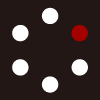
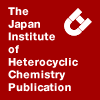
HETEROCYCLES
An International Journal for Reviews and Communications in Heterocyclic ChemistryWeb Edition ISSN: 1881-0942
Published online by The Japan Institute of Heterocyclic Chemistry
e-Journal
Full Text HTML
Received, 25th February, 2009, Accepted, 19th March, 2009, Published online, 23rd March, 2009.
DOI: 10.3987/COM-09-11689
■ Effective Procedure for the Oxidative Cleavage of Olefins by OsO4-NaIO4
Hong Woo Lee,* Sung Kwon Kang, Choong Leol Yoo, and Seung Uk Lee
New Drug Development Laboratories, Chemical Research Group, Chong Kun Dang Research Institute, Chonan P.O. Box 74, Chonan, 330-831, Korea
Abstract
Oxidative cleavage of olefins by OsO4-NaIO4 sometimes suffers from low yields due to the formation of side products. It is reported that the addition of pyrimidine can suppress the side reactions and dramatically improve the yield of this classic reaction.Phosphodiesterase (V) inhibitors (PDE5 inhibitors) are an important class of pharmaceutical compounds. The first clinical use of these compounds was in the treatment of male erectile dysfunction (MED).1
Further uses are being proposed and investigated, including pulmonary hypertension. There are currently three commercial PDE5 inhibitors which have been approved by the FDA, that is, Viagra (Pfizer), Cialis (Eli-Lilly), and Levitra (Bayer). In the course of synthesis of novel quinazoline derivatives, we needed to convert olefin 1 to aldehyde 2 via an oxidative cleavage reaction (Scheme 1). Due to the presence of the qninazoline moiety, ozonolysis did not give satisfactory results. Therefore we decide to apply the well-known OsO4-NaIO4 protocol. The oxidative cleavage of the double bond of compound 1 under the standard literature conditions of OsO4-NaIO4 gave the desired aldehyde 2 in only 58-62% moderate yield (Table entry 1).2 We found that the relatively low yield was due to the formation of 25-30% α-hydroxy ketone 3, presumably formed via the over-oxidation of the diol intermediate. Others also have reported the formation of α-hydroxy ketones as side products under the standard literature conditions.3 Although we did not find many example that actually reported α- hydroxyl ketones as the side product, we noticed that the tedious two-step procedure (dihydroxylation employing OsO4-NMO or Sharpless asymmetric dihydro-oxidation followed by oxidative cleavage with NaIO4 was employed more often than the one-step protocol (OsO4-NaIO4).4-5
Recently, Jin et al. reported an improved procedure for the oxidative cleavage of OsO4-NaIO4 using basic condition.6 Since the reaction media was acidic (pH = 3.5) condition, we thought that buffering the reaction might change the outcome of the reaction. Deionized water was replaced with the phosphate buffer (about pH = 7.0-7.2). But the reaction appeared to be very slow and no selectivity was observed (Table 1, entry 2). We next examined the effect of the addition of pulverized K2CO3 (3.5 equiv). Interestingly, TLC and HPLC7 showed very good selectivity, and only a trace amount of compound 3 wasformed (Table 1, entry 3). However, the reaction was still very slow and never went to completion even after 36 h, and gave several other unknown impurities. This experiment indicated that it was possible to inhibit the formation of compound 3 by the addition of a weak base. Nevertheless, more experiments were needed to search for the good matched base that could prevent the formation of compound 3 at a reasonable reaction rate. It is known that the rate of formation of osmium (VI) ester complex could be dramatically increased by the addition of an excess of tertiary amine such as triethylamine, Hünig base, NMO, pyridine, 2,6-lutidine, pyrazine, and pyridazine (Table 1, entry 4-10).8-9 Especially, when 2,6-lutidine was added, the reaction was indeed faster, and the selectivity was excellent (Table1, entry 8).
Although we observed only a trace amount of compound 3 by using 2,6-lutidine, we found the formation of several unknown impurities, instantaneously.
Further trial with 2,6-di-tert-butylpyridine, which is very similar to 2,6-lutidine except bulky tert-butyl part, had no effect on the reaction (Table 1, entry 11). This finding led us to investigate the use of pyrimidine as a base. To our delight, we found that 2.0 equivalent of pyrimidine effectively suppressed the formation of compound 3 and improved the yield of the desired aldehyde 2 to 85-90% with 3-5% of the side product 3 (Table 1, entry 12). Furthermore, the reaction went fast and was completed in 6-7 h without any significant impurity. 2-Chloropyrimidine, derivative of pyrimidine, gave a good result with 76-80% yield of the desired aldehyde 2. However, the reaction gave 6-9% of the side product 3 and several other unknown impurities (Table 1, entry 13). With this result in hand, we set out to explore the effect of pyrimidine on a variety of substrates in OsO4-NaIO4 reaction (Table 2).
In the absence of pyrimidine, the reaction of compound 4 and compound 6 with OsO4-NaIO4 gave only 58-66% yield of the desired product 5 and 7 (Table 2, entry 1 and 2). Although compound 4 and 6 are structurally very similar to compound 1, we have observed that the oxidative cleavage reaction of compound 4 and 6 under the classic condition (without pyrimidine as base) gave complex side products.10 Surprisingly, the yields of the desired aldehyde product 5 and 7 were improved to 88-90% with the addition of pyrimidine. It appeared that pyrimidine inhibited several uncharacterized side products. The reactions of compound 8 and 12 under the classic condition were quite slow and gave only 46-48% yields of the desired products 9 and 13 along with 20-25% recovery of the starting materials (Table 2, entry 3 and 5). It was noteworthy that prolonged reaction times gave even lower yields due to cleavage of the quinazoline moiety under basic condition. In the presence of pyrimidine base, the same reactions were completed in 8-10 h and afforded 82-72% yields of desired products 9 and 13. Substrate 12, which has an internal olefin moiety, exhibited a little bit lower yield and long reaction time compared to terminal olefin substrate 6 for both classical condition and pyrimidine base condition (Table 2, entry 2 and 5). Compound 10, branched olefin, gave about 85% yield, and this case showed similar result compared to compound 6 (Table 2, entry 2). In the case of ring open compound, substrate 14 gave only 52% yield of the aldehyde compound 15 under classical condition. Again, the yield was improved to 80% after the addition of pyrimidine base (Table 2, entry 6). In the course of the synthesis of novel fumagillol derivatives in our other project, we tried to apply this oxidative cleavage reaction to convert fumagillol compounds 16 and 18 to corresponding aldehyde fumagillol compounds 17 and 19 (Table 2, entry 7 and 8). Particularly, fumagillol substrate 16, having two epoxides and α,β-unsaturated ester at C-6 position, needed cautious approach of choosing oxidizing condition.
In this case of substrate 16 having two epoxides (spiro-epoxide and the one on C-4 olefin side chain) and the α,β-unsaturated ester at C-6 position, it exhibited only 32 % yield of the aldehyde fumagillol derivative under the classical condition. However, the yield was increased to 51% after the addition of pyrimidine base (Table 2, entry 7). Fumagillol compound 18 without C-6 side chain, that is N,N-dimethyl aminocinnamoyl moiety, showed about 38% yield of the aldehyde fumagillol 19 under no base condition. The yield also dramatically improved to 53% after the addition of pyrimidine base (Table 2, entry 8). The reaction of compound 20 under classical conditions gave only 42% yield of the desired product 21 after the reaction was stirred at rt for 10 h (Table 2, entry 9). In the presence of pyrimidine, the same reaction was completed in 7 h and afforeded 74% yield of compound 21. Both inhibiting and rate acceleration
effects by pyrimidine were quite obvious in this instance. Similar effects were also observed in
compound 22 (Table 2, entry 10). Compound 24, a terminal olefin, gave only 43% yield of the
aldehyde 25 under the classic conditions. However, the yield was improved to 81% after the addition of pyrimidine (Table 2, entry 11). Compound 26, which has a terminal olefin, gave improved result as a compound 24 (Table 2, entry 12).
In conclusion, we have successfully developed an improved procedure for the classic OsO4-NaIO4
mediated oxidative cleavage reaction. Addition of pyrimidine effectively suppressed the formation of α hydroxy ketone side product 3, accelerated the rate of the desired reaction, and dramaticallyimproved the reaction yields. In addition, these results indicated that pyrimidine base can also be used as a weak base to neutralize the acid generated in the reaction. Furthermore, we have noted that pyrimidine can suppress some other side reactions that are not characterized at the present time. We are certain that this new procedure will find wide application in organic synthesis. The mechanistic explanation of the inhibiting effect of pyrimidine is currently under investigation and will be reported in due course.
EXPERIMENTAL
Commercial available reagents used without further purification. NaBH4 (98% Aldrich Co.) was used without further purification. Anion exchange resin (Amberlite IRA-400) was used supporting the polymer of borohydride exchange resin (BER). All reactions were conducted under anhydrous condition in solvents dried over molecular sieves type 4Å under nitrogen atmosphere and performed using oven dried glassware. Melting points were determined on a Büchi 510 capillary apparatus and are uncorrected. IR spectra were recorded on a Bruker Vector 22 FT-IR spectrophotometer. NMR spectra were recorded on a Bruker DPX 400 MHz instrument operating at 400 MHz for proton and 100 MHz carbon NMR and were performed in CDCl3 solution using tetramethylsilane as the internal reference and chemical shift (δ) is reported in ppm downfield from internal tetramethylsilane. The coupling constants (J) is reported in Hz. MS spectra were recorded on a HP 5989B instrument. Flash chromatography was performed using Merck silica gel 60 (230-400 mesh) according to the published procedure.11 TLC was performed on glass backed plates pre-coated with silica (0.2 mm, 60 F254) and developed using standard visualizing agents UV fluorescence (254 and 365 nm), potassium permanganate and iodine. Fumagillol substrate 16 and 18 having cinnamoyl moiety compounds were synthesized and purified according to in house procedures.12
General Procedure: 2-(7-Hydroxy-6-nitro-4-oxo-3,4-dihydroquinazolin-8-yl)acetaldehyde (2). To a solution of compound 1 (296 mg, 0.812 mmol) in dioxane-water (10 mL, 3:1, v/v) was added pyrimidine (0.189 mL, 1.62 mmol), OsO4 (3.0 mol% in 2-methylpropanol, 180 mg, 0.016 mmol), and NaIO4 (695 mg, 3.25 mmol). The reaction was stirred at rt and monitored by HPLC and TLC. After the reaction was complete, water (10 mL) and CH2Cl2 (20 mL) were added. The organic layer was separated, and the water layer was extracted by CH2Cl2 (10 mL) three times. The combined organic layer was washed with brine and dried over Na2SO4. The solvent was removed, and the product was purified with silica gel column chromatography to afford aldehyde compound 2 (268 mg, 88%) as a white powder. mp 112-115 oC. IR (KBr) νmax cm-1: 3581, 2852, 1521, 1431. 1H NMR (400 MHz, CDCl3) δ: 3.78-3.95 (m, 2H), 7.47 (s, 1H), 8.84 (s, 1H), 9.65-9.71 (m, 1H), 9.82 (br, 1H), 12.38 (s, 1H). 13C NMR (100 MHz, CDCl3) δ: 38.32, 114.85, 117.68, 122.74, 136.15, 145.52, 149.51, 159.02, 160.77, 199.91. Anal. Calcd for C10H7N3O5 (249.18): C, 48.20; H, 2.83; N, 16.86; O, 32.10. Found: C 48.11; H 2.88; N 16.99; O 32.01.
2-(4,7-Dimethoxy-6-nitroquinazolin-8-yl)acetaldehyde (5). Yield: 0.82 g, 88% as yellowish solid. mp 113-116 oC. IR (KBr) νmax cm-1: 3101, 2869, 2837, 1508. 1H NMR (400 MHz, CDCl3) δ: 3.55-3.68 (m, 2H), 3.76 (s, 3H), 3.84 (s, 3H), 8.61 (s, 1H), 8.91 (s, 1H), 9.82 (br, 1H). 13C NMR (100 MHz, CDCl3) δ: 38.80, 55.10, 56.28, 111.55, 113.47, 117.69, 140.57, 153.20, 159.83, 160.08, 185.35, 199.91. Anal. Calcd for C12H11N3O5 (277.23): C, 51.99; H, 4.00; N, 15.06; O, 28.86. Found: C 51.85; H 4.03; N 15.16; O 28.97.
2-(4,7-Diethoxy-6-nitroquinazolin-8-yl)acetaldehyde (7). Yield: 0.86 g, 90% as yellowish solid. mp 122-125 oC. IR (KBr) νmax cm-1: 2869, 2833, 1511, 1382. 1H NMR (400 MHz, CDCl3) δ: 1.16 (t, 3H, J = 7.6 Hz), 1.22 (t, 3H, J = 7.3 Hz), 3.63-3.69 (m, 2H), 3.72-3.78 (m, 2H), 3.83-3.92 (m, 2H), 8.64 (s, 1H), 8.89 (s, 1H), 9.94 (br, 1H). 13C NMR (100 MHz, CDCl3) δ: 14.85, 14.98, 38.87, 63.90, 65.02, 111.50, 113.42, 116.28, 138.25, 154.78, 160.25, 161.38, 185.48, 198.58. Anal. Calcd for C14H15N3O5 (305.29): C, 55.08; H, 4.95; N, 13.76; O, 26.20. Found: C 55.00; H 4.92; N 14.01; O 26.06.
Dimethyl 2,2-[6-nitro-8-(2-oxoethyl)quinazoline-4,7-diyl]bis(oxy)diacetate (9). Yield: 0.87 g, 82% as yellow solid. mp 139-142 oC. IR (KBr) νmax cm-1: 2911, 2835, 1733, 1514. 1H NMR (400 MHz, CDCl3) δ: 3.51-3.60 (m, 2H), 3.66 (s, 3H), 3.74 (s, 3H), 4.68 (s, 2H), 4.71 (s, 2H), 8.63 (s, 1H), 8.97 (s, 1H), 9.92 (br, 1H). 13C NMR (100 MHz, CDCl3) δ: 38.25, 50.92, 51.78, 64.32, 65.41, 110.98, 113.24, 117.25, 140.78, 153.78, 159.02, 161.38, 169.38, 184.45, 199.07. Anal. Calcd for C16H15N3O9 (393.31): C, 48.86; H, 3.84; N, 10.68; O, 36.61. Found: C 49.06; H 3.74; N 10.67; O 36.52.
2-(4,7-Diethoxy-6-nitroquinazolin-8-yl)propanal (11). Yield: 0.83 g, 85% as yellow solid. mp 126-129 oC. IR (KBr) νmax cm-1: 2852, 2833, 1522, 1385. 1H NMR (400 MHz, CDCl3) δ: 1.16 (t, 3H, J = 7.6 Hz), 1.22 (t, 3H, J = 7.3 Hz), 1.36 (d, 3H, J = 12.3 Hz), 3.63-3.71 (m, 1H), 3.75-3.80 (m, 2H), 3.84-3.99 (m, 2H), 8.64 (s, 1H), 8.89 (s, 1H), 9.94 (br, 1H). 13C NMR (100 MHz, CDCl3) δ: 13.85, 14.57, 15.57, 45.28, 63.54, 65.17, 111.12, 112.97, 118.49, 139.27, 153.54, 159.88, 160.41, 185.20, 200.17. Anal. Calcd for C15H17N3O5 (319.31): C, 56.42; H, 5.37; N, 13.16; O, 25.05. Found: C 56.28; H 5.45; N 13.15; O 25.12.
1-(4,7-Diethoxy-6-nitroquinazolin-8-yl)propan-2-one (13). Yield: 0.67 g, 72% as yellow solid. mp 119-121 oC. IR (KBr) νmax cm-1: 2836, 1689, 1508, 1378. 1H NMR (400 MHz, CDCl3) δ: 1.18 (t, 3H, J = 7.6 Hz), 1.25 (t, 3H, J = 7.3 Hz), 2.14 (s, 3H), 3.55-3.62 (m, 2H), 3.65-3.78 (m, 2H), 3.83-3.97 (m, 2H), 8.66 (s, 1H), 8.91 (s, 1H). 13C NMR (100 MHz, CDCl3) δ: 14.85, 15.02, 30.59, 42.82, 63.08, 65.75, 111.53, 113.07, 117.49, 140.27, 153.08, 159.81, 160.55, 185.86, 204.14. Anal. Calcd for C15H17N3O5 (319.31): C, 56.42; H, 5.37; N, 13.16; O, 25.05. Found: C 56.21; H 5.52; N 13.25; O 25.02.
Methyl 4-(2-methoxy-2-oxoethoxy)-5-nitro-2-(2-oxoethylamino)benzoate (15). Yield: 0.87 g, 80% as yellow solid. mp 112-115 oC. IR (KBr) νmax cm-1: 3444, 2865, 1740, 1523. 1H NMR (400 MHz, CDCl3) δ: 3.71 (s, 3H), 3.86 (s, 3H), 4.22-4.39 (m, 2H), 4.89 (s, 2H), 6.11 (br, 1H), 6.45 (s, 1H), 8.64 (s, 1H), 9.88 (br, 1H). 13C NMR (100 MHz, CDCl3) δ: 51.17, 51.95, 64.05, 65.27, 97.23, 100.04, 123.50, 125.87, 156.19, 159.21, 165.35, 169.26, 199.80. Anal. Calcd for C13H14N2O8 (326.26): C, 47.86; H, 4.33; N, 8.59; O, 39.23. Found: C 47.88; H 4.38; N 8.62; O 39.13.
(E)-[(3R, 5S, 6R)-5-Methoxy-4-[(2R,3R)-2-methyl-3-(2-oxoethyl)oxiran-2-yl]-1-oxaspiro[2.5]octan-6-yl] 3-[4-[2-(dimethylamino)ethoxy]phenyl]acrylate (17). Yield: 0.37 g, 51% as yellow oil. mp 145-149 oC. IR (KBr) νmax cm-1: 3429, 2928, 1603, 1512. 1H NMR (CDCl3) δ: 1.06-1.18 (m, 1H), 1.24 (s, 3H), 1.81-2.20 (m, 6H), 2.37 (s, 6H), 2.56 (d, 1H, J = 4.3 Hz), 2.58 (t, 1H, J = 6.4 Hz), 2.79 (t, 2H, J = 5.6 Hz), 3.01 (d, 1H, J = 4.3 Hz), 3.45 (s, 3H), 3.71 (dd, 1H, J = 2.8 Hz), 4.12 (t, 2H, J = 5.6 Hz), 5.73-5.95 (m, 1H), 6.36 (d, 1H, J = 15.9 Hz), 6.91 (d, 2H, J = 8.7 Hz), 7.46 (d, 2H, J = 8.7 Hz), 7.62 (d, 1H, J = 15.9 Hz), 9.72 (br, 1H). 13C NMR (100 MHz, CDCl3) δ: 18.80, 21.08, 29.47, 42.08, 43.51, 45.18, 45.73, 52.07, 54.01, 54.58, 57.55, 58.49, 59.66, 66.22, 77.16, 77.68, 114.02, 114.53, 116.69, 126.80, 127.51, 128.67, 145.50, 156.79, 166.50, 202.11. Anal. Calcd for C26H35NO7 (473.56): C, 65.94; H, 7.45; N, 2.96; O, 23.65. Found: C 65.55; H 7.62; N 3.04; O 23.79.
2-[(2R,3R)-3-[(3R,5S,6R)-6-Hydroxy-5-methoxy-1-oxaspiro[2.5]octan-4-yl]-3-methyloxiran-2-yl]acetaldehyde (19). Yield: 0.42 g, 53% as yellow oil. mp 119-121 oC. IR (KBr) νmax cm-1: 3424, 2924, 1632, 1170. 1H NMR (CDCl3) δ: 1.03-1.12 (m, 1H), 1.14 (s, 3H), 1.78-2.27 (m, 7H), 2.30-2.37 (m, 1H), 2.40-2.48 (m, 1H), 2.52 (d, 1H, J = 4.4 Hz), 2.70 (dd, 1H, J = 7.1 and 5.9 Hz), 2.86 (d, 1H, J = 4.4 Hz), 4.21-4.38 (m, 1H), 9.66 (s, 1H). 13C NMR (100 MHz, CDCl3) δ:18.84, 24.32, 29.28, 41.85, 43.51, 52.44, 54.26, 55.09, 57.78, 58.81, 72.91, 80.64, 202.13. Anal. Calcd for C13H20O5 (256.29): C, 60.92; H, 7.87; O, 31.21. Found: C 61.25; H 7.81; O 30.94.
1-Benzylpiperidine-4-carbaldehyde (21). Yield: 0.66 g, 74% as yellow oil. mp 145-149 oC. IR (KBr) νmax cm-1: 3046, 2865, 1725,. 1H NMR (400 MHz, CDCl3) δ: 1.55-1.65 (m, 2H), 1.88-1.95 (m, 2H), 2.22-2.33 (m, 4H), 2.38 (m, 1H), 3.66 (s, 2H), 7.10 (m, 2H), 7.22-7.38 (m, 3H), 9.68 (m, 1H). 13C NMR (100 MHz, CDCl3) δ: 26.43, 26.49, 50.06, 51.59, 51.77, 60.05, 126.51, 128.16, 128.98, 129.28, 130.22, 136.27, 202.83. Anal. Calcd for C13H17NO (203.13): C, 76.81; H, 8.43; N, 6.89; O, 7.87. Found: C, 76.97; H, 8.60; N, 6.85; O, 7.58.
2-[3-(1-Benzylpiperidin-4-yl)-2-oxopropyl]-4,5-dimethoxybenzaldehyde (23). Yield: 0.72 g, 41% as yellow solid. mp 188-191 oC. IR (KBr) νmax cm-1: 3381, 3037, 2870, 1240. 1H NMR (400 MHz, CDCl3) δ: 1.42-1.50 (m, 4H), 1.68-1.72 (m, 1H), 2.22-2.29 (m, 4H), 2.41 (d, 2H, J = 5.1 Hz), 3.62 (s, 2H), 3.74 (s, 2H), 3.77 (s, 3H), 3.84 (s, 3H), 7.01 (s, 1H), 7.05-7.12 (m, 3H), 7.24-7.32 (m, 3H), 10.12 (s, 1H). 13C NMR (100 MHz, CDCl3) δ: 29.61, 29.99, 31.84, 47.64, 49.75, 51.12, 51.48, 56.15, 56.28, 60.08, 114.02, 115.42, 127.01, 128.11, 128.45, 128.49, 128.87, 129.08, 129.63, 125.51, 148.07, 156.25, 192.47, 206.67. Anal. Calcd for C24H29NO4 (395.21): C, 72.89; H, 7.39; N, 3.54; O, 16.18. Found: C, 72.85; H, 7.49; N, 3.64; O, 16.02.
2-[(4S,6S)-2,2-Dimethyl-6-(2-oxoethyl)-1,3-dioxan-4-yl]acetonitrile (25). Yield: 0.65 g, 81% as yellow oil. mp 148-153 oC. IR (KBr) νmax cm-1: 3042, 1682, 1328. 1H NMR (400 MHz, CDCl3) δ: 1.19 (s, 3H), 1.23 (s, 3H), 1.44-1.50 (m, 1H), 1.78-1.84 (m, 1H), 2.41-2.52 (m, 2H), 2.62-2.69 (m, 2H), 3.80-3.88 (m, 1H), 4.02-4.10 (m, 1H), 9.71 (s, 1H). 13C NMR (100 MHz, CDCl3) δ: 23.04, 26.77, 26.98, 37.66, 50.20, 61.32, 65.77, 95.28, 117.45, 201.23. Anal. Calcd for C10H15NO3 (197.11): C, 60.90; H, 7.67; N, 7.10; O, 24.34. Found: C, 60.80; H, 7.62; N, 7.18; O, 24.41.
(R)-3-(tert-Butyldimethylsilyloxy)-3-((R)-1,4-dioxaspiro[4.5]decan-2-yl)propanal (27). Yield: 0.77 g, 73% as yellow oil. IR (KBr) νmax cm-1: 3402, 3003, 1654, 1388. 1H NMR (400 MHz, CDCl3) δ: 0.06 (s, 6H), 0.90 (s, 9H), 1.42-1.60 (m, 10H), 2.17-2.22 (m, 2H), 3.31-3.42 (m, 1H), 3.62-3.72 (m, 1H), 3.90-4.08 (m, 2H), 9.76 (s, 1H). 13C NMR (100 MHz, CDCl3) δ: 4.52, 5.24, 16.11, 16.25, 18.44, 25.78, 25.99, 26.45, 28.41, 35.47, 36.29, 47.10, 62.25, 65.52, 83.57, 111.36, 201.02. Anal. Calcd for C17H32O4Si (328.21): C, 62.15; H, 9.82; O, 19.48; Si, 8.55. Found: C, 61.87; H, 9.91; O, 19.99.
ACKNOWLEDGEMENTS
The authors would like to thank for the Research Grant (A08-1022-B91801-08N1-00010A) from Ministry of Health and Welfare, Korea for financial support.
References
1. M. Boolell, M. J. Allen, S. A. Ballard, S. Gepi-Attee, G. J. Muirhead, G. J. A. M. Naylor, I. H. Osterloh, and J. C. Gingell, Clin. J. Impot. Res., 1996, 8, 47; H. Wilkens, A. Guth, J. Koning, N. Forestier, B. Gremers, B. Hennen, M. Bohm, and G. W. Sybrecht, Circulation, 2001, 104, 1218. CrossRef
2. R. Paper, D. S. Alen, R. U. Jr. Lemieux, and W. S. Johnson, J. Org. Chem., 1956, 21, 487. CrossRef
3. P. K. Grant, L. R. Hanton, G. P. Lynch, W. T. Robinson, and G. Wong, Aust. J. Chem., 1994, 47, 71; B. B. Lohray, V. Bhushan, and R. K. Kumar, J. Org. Chem., 1994, 59, 1357. CrossRef
4. There are numerous examples of using the two-step procedure to cleave olefins. The following are some of the recent applications: C. Francavilla, W. Chen, and F. R. Jr. Kinder, Org. Lett., 2003, 5, 1233; CrossRef Z. Wang and M. G. Moloney, Tetrahedron Lett., 2002, 43, 9629; CrossRef R. E. Taylor, Y. