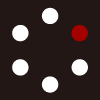
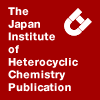
HETEROCYCLES
An International Journal for Reviews and Communications in Heterocyclic ChemistryWeb Edition ISSN: 1881-0942
Published online by The Japan Institute of Heterocyclic Chemistry
e-Journal
Full Text HTML
Received, 20th February, 2009, Accepted, 1st April, 2009, Published online, 2nd April, 2009.
DOI: 10.3987/REV-09-654
■ The Chemistry of 2H-Cyclohepta[b]furan-2-one: Synthesis, Transformation and Spectral Properties
Noboru Morita,* Kozo Toyota, and Shunji Ito
Department of Chemistry, Graduate School of Science, Tohoku University, Aoba-ku, Sendai 980-8578, Japan
Abstract
Overview of data, obtained in the last 60 years, concerning the synthetic methodology for 2H-cyclohepta[b]furan-2-one, their reactivity and mechanistic aspects, the synthetic potential for the extended π-electronic systems, and their spectral properties, are described.1. INTRODUCTION
The chemistry of 2H-cyclohepta[blfuran-2-one (1a) is important in connection with troponoid chemistry, fulvenoid chemistry and azulenoid chemistry. Since the first synthesis of 2H-cyclohepta[blfuran-2-ones by Nozoe and Seto was reported at 19531 during the investigation of reactivities in troponoid compounds, a lot of interesting discoveries about 2H-cyclohepta[b]furan-2-one have been reported. Therefore, we will described here about the synthetic methodology for 2H-cyclohepta[b]furan-2-ones, their reactivity, mechanistic aspects, and the other properties about synthetic potential for new extended π-electronic systems.
2. THE FIRST SYNTHESIS OF 2H-CYCLOHEPTA [b]FURAN-2-ONES
Nozoe and Seto and their co-workers found the first synthetic way of 2H-cyclohepta[b]furan-2-one (1a) which is a key precursor to azulenes starting from troponoids, during studying reactivity of the reactive troponoids (-which we call for 2-chlorotropone (2a), 2-methoxytropone (2b), and 2-tosyloxytropone (2c)) with active methylene compounds. To be concrete, 3-ethoxycarbonyl-2H-cyclohepta[b]furan-2-one (1b) and 3-aceyl-2H-cyclohepta[b]furan-2-one (1c) were prepared by the reaction of 2-chlorotropone with corresponding sodium salts of diethyl malonate and sodium ethyl acetoacetate, respectively. Therefore, these derivatives could be converted to unsubstituted 2H-cyclohepta[blfuran-2-one (1a) itself by hydrolysis and decarboxylation or deacetylation with concentrated sulfuric acid.
3. MECHANISTIC DETAILS IN REACTION OF TROPONOID WITH ACTIVE METHYLENE COMPOUNDS
The mechanism of these reactions may be similar. But there are some differences among the reactive troponoids. In case of the reaction of 2-chlorotropone with diethyl malonate, the reaction proceed according to path a (Scheme 2). The carbanion of the active methylene attacks at C-7. The hydrogen at C-7 shifts to C-2 in the initial intermediate. Then the oxygen of tropone attacks at ester carbonyl carbon and the elimination of ethanol gives 2H-cyclohepta[b]furan-2-one (1).
However, when malononitrile was used as an active methylene reagent, 3-cyano-2H-cyclohepta[b]furan-2-imine (3d, X=H) is obtained initially by according to a similar mechanism (path a). Imine group easily converts to carbonyl group by hydrolysis during treatment of the product. If 5-substituted 2-chlorotropone is used, either 5-substituted 3-cyano-2H-cyclohepta[b]furan-2-imine (3e) or the corresponding 2H-cyclohepta[b]furan-2-one is obtained.
If 5-substituted 2-methoxytropone is used as a substrate instead of 2-chlorotropone, diethyl malonate anion attacks at 2-position to give 6-substituted 3-ethoxycabonyl-2H-cyclohepta[b]furan-2-one as shown in Scheme 4 (path b).
In case of 2-tosyloxytropone (2d),2 the distribution of products are little complicated depend on the reaction conditions. Usually the reactions proceed as similar to those of 2-chloroptopone (path a). When sodium ethoxide is used as a base, the reaction of 2d with diethyl malonate gives 8-hydroxy-2H-cyclohepta[b]furane-2-one (1d) or furotropones (7). These products react with diazomethane to give both corresponding O-methylated products. The mechanism can be considered as follows. After nucleophilic attack of diethyl malonate anion, toluenesulfinate anion dissociates according to path c to give intermediate 4 as shown in Scheme 5. Compound 4 cyclized by dissociation of ethanol to give a mixture of 1d and 7. When malononitrile was used as a reagent, products 3f and 8 were obtained similarly.
Thus, a variety of 2H-cyclohepta[b]furan-2-ones can be prepared by the reaction of active troponoid compounds with active methylene reagents, although there are some troubles in case of 3- or 7- substituted troponoid compounds due to steric hindrance.
For examples, by using this procedure, Sato prepared methyl derivatives except 3- methyl-2H-cyclohepta[b]furan-2-one (13) and 8-methyl-2H-cyclohepta[b]furan-2-one (14) and their properties were made clear.3
4. SYNTHETIC WAY FOR 2H-CYCLOHEPTA [b]FURAN-2-ONES BY NUCLEOPHILIC ATTACK
Several other preparing methods for preparation of 2H-cyclohepta[b]furan-2-one and its derivatives were reported. The lithium salt of ethyl acetate also reacts with 2-chlorotropone to give ethyl 2-troponylacetate which is easily cyclized to 2H-cyclohepta[b]furan-2-one according to eliminate alcohol.4
Tropone itself is also shown to be useful in the preparation of 3-cyano-2H-cyclohepta[b]furan-2-imine (3d). Tropone undergoes nucleophilic attack at 2-position by anion of malononitrile. The oxygen of tropone attack to carbon of nitrile and subsequent hydrogen shits to give 2-amino-3-cyano-8H-cyclohepta[b]furan (15). It undergoes dehydrogenation with tetrachloro-1,2-benzoquinone to give ionic complex (16). The complex 16 is hydrolyzed to give 3-cyano-2H-cyclohepta[b]furan-2-imine (3d) [orange crystals, mp 143 ºC (decomp.)].5
5. SYNTHETIC WAY FOR 2H-CYCLOHEPTA [b]FURAN-2-ONE AND 2H-CYCLOHEPTA- [b]THIOPHEN-2-ONE BY USING CYCLOADDITIONS OF SEVEN-MEMBERED RINGS
In 1967, Ciabattoni and Anderson reported the reaction of tropone with dichloroketene as follows.6 Tropone reacts with dichloroketene which comes from the reaction of dichloroacetyl chloride with triethylamine to give a [2+8]π cycloadduct. But it cannot be isolated. It undergoes further elimination of hydrogen chloride with triethylamine to give 3-chloro-2H-cyclohepta[b]furan-2-one (1f) as yellow-orange needles of mp 179 – 180 °C. However, the yield was not so good (Scheme 8).
Machiguchi reported7 the reaction of tropothione (2’a) with chloroketene which is generated in situ from chloroacetyl chloride and triethylamine, in benzene at room temperature affords only a 1:2 adduct 17b in 68 % yield (deep orange leaflets, mp 226 °C). The formation of the adduct 17b is interpreted by three steps, initially [2+8]π cycloaddition, then dehydrohalogenation, to give 2H-cyclohepta[b]thiophen-2-one (17a). Finally, the compound 17a underwent electrophilic substitution to give 3-chloroacetyl-2H-cyclohepta[b]thiophen-2-one (17b). The expected initial product 17a can be obtained in 72% yield as red needles (mp 88 °C) when the reaction temperature is below -60 °C.
