetophenone derivatives and benzaldehyde derivatives as following the reported procedure.15 The 1H and 13C NMR of 7 and 8 were identical with those of reported value.15 10: 1H NMR: δ 2.13 (s, 3H), 2.14 (s, 3H), 3.20 (t, 2H, J = 7.6 Hz) 3.42 (t, 2H, J = 7.6 Hz), 3.66 (s, 3H), 3.73 (s, 3H), 7.16-7.30 (m, 5H), 13.00 (s, 1H). 13C NMR: δ 8.7, 9.2, 30.6, 44.6, 60.0, 61.7, 111.5, 115.3, 115.6, 126.0, 128.4 (2C), 128.4 (2C), 141.3, 158.8, 161.0, 163.5. IR ν: 2871 (br.), 1613, 1586, 1520, 1408, 1136, 1108 cm-1. EI-HR-MASS calcd for C21H27NO4: 357.1940. Found: 357.1950. 11: 1H NMR: δ 2.12 (s, 3H), 2.13 (s, 3H), 2.90 (s, 6H), 2.92 (t, 2H, J = 7.7 Hz) 3.37 (t, 2H, J = 7.7 Hz), 3.66 (s, 3H), 3.72 (s, 3H), 6.69 (d, 2H, J = 8.4 Hz), 7.10 (d, 2H, J = 8.4 Hz), 13.04 (s, 1H). 13C NMR: δ 8.7, 9.2, 29.8, 40.9 (2C), 45.1, 60.0, 61.7, 111.5, 113.0 (2C), 115.2, 115.5, 129.0 (2C), 148.4, 149.1, 158.8, 161.0, 163.4. IR ν: 2870 (br.), 1614, 1586, 1519, 1407, 1136, 1108 cm-1. EI-HR-MASS calcd for C19H22O4: 314.1518. Found: 314.1517.
Measurement of HIF-1α inhibitory activity.
Cell Culture: A stable transformant of CHO cells (clone A4-4) was established by the transfection of HIF-1-dependent luciferease (5XHRE/pGL3/VEGF/E1b) and neomycin-resistant genes as described previously.16
Methods: Cells were plated into 96-well tissue culture plates (Falcon) at a density of 1 X 104 cells/well, and treated with synthetic compounds 16 h later. They were incubated further 48 h under hypoxic conditions, and harvested for the determination of luciferase activity. The assay was carried out using a kit provided by Promega Corp. (Madison, WI, USA) following the manufacturer’s manual. The cell viability was estimated by the MTT method previously reported.16
ACKNOWLEDGEMENTS
We thank Mr. Takuya Genkai, Mr. Shintaro Koike and Mr. Kijyu Konno (Showa University) for their contribution to this research.
References
1. D. Shweiki, A. Itin, D. Soffer, and E. Keshet, Nature (London), 1992, 359, 843. CrossRef
2. J. A. Forsythe, B.-H. Jiang, N. V. Iyer, F. Agani, S. W. Leung, R. D. Koos, and G. L. Semenza, Mol. Cell. Biol., 1996, 16, 4604.
3. G. L. Semenza, Curr. Opin. Cell. Biol., 2001, 13, 167. CrossRef
4. G. L. Wang and G. L. Semenza, J. Biol. Chem., 1995, 270, 1230. CrossRef
5. G. L. Semenza, M. K. Nejelt, S. M. Chi, and S. E. Antonarakis, Proc. Natl. Acad. Sci. U.S.A., 1991, 88, 5680. CrossRef
6. I. Beck, R. Weinmann, and J. Caro, Blood, 1993, 82, 704.
7. G. L. Semenza, Genes. Dev., 2000, 14, 1983. CrossRef
8. M. Ivan, K. Kondo, H. Yang, W. Kim, J. Valiando, M. Ohh, A. Salic, J. M. Asara, W. S. Lane, and W. G. Kaelin, Jr., Scienece, 2001, 292, 464. CrossRef
9. T. Mustonen and K. Alitalo, J. Cell. Biol., 1995, 129, 895. CrossRef
10. K. H. Plate, G. Breier, B. Millauer, A Ullrich, and W. Risau, Cancer. Res., 1993, 53, 5822.
11. Y. Yamazaki, K. Egawa, K. Nose, S. Kunitomo, and T. Takeuchi, Biol. Pharm. Bull., 2003, 26, 417. CrossRef
12. T. Asano, T. Murayama, Y. Hirai, and J. Shoji, Chem. Pharm. Bull., 1993, 41, 391.
13. Y. Hasebe, K. Egawa, Y. Yamazaki, S. Kunimoto, Y. Hirai, Y. Ida, and K. Nose, Biol. Pharm. Bull., 2003, 26, 1379. CrossRef
14. M. Fujii, K. Egawa, Y. Hirai, M. Kondo, H. Akita, K. Nose, K. Toriizuka, and Y. Ida, Heterocycles, 2009, 78, 207. CrossRef
15. A. Tada, R. Kasai, T. Saitoh, and J. Shoji, Chem. Pharm. Bull., 1980, 28, 2039. CrossRef
16. K. Egawa, T. Yamori, C. Nosaka, S. Kunitomo, T. Takeuchi, and K. Nose, Biol. Pharm. Bull., 2000, 23, 1036. CrossRef