It is well known that tropylcarbonyl chloride (18) undergoes elimination of hydrogen chloride with triethylamine to produce 8-oxoheptafulvene (19).8
When 8-oxoheptafulvene occurred in the presence of tropone in refluxing benzene, a [2+8]π cycloadduct 20a is obtained as main product along with small amount of heptafulvalene (21a).9
The [2+8]π cycloadduct 20a converts to 3-cyclohexa-1,4-dienyl-2H-cyclohepta[b]-furan-2-one (1g) under the conditions by heating in DMSO. The methyne proton in seven-membered ring shifts to six-membered ring by 1,5-hydrogen shift. The dihydrobenzene can be oxidized with DDQ to give3-phenyl-2H-cyclohepta[b]furan-2-one (1h).10
Initially, we thought that the structure of thermal product was 1,3-cyclohexadiene derivative on the basis of 100 M Hz NMR data. Coupling constant between methine and methylene proton is large. But recently, on the basis of X-ray analysis, the structure of thermal product is established as 1,4-cyclohexadiene derivative 1g as shown in Figure 2.11 Both planes of 2H-cyclohepta[b]furan-2-one ring and cyclohexadiene ring are flat and intersect orthogonally each other. There are some difference among single bonds and double bonds in the 2Hcyclohepta[b]furan-2-one ring of 1g. Observed bond distances (Å) are O1-C2 1.407(2), C2-C3 1.431(2), C3-C10 1.374(2), C10-C4 1.43082), C4-C5 1.359(2), C5-C6 1.425(3), C6-C7 1.352 (3), C7-C8 1.424(3), C8-C9 1.351(2), C9-C10 1.443(2), C9-O1 1.369(2).
The similar products are obtained in the reactions of 2-methyltropone, 2-chlorotropone, and 2-acetoxy tropone with 8-oxoheptafulvene. But in the case of 2-methoxytropone very different reactivity is observed.12 2-Methoxytropone reacts with 8-oxoheptafulvene to give 4 products (18’, 20 – 22). The products 20c and 21c are similar to those of previous experiments. The other products are very different.
Reaction mechanism of 2-methoxytropone with 8-oxoheptafulvene is considered as follows. 1-Methoxyheptafulvalene (21c) is produced by [2+2]π cycloaddition and subsequent decarboxylation of β-lactone 23c. Norcaradiene type adduct 20c is produced by 1,7-shift of C-C bond in β-lactone 23c although there is a possibility of direct [2+8]π cycloaddition of 2-methoxytropone with 8-oxoheptafulvene (Scheme 14).
Especially the structure and occurring mechanism of bridged 2H-cylohepta[b]furan-2-one 22 are very interesting. The structure of bridged 6-bromo-2H-cylohepta[b]furan-2-one which is produced from 5-bromo-2-methoxytropone (2g) is firmly established on the basis of X-ray analysis (Figure 3).13 There are also some differences between single and double bond length in 2H-cylohepta[b]furan-2-one moiety which is flat.
On the basis of experimental observation using duterated 2-methoxytropons and some other substituted 2-methoxytropons, the mechanism of its formation is hypothesized to involve the [2+2]π cycloaddition, 1,7-shift of C-O bond of β-lactone, Cope rearrangement, and final elimination of methanol (Scheme 15).13
Furthermore, the reaction of 2-N,N-dimethyaminotropone (2h) with 8-oxoheptafulven also gives bridged 2H-cyclohepta[b]furan-2-one as a minor product. The main product is 3-phenyl-2H-cyclohepta[b]furan-2-one (1h) although the yield is low.14
However, if 2,7-dibromotropone (2i) is used as a substrate, 8-bromo-3-phenyl-2H-cyclohepta[b]furan-2-one (1i) can be obtained in good yield. The reaction of troponoid compounds with 8-oxoheptafulvene afford a variety of 2H-cyclohepta[b]furan-2-ones.14
TroponeFe(CO)3 24a (R=H) can be easily prepared by the reaction of tropone with ironcarbonyl reagents such as Fe2(CO)9, Fe3(CO)12, Fe(CO)5, benzalacetoneFe(CO)3, etc.15 TroponeFe(CO)3 itself reacts with 8-oxoheptafulvene to give heptafulvaleneFe(CO)3 as a single product.15g If there is a substituent at 2-position of tropone, its reactivity changes to give a [2+8]π cycloadduct. In case of complex 24f (R=Me), two [2+8]π cycloadducts such as 26f and 27f can be obtained.16
[2+8]π cycloadduct 26f of 8-oxoheptafulvene (19) to 2-methyltroponeFe(CO)3 24f is treated with ceric ammonium nitrate (CAN) in acetonitrile to give norcaradiene derivative 20f in 45% yield and 8-methyl-3-phenyl-2H-cyclohepta[b]furan-2-one (1j) in 11% yield. In the case of 26g, norcaradiene
derivative 20g and 8-t-butyl-3-phenyl-2H-cyclohepta[b]furan-2-one (1k) in 63% and, 36% yields, respectively.17
TroponeFe(CO)3 reacts with concentrated sulfuric acid to give a dienonium cation (27), Treatment of the cation with potassium carbonate and water give a hydroxyl compound 28 which is oxidized with the complex of chromium trioxide and pyridine to give 2,4-cyclohexadiene-1,6-dioneFe(CO)3 (29).18, 19 It is iron tricarbonyl complex of a keto form of β-tropolone. It can be also prepared directly by reaction of 3-hydroxytropone with Fe2(CO)9. This complex exhibits a variety of reactivities. It is very interesting that chloroacetate of 2,4-cyclohexa-1,6-dioneFe(CO)3 (30) easily reacts with 8-oxoheptafulvene to give a product 31 as a keto form of 2-hydroxyheptafulvaleneFe(CO)3 by one pot reaction. Because the haloacetate, is susceptible to hydrolysis, chloroacetyl group easily eliminates from chloroacetyloxyheptafulvaleneFe(CO)3. 5- Cycloheptatrienlidene-cyloheptadiene-1-one (31) is treated with trimethylamine N-oxide followed by separation with silica gel column using dichloromethane as an eluent to give 3-chloro-2H-cyclohepta[b]furan-2-one (1f) (about 30% yield).20
Recently, we have also found other new synthetic method for 2H-cyclohepta[b]furan-2-one, 3- and 6-chloro-2H-cyclohepta[b]furan-2-one during the investigation for cycloadducts of cycloheptatriene with dichloroketene as follows.20 Dichloroketene which was prepared by the reaction of trichloroacetyl chloride with activated zinc, undergoes [2+2]π cycloaddition to cyloheptatriene. The [2+2]π cyclobutanone adduct could be converted to γ-lactone by Baeyer–Villiger oxidation. Dehydrogen chloride with lithium chloride at ca. 120 °C in DMF easily converts to 2H-cyclohepta[b]furan-2-one (Scheme 21).
The γ-lactone 34 undergoes epoxidation with m-CPBA to give 35 which was directly obtained from [2+2]π cycloadduct 33 by oxidation with m-CPBA. The epoxide 35 is treated with LiCl at 140 °C to give a mixture of 3-chloro-2H-cyclohepta[b]furan-2one (1f) and 6-chloro-2H-cyclohepta[b]furan-2one (1l). Chloro-2H-cyclohepta[b]furan-2ones could be obtained by three steps from cycloheptatriene (Scheme 22).
6. SYNTHETIC WAY FOR 2H-CYCLOHEPTA [b]FURAN-2-ONE FROM HEPTAFULVENE AND CYCLOHEPTATRIENES BY PHOTO-OXYGENATION
Next method for preparation of 2H-cyclohepta[b]furan-2-ones is photo-sensitized oxygenation of heptafulvenes or cycloheptatrienes.21 When a solution of 8-cyanoheptafulvene (36) in acetone is irradiated in the presence of a sensitizer (Rose bengal) with a 100 W high-pressure mercury lamp through a water cooled pyrex filter under oxygen, it absorbed one mole of oxygen within two hours to give a (1:1) mixture of epidioxiede 37 and 38 in 85% yield as colorless crystals which detonates at 106 ºC in a capillary tube. These epidioxides are treated with triethylamine to give 6-hydroxy-3-cyano-2H-cyclohepta[b]furan-2-imine (3e) in 90% yield.
This reaction is applicable to 8-methoxycarbonylheptafulvene (39) and 8,8-dimethoxycarbon- ylheptafulvene (40) to give corresponding 2H-cyclohepta[b]furan-2-one 1a and 1b’. Epidioxide is converted to 2H-cyclohepta[b]furan-2-one derivatives without hydroxyl group at C-6 using thiourea instead of trietylamine.
These photo-sensitized reactions are utilized to the synthesis of 1a and 3d from cycloheptatriene derivatives 41 and 42 as shown in Scheme 25.
7. SYNTHETIC WAY FOR 2H-CYCLOHEPTA [b]FURAN-2-ONE FROM PHENYL PROPARGYL ETHER BY THE FLASH VACUUM PYROLYSIS
Trahanovsky has found general synthetic way for 2H-cyclohepta[b]furan-2-ones by the flash vacuum pyrolysis (FVP) of phenyl propargyl ethers at 650 °C and ~10-4 Torr.22a This method is applicable for methyl substituted phenyl propargyl ethers. On the basis of methyl groups position of starting phenylpropiolate and product 2H-cyclohepta[b]furan-2-ones the FVP mechanism is shown in Scheme 26.
The acetylenic hydrogen of 45 undergoes 1,2-hydrogen shift to give intermediate methylenecarbene 46. The methylenecarbene 46 undergoes intramolecular cycloaddition to give norcaradine intermediate 47. It undergoes valence bond isomerization to give 2H-cyclohepta[b]furan-2-one.22d
By this FVP method, 8-methyl-2H-cyclohepta[b]furan-2-one (mp 116 – 117 °C), which cannot be prepared by Nozoe and Seto’s synthetic way, can be obtained as a main product (38 – 45%) along with 4-methyl-2H-cyclohepta[b]furan-2-one (13 – 15%). The isomeric lactones are separated by column chromatography.
4,8-Dimethyl-2H-cyclohepta[b]furan-2-one (49) is prepared by the FVP of 2,6-dimethylphenylpropiolate. 2,4,6-Trimethyl-phenylpropiolate gives 4,6,8-trimethyl-2H-cyclohepta[b]furan-2-one (50) by the FVP.
Indane derivative also underwent pylolysis to give a mixture of 2H-cyclohepta[b]furan-2ones condensed with five-membered ring in the same 20% yields, respectively. This observation also is consistent with the mechanism shown in Scheme 26.
More polymethylated 2H-cyclohepta[b]furan-2ones (54 – 56) have also been prepared from corresponding polymethyl phenylpropiolate by dynamic gas phase thermo-isomerization. 4,5,6,7,8-Pentamethyl-2H-cyclohepta[b]furan-2-one reveals a slight deviation from planarity on the basis of X-ray analysis and calculation.22b-d
8. SYNTHETIC WAY FOR 2H-CYCLOHEPTA[b]FURAN-2-ONE BY WAY OF CYCLOHEPTAOXAPHOSPHOLE
Active troponoid reacts with a variety of ylids to give cycloheptaoxaphosphole. Kawamoto and co-workers characterized a “bonding betaines” as the most serious resonance hybrid forms as shown in Scheme 29.23
In the reaction with phenyl isocyanate the cycloheptaoxaphospholes act as an ylide to give corresponding 2H-cyclohepta[b]furan-2-ones (1a, 1b, and 1d) and 2H-cyclohepta[b]furan-2-imines (3e, 3f, and 3g) accompanied by the liberation of triphenylphosphine N-phenylimine and triphenylphosphinre oxide. The product 3e is a mixture of (E) and (Z). Products 3f and 3g are (Z). The cycloheptaoxaphosphole 57a reacts with phenyl isothiocyanate to give 2-(N-phenylamino)cyclohepta[b]thiophen-4- one (59) and a mixture (E)-3e and (Z)-3e.
The cycloheptaoxaphosphole 57b reacts with phenyl isothiocyanate to give ethoxycarbonylcyclohepta[b]furan-2-thione (58b) and (Z)-imine 3f in 12% and 4% yield, respectively. In the case of 57d, imine 3g is a main product and a small amount of cyclohepta[b]furan-2-thione 58d produced as a by-product. Furthermore, cycloheptaoxaphospholes 57 react with N-methoxyphenylsuccinimide to give [2+8]π adduct ( 60) and[2+4]π cycloadduct (60’) similar to 2H-cyclohepta[b]furan-2-one.
9. ORGANOMETALLIC COMPOUNDS RELATE TO 2H-CYCLOHEPTA [b]FURAN-2-ONE
There is not an organometallic compound in which 2H-cyclohepta[b]furan-2-one moiety coordinates to metal until now. But two unique organometalic compounds 24, 25 are reported.
Tropone reacts with metal carbonyl reagents to give corresponding troponeiron carbonyls. However, tropone reacts with decacarbonyl dimanganese to give tricarbonyl-1-syn-(1’,2’-dihydro-2’-oxo-1’- oxa-azulen-3’-yl)η5-pentadienylmanganese as a main product.24
It is a purple crystalline solid whose structure is determined by X-ray analysis as shown in Figure 6. Mangane coordinates dienyl part. It is interesting that this 2H-cyclohepta[b]furan-2-one portion exhibits characteristic bond alternation although bond lengths of dienyl porttion is almost same.24
The other example is a compound which is replaced carbonyl group of 2H-cyclohepta[b]furan-2-one with metalcarbonyl group. The anions derived from methylmethoxycarbene complexes of chromium and tungsten as nucleophiles react with tropone and 2-substituted tropones to give corresponding oxaazulenylidene complexes,25 although their yields are very low as shown in Scheme 32.
On the basis of spectral data, these oxaazulenylidene metal complexes 64 and 65 undergo the perturbation of the canonical formulas B and C similar to 2H-cyclohepta[b]furan-2-one.
Pentacarbonyl(1-oxazulen-2-ylidene)chromium (64a) reacts with S8 in refluxing THF to give 1-oxa-2-azulenethione (58a) as a red crystals (mp 181 – 182 ºC) in 82% yield.
10. Structural and Spectroscopic Properties of 2H-cyclohepta[b]furan-2-one
2H-Cyclohepta[b]furan-2-one is obtained as orange needle crystals (mp 69 – 70 °C). Molecular Structure of 2H-cyclohepta[b]furan-2-one is investigated by X-ray analysis.26 As a result, 2H-cyclohepta[b]furan-2-ones possess an almost planar structure.
It is recognized clearly that a larger and a shorter C-C bonds are disposed alternately. The mean values for the lager and shorter C-C bonds are 1.409 and 1.357 Å with standard deviations of 0.010 Å respectively. The difference between these two values is highly significant, and this fact favors the conventional chemical formula I shown in Fig. 9. However, the mean value of the larger C-C bonds and those of two C-O bonds in the five-membered ring (with standard deviation of 0.010 Å) are significantly different from their pure single bond lengths respectively. Thus, in order to interpret the bond distances more quantitatively, it is necessary to take into account the many resonance structures as shown in Figure 9.
Considering the resonance structures given above, it seems that the seven-membered ring itself is somewhat positively charged. 2H-Cyclohepta[b]furan-2-one is expected to undergo nucleophilic attack of active methylene at C8a, regiospecifically due to the contribution of 1D. This is justified by the large dipole moment (5.64 D) of this molecule and by some chemical evidences.27
The most intense bands for carbonyl region in the IR spectrum (KBr) appears at1748 cm-1. Absorptions of the longest wave length (MeOH) appear at 427 nm (sh, ε = 1980), 453 nm (sh, 780), and 488 nm (sh, 190), assigned as n→π∗ transition. The peaks at 373 nm (14660) and 387 nm (14700) are assigned as π→π∗ (2). The peaks at 223 nm (14350) and 251 nm (23150) are assigned as π→π∗ (1).22b The hydrogen chemical shifts of 2H-cyclohepta[b]furan-2-one by using 1H (600 MHz) and 13C (150 MHz) NMR spectrum (CDCl3) can be assigned completely as follows. δ 7.29 (dd, J=11.2, 1.1 Hz, H-4), 7.03 (ddd, J=11.2. 8.6, 0.7 Hz, H-5), 6.99 (ddd, J=10.8, 9.1, 0.7 Hz, H-7), 6.94 (ddd, J=9.1, 1.3, 1.1 Hz, H-8), 6.81 (ddt, J=10.8, 8.6, 1.1 Hz, H-6), 5.75 (d, J=1.3 Hz, H-3),13C NMR (CDCl3), δ 169.44 (C-2), 158.27, 153.10, 135.31 (C-5), 132.44 (C-7), 130.40 (C-6), 127.78 (C-4), 113.75 (C-8), 98.64 (C-3).22b Thease data are very useful when we will investigate the substitution effects for new 2H-cyclohepta[b]furan-2-one derivatives.
11. THE FORMATION OF AZULENES BY NUCLEOPHILIC ADDITION OF 2H-CYCLOHEPTA[blFURAN-2-ONE
2H-Cyclohepta[blfuran-2-ones, such as 3-ethoxycarbonyl-2H-cyclohepta[b]furan-2-one and 3-aceyl-2H-cyclohepta[b]furan-2-one reacted easily with malononitrile, cyanoacetamide, ethyl cyanoacetate, and diethyl malonate in the presence of NaOEt or t-butylamine at room temperature or under cooling with ice-water, giving the corresponding 1,2,3-trisubstituted azulene derivatives, respectively.28
The carbanions, R2--CH-R3, being produced from malononitrile, cyanoacetamide, ethyl cyanoacetate or diethylmalonate, attack 2H-cyclohepta[b]furan-2-ones at the 8a-position, regioselectively and the lactone ring opens to give a heptafulvene-type intermediate which should exist in the tautomers 62 and 63. The position at which the carbanions attack is confirmed from the observation on the formation of azulene derivatives from 2H-cyclohepta[b]furan-2-ones bearing the substituent at the seven-membered ring.
12. REACTION OF 2H-CYCLOHEPTA[b]FULAN-2-ONE WITH ENAMINES, ETHERS, OTHER ALKENE, AND ALKYNE
Yasunami and Takase found 2H-cycylohepta[b]furan-2-one reacts with enamines to give azulenes by the [8+2]π cycloaddition, removable of carbon dioxide and deamination. By this procedure, it is possible to introduce regiospecifically a expected functional group in five membered ring of azulene.29
As there is the review of this reaction in1981 by Yasunami,30 the preparation of new azulenes condenced with π-electronic systems by using this procedure will be described. as shown in Scheme 37-39. 1-Pyrrolidylacenaphtylene (73a) or its derivative (73b) reacts with 1a to give corresponding azuleno[4,5-a]acenaphthylene 74a or 74b. Compound 74a reacts further with dimethyl acetylenedicarboxylate (77b) to give dimethyl acenaphthyleno[1,2-d]heptalene-8,9-dicarboxylate (76).31
The compound 80 is prepared by the reaction of 2H-cyclohepta[b]furan-2-one with the enamine 79 as a key compound. Compound 80 undergoes trifluoroacetylation and bromination to give 81. After the reaction of 80 with KOH, methylation with diazomethane was carried out to give 82. Oxidation of 82 with DDQ and hydrolysis with KOH and decarboxylation in CF3CO2H gave 2,7-methanocyclodeca[a]azulene (83). The NMR spectrum of 2,7-methanocyclodeca[a]azulene (83).32 Its NMR spectrum revealed that the double bond of methano[10]annulene moiety are delocalized and azulene moiety indicate a bond-length alternation.
The cata condenced nonalternant hydrocarbon of new azulenazulenes 89a and 89b have been synthesized by combination of the reaction of 2H-cycylohepta[b]furan-2-one with enamines 84 and the reaction of acetylene derivatives (77a and 77b) with enamines 86 according to Scheme 39. Methyl azuleno[1,2-b]azulene-2-carboxylate during the reaction of 88b, methoxy carbonyl group shifted to 89b. Dimethyl azuleno[1,2-b]azulene-2,4-dicarboxylate.33
Nozoe, Wakabayashi and co-workers found that 2H-cyclohepta[b]furan-2-one reacts with enol ethers instead of enamines to give corresponding azulens by [2+8]π cycloaddition and followed by decarboxylation and elimination of alcohol. Sometimes, during this reaction, [2+4]π cycloaddition as a side reaction is observed. This experiments carried out as follows, a mixture of 2H-cyclohepta[b]furan-2-one and 3 – 5 equivalent of enol ethers are heated in aprotic solvents such as THF, acetonitrile, or toluene at 160 – 190 °C in a Pyrex sealed tube for 20 – 40 h to give deep-colored azulene 72 as a main product as shown in Scheme 40.34
Instead of enolethers, trialkyl orthoformates can be applicable for preparation of 2-alkyloxyazulenes 93, because it can be converted to ketene acetal 92 by heating. The ketene acetal 92 react with 1 to give 2-alchoxyazulene 93 (Scheme 41).
2H-Cycylohepta[b]furan-2-one reacts with electron deficient olefins such as 4-phenyl-1,2,4-triazoline-3,5-dione (PTAD) and dimethyl acetylenedicarboxylate (DMAD) to give a [4+2]π cycloadduct and /or a [8+2]π cycloadduct.
2H-Cycylohepta[b]furan-2-one reacts with PTAD at room temperature to give [4+2]π cycloadduct 95 in a 66% yield as a single product. On the other hand, reaction of 2H-cycylohepta[b]furan-2-one with excess amount of dimethyl acetylenedicarboxylate (DMAD) is carried out under reflux in o-xylene to give a [4+2]π cycloadduct 96 (71%) and azulene derivative 98 (9%) in a ratio of 7:1. The azulene derivative is probably derived from [8+2]π cycloaddition followed by decarboxylation as in the enamine reaction.35
1,3-Butadiene derivatives which are nonpolar olefins react with 2H-cycylohepta[b]furan-2-one under similar reaction condition to enamines to give heptafulvene (or dihydroazulene) derivatives in good yields.36
Methyl 2H-cylohepta[b]furan-2-one-3-carboxylate reacts with 6,6-dimethylfulvene by [4+2]π cycloaddition in ethanol refluxed preferably. In refluxing xylene, product 103 is obtained as a sole product. It is produced by [8+2]π cycloaddition and decarboxylation. The adduct 102 is heating to give a mixture of retro Diels-Alder reaction product 1b’ and 101 along with 103.37
When the complex 103 is treated with phosphoric acid, it underwent proton shift to give azulene derivative 105.
Coefficients and energy levels of molecular orbitals (NHOMO, HOMO, LUMO, and NLUMO) of 2H-cyclohepta[b]furan-2-one are as follows (Figure 10). The periselectivity of [2+8]π and [2+4]π cycloadditions is discussed on the basis of these data. 22c, 37a, 38, 39
13. ELECTROPHILIC SUBSTITUTION OF 2H-CYCLOHEPTA[b]FURAN-2-ONE
Because 2H-cyclohepta[b]furan-2-one is one of aromatic compounds, there is a possibility to undergo electrophilic aromatic substitution. Initially, bromination with bromine, nitration with fuming nitric acid in concentrated sulfuric acid, and acylation with acetic anhydride in the presence of tin tetrachloride are explored.
Thease reactions occur at 3-position of 1a regiospecifically, in good yields.1d, 40 After them, Nozoe and co-worker have investigated the reaction of tropyl ethers with various 1-oxaazulan-2-one to give corresponding 3-tropyl-2H-cyclohepta[b]furan-2-ones.41a 3-Formyl-2H-cyclohepta[b]furan-2-one is obtained in 63% yield by Vilsmeier reaction of 1a.41b
It is well known42 that pyridine react with tifluoromethanesulfonic anhydride to give 1-trifluoromethanesulfonylpyridinium trifluoromethanesulfonate (TPT) which is used as a reagent for trifuloromethanesulfonation of hydroxyl group such as phenol. Recently we found this reagent acts as an electrophile in the reaction of azulene to give 1-(N-trifuluoromethanesulfonyl-dihydropyridyl)azulene. It is easily remove trifluoromethanesulfinic acid by potassium hydroxide to give 1-pyridyl-azulene. This reaction is applicable for 2H-cyclohepta[b]furan-2-one to give 3-(4-pyridyl)-2H-cyclohepta[b]furan-2-one.43
The reaction of triflic anhydride with dimethylsulfoxide leads to formation of dimethyl (trifluoromethanesulfonyloxy)sulfonium trifluoromethanesulfonate, “dimethyl sulfide ditriflate” (DMSD). It is also a good electrophile for benzenoid compound to give aryldimethylsulfonium trifluoeomethanesulfonate.44 In case of 2H-cyclohepta[b]furan-2-one and 5-isoprpylderivative 1u, corresponding (2-oxo-2H-cyclohepta[b]furan-3-yl)dimethylsulfonium trifluoromethanesulfonates (1s and 1v) are obtained.45 They are treated with diethylamine to give 3-methylthio-2H-cyclohepta[b]furane-2-one (1w) and 5-isopropyl-3-methylthio-2H-cyclohepta[b]furane-2-one (1x).
These methylthio derivatives 1w and 1x are oxidized with m-CPBA to give 3-methylsulfinyl-2H-cyclohepta[b]furan-2-one (1y) and isopropyl derivative 1z.
3-Methylsulfinyl-2H-cyclohepta[b]furane-2-one (1y) is heated at ca. 40 ºC to give 3,3’-bi(2-oxo-2H-cyclohepta[b]furanyl) (107). Its reaction is carried out -80 C to give bis(2-oxo-2H-cyclohepta[b]furan-3-yl)sulfide (108).
Recently, we reported 2-hydroxyazulene reacts with trifluoromethanesulphonyl chloride in the presence of pyridine to give 1,3-dichloro-2-hydroxyazulene.46a On the basis of this observation, we found new haloganation with trifluoromethanesulphonyl chloride and halogenide ion. For example, azulene reacts with tetrabuthylammmonium bromide and trifluoromethanesulphonyl chloride to give 1-bromoazulene and/or 1,3-dibromoazulene. Under similar conditions, 2H-cyclohepta[b]furan-2-one gives 3-bromo-2H-cyclohepta[b]furan-2-one (1n) (Scheme 50). By the combination of trifluoromethanesulphonyl chloride and potassium iodide, 3-iodo-2H-cyclohepta[b]furan-2-ones (1o) is obtained in 88% yield. 2H-Cyclohepta[b]furan-2-one also undergoes halogenations with halosuccinimide.46b
14. CYCLOADDITIONS OF METHANO[11]ANNULENONES WITH DICHLORO- AND CHLOROKETENES. PREPARATION OF 2H-METHANOCYCLOUNDECA[b]FURAN-2-ONE RING SYSTEMS
There are a few 2H-cyclohepta[b]furan-2-one analogues. One of them is 2H-methanocycloundeca[b]furan-2-one. It is prepared from methano[11]annulenones by Nitta and co-workers.47 Methano[11]annulenones reacted with dichloroketene by [2+2]π cycloaddition and subsequent elimination of hydrogen chloride to give two products 110 and 111.
The chlorine of 110 can be removed by the treatment of NaI in the trifluoroacetic acid.
Furthermore, they can convert them to azulene analogous 114 by the [2+12]π cycloaddition with dimethyl acetylendicarboxylate.
8H-Cyclohepta[c]tropolone (115) which is prepared from azulene48 reacts with tosyl chloride and dimethyl malonate in the presence of sodium methoxide by Nozoe’s method (70%), to give 8H- heptaleno[b]furan-2-one (117) as orange crystals.49 This derivative will have potentiality as a synthetic key intermediate for a lot of compounds containing 2H-cyclohepta[b]furan-2-one skeleton.
15. THE ROLE OF 2H-CYCLOHEPTA[b]FURAN-2-ONE IN THE CHEMISTRY OF NATURAL PRODUCTS
Two derivatives (Lettucenine A. and Malophylidin) of 2H-cyclohepta[b]furan-2-one are found in plant.50
Named lettucenin A has been isolated from leaves of the lettus (Lactuca sativa var. capitata, compositae). Isolated yield from the dried leaves is 0.00084% yields. Lettucenine A is the first guaianolide phytoalexin containing a unique 2H-cyclohepta[b]furan-2-one ring system. Lettucenine A completely inhibits spore germination of Ceratocystis fimbriataat concentrations of 2 μg/ml.
An isomeric extended azulenequinone malophylidin (119) is isolated from the root of Ferula Malacophylla by Baginov et al.51 During the isolation of violet pigment linderaazulene from gorgonian Paramuricea Chamaeleon. Alpertunga et al. 52 detected a small amount of yellow pigment, which was a photo oxidation product 121 formed by exposing an ethanol solution of linderaazulene to direct sunlight for six days which is an isomer of malophylidin.51
The 2H-cyclohepta[b]furane derivative 123 have been synthesized from the antimitotic agent colchicines (122) which is one of well-known natural products, by Nozoe’s method as shown in the Scheme 56.53
As an example of availability of 2H-cyclohepta[b]furan-2-ones for total syntheses of natural products, Ando and co-workers reported synthesis of bioactive molecules Hymenolin and Parthenin used for 8-hydroxy-6-methyl-2-oxo-2H-cyclohepta[b]furan-3-carboxylate (124) as a key intermediate (Scheme 57).54
Wakabayashi and co-workers have been investigating on cytotoxic activity against human oral tumor cell lines and inhibition of LPS-stimulated NO production in mouse macrophage-like cells by some 2H-cyclohepta[b]furan-2-ones such as 1b, 1d, and 1d’. However, they cannot get a good result until now.55
16. UTILIZATION OF 2H-CYCLOHEPTA[b]FURAN-2-ONE AS FUNCTIONAL GROUPS
In the development of organic redox chemistry, 2-oxa-2H-cyclohepta[b]furan-3-yl group shows electron donating character and contributes to make reversible multistage redox systems as follows.
Tropylium ion, one of the most stable carbonium ion, is known to react with various anionons such as active methylene compounds and aryl compounds such as phenol and azulene. The reaction can also be carried out conveniently using tropyl ethers, in the presence of catalytic amounts of the tropyliun ion or acids, in place of tropylium salts. The 3-position of 1a is expected to be capable of electrophilic substitution with tropylium ion to give 3-(cycloheptatrien-7-yl)-2H-cyclohepta[b]furan-2-one (1p) as mentioned previously. The methine hydrogen of cycloheptatriene ring undergoes 1,5-shift thermally. Then, hydride removes by DDQ and anion-exchange reaction with aq, 42% HBF4 gives (2-oxo-2H-cyclohepta[b]furan-3-yl)tropyliumBF4- (129). From 2H-cyclohepta[b]thiophen-2-one, (2-oxo-2H-cyclohepta[b]thiophen-3-yl)tropyliumBF4- (132) [dark brown needles mp 170 –171 ºC] can also be easily obtained. The pKR+ values of these cations (129 and 132) are 3.8 and 3.2, respectively. These values are lower than that (3.9) of tropylium ion.
The averaged chemical shifts of seven–membered ring moiety in 1H NMR and 13C NMR are (8.90 and 152.7 for 129, 9.00 and 155.0 for 132. These chemical shifts appear at higher field compared with tropylium ion (9.26 ppm and 156.2 ppm).56 The ring protons of 2H-cyclohepta[b]furan-2-one moiety of 129 and 132 appear at lower fields compare with that of 1a and 17a.
The connecting at 3-position of 2H-cycylohepta[b]furan-2-one and 2H-cyclohepta[b]thiophen-2-one connect with tropylium cation works decrease the pKR+ value due to increasing the attacking places of hydroxide ion by contribution of 128B and 128C structures.
Nitta and co-worker57a have prepared tris(2-oxo-2H-cyclohepta[b]furan-3-yl)methyl cation (134) in excellent yields, starting from 1a by the electrophilic substitution with trimethyl orthoformate in a solution of trifluoromethanesulfonic acid and dichloromethane, and followed by oxidation with DDQ and treatment with HPF6. Its pKR+ value is 9.7. 2-Oxo-2H-cyclohepta[b]furan-3-yl group highly stabilized methyl cation. Its effectiveness is smaller than that of azulen-1-yl group (pKR+ is 11.3, E1red – 0.78 V, E2red –1.56 V, CH3CN).
The reduction potentials of 134 determined by cyclic voluammetry (CV) in CH3CN are E1red – 0.31 V and E2red – 0.95 V.
Bis(2-oxo-2H-cyclohepta[b]furan-3-yl)methane (137) are prepared by the reaction of 1a with paraform- aldehyde quantitatively. It was oxidized with DDQ to give a hydroquinone salt of bis(2-oxo-2H- cyclohepta[b]furan-3-yl)methyl cation and exchange the counter anion using HBF4 to give 138. 57b The longest wavelength absorption maxima of 138 appear 603 nm (log ε, 4.73). The pKR+ value of 138 is 2.6. The stabilization effect is not enough.
The reduction wave in cyclic voltammetry (CV) of 138 is – 0.27 V and irreversible. This observation expects to dimerize the radical species from 138 during CV measurement. It is treated with Zn to give dimer 139 along with small amount of 137. It is easily reduced with NaBH4 to give 137 in 92% yield.
Bis(2-oxo-2H-cyclohepta[b]furan-3-yl)phenylmethyl cations58 (143a-e) were prepared by the electrophilic substitution of 1a with 141c or its derivatives and oxidation with DDQ and treatment with HPF6. The pKR+ values of cations 143a-e depend on the substituents on the phenyl group. The pKR+ values of 143a-e are determined as shown in Table 1. In order to clarify the stabilizing effect of substituents in benzyl cations 143a-e, related radicals and anion species, they studied the synthesis and properties of bis(2-oxo-2H-cyclohepta[b]furan-3-yl)phenylmethyl cations. The characteristic absorption bands of the counter ion PF6- are observed at 838-845 cm-1 in the IR spectra of 143a-e. The longest wavelength absorption maxima of 143a-e in CH3CN are shown in Table 1. The spectrum of 143a shows remarkable difference. There are two big absorption maxima at 671 nm and 575 nm. Average of the two wave lengths is 623 nm which is similar to the longest wavelength absorption maxima (621 nm) of the other cations (143b-e). This observation could be the contribution of qinodimethane type structure similar to compound 156 which will be described later.
The synthetic method for 1,3- and 1,4-bis[bis(2-oxo-2H-cyclohepta[b]furan-3-yl)methyliumyl]benzenes are based on a single and stepwise TFA-catalyzed electrophilic aromatic substitution on 1a with isophthalaldehyde and terephthalaldehyde to afford the corresponding 1,3- and1,4-dimethylbenzene derivatives, followed by oxidative hydrogen abstraction with DDQ, and subsequent exchange of the counter-anion by using aq. HPF6 solution. In spite of the dicationic nature of 144 and 145, they exhibited high stability with large pKR+ values 9.3 and 9.0 due to the stabilizing effect of the 2-oxo-2H-cyclohepta[b]furan-3-yl units, however, we could not determine pKR+ and pKR++ values separately. The electrochemical reduction of the cation 144 exhibits reversible four waves at – 0.04 V, – 0.34 V, – 1.06 V, and – 1.34 V. The electrochemical reduction of the cation 145 exhibits reversible two waves at – 0.33 V and – 1.05 V.
Bis(2-oxo-2Hcyclohepta[b]furan-3-yl)methylimbenzene(phenyl)methyl cations 144 and 145 are stablized by 2-oxo-2H-cyclohepta[b]furan-3-yl units.
The reaction of 1,3,5-triformylbenzene with six molar equivalent amounts of 1a in CH2Cl2- trifluoroacetic acid (5:1) at rt for 48 h afforded 1,3,5-tris[bis(2-oxo-2H-cyclo- hepta[b]furan-3-ylmethyl)benzene in 68% yield.59b The oxidative hydrogen abstraction of 1,3,5-tris[bis(2-oxo-2H-cyclohepta[b]furan-3- yl)methyl]benzene with DDQ in CH2Cl2 at rt for 1 h, followed by treatment with aqueous 42% HBF4 in Ac2O, afforded crystals of stable 1,3,5-tris[bis(2-oxo-2H-cyclohepta[b]furan-3-yl)methyliumyl]benzene tris(tetrafluoroborate) (146) in 89% yield. The pKR+++ and pKR+ are 9.0 and 6.4. The longest wavelength absorption maximun of trication 146 is 615 nm.
The reduction potentials of trications are determined by cyclic volutammetry (CV) in CH3CN. The only two reduction waves of E3red and E6red observed at – 0.30 V and – 1.13 V, respectively.
The highly polarized compounds, quinonemethides,60 4-Hydroxyphenyl-bis(2-oxo-2H-cyclohepta[b]- furan-3-yl)methane and 3,5-di-tert-butyl-4-hydroxyphenyl-bis(2-oxo-2H-cyclohepta[b]furan-3-yl)- methane prepared by using described procedure previously. The pKa values of the conjugated acids of 147and 148 are 4,2 and <0, respectively.
The reduction and oxidations of quinonemethides 147 and 148 determined by cyclic volutammetry (CV) in CH3CN are E1red – 1.07 V, E2red – 1.44 V, E3red – 1.66 V, E1ox (+0.78 V), E2ox (+1.47 V) of compound 147. E1red – 1.24 V, E2red – 1.56 V, E1ox +0.74 V, E2ox (+1.09 V) were observed in compound 148. These reductions exhibit reversible but oxidations do not exhibit reversible except E1ox of 148.
The preparation of 7,7-bis(2-oxo-2H-cyclohepta[b]furan-3-yl)-8,8-dicyano-1,4-qunoinodimethane (151) was prepared by the TFA-catalized electrophilic substitution of 1a with 4-(dicyanomethyl)benzaldehyde (149) and subsequent oxidation with DDQ and treatment of triethylamine in good yield. 61 The longest wave length absorption maxima of 151 in CH3CN appears at 663 nm and 531 nm. But the longest wave length absorption maxima of 151 changes to 621 nm by addition of trifluoroacetic acid. The compound 151 become bis(2-oxo-2H-cyclohepta[b]furan-3-yl)phenylmethyl cations by protonization. Reduction waves (reversible) and oxidation waves (irreversible) of 151 by CV spectrum appear E1red – 0.70 V, E2red – 1.28, E1ox (+0.51 V), and E2ox (+1.55 V).
17. CONCLUSIONS
We have described the variety of synthetic methods for 2H-cyclohepta[b]furan-2-one and its derivatives. Moreover, a variety of methodologies for functionalization of these molecules using electrophilic substitution or nucleophilic reactions and chcloaddition and so on are described here. Now investigation towards the biology and materials science of the impressive number of functionally and structurally modified 2H-cyclohepta[b]furan-2-ons are going to begin. We expect that interesting reports about 2H-cyclohepta[b]furan-2-on chemistry will be increasing from now.
References
1. a) T. Nozoe, S. Seto, and S. Matsumura, Proc. Jpn. Acad., 1952, 28, 483; b) S. Seto, Sci. Rep. Tohoku Univ., Ser. 1, 1953, 37, 367; c) Chem. Abstr., 49, 8234h (1955); d) T. Nozoe and K. Kikuchi, “Dai-Yuuki Kagaku” (Comprehensive Organic Chemistry), Vol. 13, Asakura Shoten, Tokyo (1960), pp. 535-608; e) F. Pietra, Chem. Rev., 1973, 73, 293; CrossRef f) T. Asao, S. Itô, and I. Murata, Eur. J. Org. Chem., 2004, 899. CrossRef
2. T. Nozoe, K. Takase, M. Kato, and T. Nogi, Tetrahedron, 1971, 27, 6023. CrossRef
3. T. Sato, Nippon Kagakuzasshi, 1959, 80, 1342; CrossRef T. Sato, Nippon Kagakuzasshi, 1959, 80, 1345; CrossRef T. Sato, Nippon Kagakuzasshi, 1959, 80, 1347; CrossRef T. Sato, Nippon Kagakuzasshi, 1959, 80, 1349. CrossRef
4. A. Wetzel, E. G. Lewars, and K.-P. Zeller, Synthesis, 1983, 945. CrossRef
5. T. Nozoe, T. Mukai, and T. Nozoe, Bull. Chem. Soc. Jpn., 1963, 36, 38. CrossRef
6. J. Ciabattoni and H. W. Anderson, Tetrahedron Lett., 1967, 3377. CrossRef
7. T. Machiguchi and S. Yamabe, Chem. Lett., 1990, 1511. CrossRef
8. T. Asao, N. Morita, and Y. Kitahara, J. Am. Chem. Soc., 1972, 94, 3655. CrossRef
9. N. Morita, Y. Kitahara, and T. Asao, Tetrahedron Lett., 1972, 869. CrossRef
10. a) T. Asao, N. Morita, and Y. Kitahara, Chem. Lett., 1974, 747; CrossRef b) Y. Kitahara, Pure Appl. Chem., 1975, 44, 833. CrossRef
11. K. Toyota, T. Yamamoto, S. Ito, T. Asao, and N. Morita, unpublished results.
12. N. Morita. T. Asao, N. Iwagame, and Y. Kitahara, Chem. Lett., 1973, 67. CrossRef
13. T. Asao, N. Morita, C. Kabuto, and Y. Kitahara, Tetrahedron Lett., 1972, 4379. CrossRef
14. N. Morita, T. Asao, and Y. Kitahara, Tetrahedron Lett., 1974, 2083. CrossRef
15. a) E. Weiss and W. Hübel, Chem. Ber., 1962, 95, 1179; CrossRef b) R. B. King, Inorg. Chem., 1963, 2, 807; CrossRef c) D. F. Hunt, G. L. Farrant, and G. D. Rodeheaver, J. Organomet. Chem., 1972, 38, 349; CrossRef d) A. Eisenstadt, J. Organomet. Chem., 1975, 97, 443; CrossRef e) M. Franck-Neumann, F. Briton, and D. Martina, Tetrahedron Lett., 1978, 5033; CrossRef f) N. Morita and T. Asao, Chem. Lett., 1982, 1575; CrossRef g) N. Morita and T. Asao, Chem. Lett., 1985, 1879. CrossRef
16. M. Kurita, Mc. Thesis, Tohoku University, 1996.
17. N. Morita, unpublished results.
18. a) N. Morita, S. Ito, and T Asao, J. Organomet. Chem., 1993, 460, 67; CrossRef b) N. Morita, M. Kurita, K. Saito, M. Kinjo, S. Ito, T Asao, M. Ueno, T. Sato, A. Tajiri, and M. Yasunami, J. Organomet. Chem., 1998, 570, 265; CrossRef c) A. J. Birch and P. E. Cross, J. Chem. Soc. A., 1968, 332. CrossRef
19. a) N. Morita, M. Uemura, M. Kinjo, K. Saito, S. Moriyama, T. Matsuki, K. Toyota, S. Kikuchi, and S. Ito unpublished results; b) S. Moriyama, Mc. Thesis, Tohoku University, 2002.
20. N. Morita, M. Kudo, R. Yokoyama, and S. Ito, Heterocycles, 2001, 54, 679. CrossRef
21. a) M. Oda and Y. Kitahara, Angew. Chem., Int. Ed. Engl., 1969, 9. 673; CrossRef b) M. Yagihara, Ph. D. Thesis, Tohoku University, 1975.
22. a) W. S. Trahanovsky, S. L. Emeis, and A. S. Lee, J. Org. Chem., 1976, 41, 4043; CrossRef b) M. Nagel and H.-J. Hansen, Helv. Chim. Acta, 2000, 83, 1022; CrossRef c) V. Lellek and H.-J. Hansen, Helv. Chim. Acta, 2001, 84, 1712; CrossRef d) M. Nagel and H.-J. Hansen, Synlett., 2002, 692. CrossRef
23. a) Kawamoto, Y. Sugimura, and Y. Kishida, “Troponoid with Ylide”, “Topics in Nonbenzenoid Chemistry Vol. II,” P219-242, 1977, ed. by T. Nozoe, R. Breslow, K. Hafner, S. Ito, and I. Murata, Hirokawa Publishing Company Inc., Tokyo; b) Kawamoto, Y. Sugimura, and Y. Kishida, Chem. Lett., 1972, 931; CrossRef c) M. Nitta and S. Naya, J. Chem. Res. (S), 1998, 522.
24. M. J. Barrow, O. S. Mills, F. Haque, and P. L. Pauson, Chem. Commun., 1971, 1239. CrossRef
25. M. Iyoda, L. Zao, and H. Matsuyama, Tetrahedron Lett., 1995, 36, 3699. CrossRef
26. a) Y. Sasada, Bull. Chem. Soc. Jpn., 1959, 32, 165; CrossRef b) Y. Sasada, Bull. Chem. Soc. Jpn., 1959, 32, 171; CrossRef c) F. Pietra, Acc. Chem. Res., 1972, 12, 132. CrossRef
27. Y. Kurita and M. Kubo, J. Am. Chem. Soc., 1957, 79, 5460. CrossRef
28. T. Nozoe, K. Takase, T. Nakazawa, and S. Fukuda, Tetrahedron, 1971, 27, 3357. CrossRef
29. P.–W. Yang, M. Yasunami, and K. Takase, Tetrahedron Lett., 1971, 4275. CrossRef
30. a) K. Takase and M. Yasunami, J. Synth. Org, Chem. Jpn., 1981, 39, 1172; CrossRef b) Y. Kitamori, M. Yasunami, C. Kabuto, and K. Takase, Bull. Chem. Soc. Jpn., 1993, 66, 245; CrossRef c) M. Yasunami, S. Miyoshi, N. Kanegase, and K. Takase, Bull. Chem. Soc. Jpn., 1993, 66, 892; CrossRef d) M. Yasunami, T. Hioki, Y. Kitamori, I. Kikuchi, and K. Takase, Bull. Chem. Soc. Jpn., 1993, 66, 2273; CrossRef e) M. Yasunami, Y. Kitamori, I. Kikuchi, H. Ohmi, and K. Takase, Bull. Chem. Soc. Jpn., 1992, 65, 2127. CrossRef
31. S. Kuroda, M. Mouri, K. Hayashi, M. Oda, M. Yamada, I. Shimao, H. Osaki, and M. Yasunami, Chem. Lett., 1994, 85. CrossRef
32. K. Ito, H. Kawaji, and M. Nitta, Tetrahedron Lett., 1994, 35, 2561. CrossRef
33. S. Kuroda, S. Hirooka, H. Iwaki, M. Ikeda, T. Nakao, M. Ogisu, M. Yasunami, and K. Takase, Chem. Lett., 1986, 2039. CrossRef
34. a) T. Nozoe, H. Wakabayashi, K. Shindo, S. Ishikawa, C. Wu, and P. Yang, Heterocycles, 1991, 32, 213; CrossRef b) H. Wakabayashi, P.-W. Yang, C.-P. Wu, K. Shindo, S. Ishikawa, and T. Nozoe, Heterocycles, 1992, 34, 429; CrossRef c) W. Pham, R. Weissleder, and C.–H. Tung, Tetrahedron Lett., 2002, 43, 19. CrossRef
35. H. Tomioka and M. Nitta, Heterocycles, 1994, 38, 629. CrossRef
36. V. Nair, G. Anilkumar, M. V. Nandakum, B. Mathew, and N. P. Rath, Tetrahedron Lett., 1997, 38, 6441. CrossRef
37. a) M. Yasunami, Y. Kitamori, I. Kikuchi, H. Ohmi, and K. Takase, Bull. Chem. Soc. Jpn., 1992, 65, 2127; CrossRef b) M. Yasunami, T. Hioki, Y. Kitamori, I. Kikuchi, and K. Takase, Bull. Chem. Soc. Jpn., 1993, 66, 2273. CrossRef
38. H. Tomioka and M. Nitta, Heterocycles, 1994, 38, 629. CrossRef
39. G. R. Tian, S. Sugiyama, A. Mori, H. Takeshita, M. Higashi, and H. Yamaguchi, Bull. Chem. Soc. Jpn., 1989, 62, 1136. CrossRef
40. a) Y. Murase, Mc Thesis, Tohoku University, 1955; b) R. W. Alder and C. Wilshire, J. Chem. Soc., Perkin Trans. 2, 1975, 1464. CrossRef
41. a) T. Nozoe, T. Toda, T. Asao, and A. Yamanouchi, Bull. Chem. Soc. Jpn., 1968. 41, 2935; CrossRef b) M. Yokota, T. Yanagisawa, K. Kosakai, S. Wakabayashi, T. Tomiyama, and M. Yasunami, Chem. Pharm. Bull., 1994, 42, 865. CrossRef
42. a) L. R. Subramanian, M. Hanack, L. W. K. Chang, M. A. Imhoff, P. v. R. Schleyer, F. Effenberger, W. Kurtz, P. J. Stang, and T. E. Dueber, J. Org. Chem., 1976, 41, 4099; CrossRef b) P. J. Stang, M. Hanack, and L. R. Subramanian, Synthesis, 1982, 87.
43. N. Morita, T. Matshuki, M. Nakashima, T. Shoji, K. Toyota, S. Kikuchi, and S. Ito, Heterocyles, 2006, 69, 119. CrossRef
44. V. G. Nenajdenko, P. V. Vertelezkij, and E. S. Balenkova, Sulfur Lett., 1983, 24, 1011.
45. a) N. Morita, J. Higashi, K. Okada, T. Shoji, K. Toyota, M. Watanabe, M. Yasunami, S. Kikuchi, and S. Ito, Heterocycles, 2007, 72, 237; CrossRef b) J. Higashi, K. Okada, T. Shoji, K. Toyota, M. Watanabe, M. Yasunami, S. Kikuchi, S. Ito, and N. Morita, Heterocycles, 2008, 76, 759. CrossRef
46. a) R. Yokoyama, S. Ito, T. Okujima, T. Kubo, M. Yasunami, A. Tajiri, and N. Morita, Tetrahedron, 2003, 59, 8191; CrossRef b) K. Toyota, T. Yamamoto, M. Takahashi, M. Watanabe, M. Yasunami, S. Kikuchi, S. Ito, and N. Morita, unpublished results.
47. a) M. Nitta, H. Tomioka, A. Akaogi, K. Takahashi, K. Saito, and K. Ito, J. Chem. Soc., Perkin Trans. 1, 1994, 2625; CrossRef b) H. Tomioka and M. Nitta, Heterocycles, 1994, 38, 629. CrossRef
48. S. Kuroda and T. Asao, Tetrahedron Lett., 1977, 289. CrossRef
49. K. Saito, Mc Theasis, Tohoku University, 1999.
50. M. Takasugi, S. Okinaka, N. Katsui, T. Masamune, A. Shirata, and M. Ohuchi, J. Chem. Soc., Chem. Commun., 1985, 621. CrossRef
51. V. Yu. Bagirov, V. I. Scheinchenko, R. Yu. Gasanova, and M. G. Pimenov, Khim. Prir. Soedin., 1978, 811.
52. B. Alpertunga, S. Imre, H. J. Cowe, P. J. Cox, and R. H. Thomson, Tetrahedron Lett., 1983, 4461. CrossRef
53. V. Nair, G. Anilkumar, M. V. Nandakum, B. Mathew, and N. P. Rath, Tetrahedron Lett., 1997, 38, 6441. CrossRef
54. a) M. Ando,N. Kataoka, M. Yasunami, K. Takase, N. Hirata, and Y. Yanagi, J. Org. Chem., 1987, 52, 1429; CrossRef b) F. Shimoma, H. Kusaka, H. Azami, K. Wada, T. Suzuki, H. Hagiwara, and M. Ando, J. Org. Chem., 1998, 63, 3758. CrossRef
55. a) H. Wakabayashi, K. Hashiba, K. Yokoyama, K. Hashimoto, H. Kikuchi, H. Nishikawa, T. Kurihara, K. Satoh, N. Motohashi, and H. Sakagami, Anticancer Res., 2003, 23, 2447; b) K. Hashiba, K. Yokoyama, H. Wakabayashi, K. Hashimoto, K. Satoh, T. Kurihara, N. Motohashi, and H. Sakagami, Anticancer Res., 2004, 24, 3939.
56. S. Naya, T. Sakakibara, and M. Nitta, J. Chem. Soc., Perkin Trans. 2, 2001, 1032. CrossRef
57. a) S. Naya and M. Nitta, J. Chem. Soc., Perkin Trans. 1, 2000, 2777; CrossRef b) S. Naya and M. Nitta, Tetrahedron, 2003, 59, 4157. CrossRef
58. S. Naya and M. Nitta, J. Chem. Soc., Perkin Trans. 2, 2000, 2427. CrossRef
59. a) S. Naya and M. Nitta, J. Chem. Soc., Perkin Trans. 2, 2001, 275; CrossRef b) S. Naya, M. Isobe, Y. Hano, and M. Nitta, J. Chem. Soc., Perkin Trans. 2, 2001, 2253. CrossRef
60. S. Naya, T. Watano, and M. Nitta, Bull. Chem. Soc. Jpn., 2003, 76, 2035. CrossRef
61. S. Naya, K. Yoda, and M. Nitta, Terahedron, 2004, 60, 4953. CrossRef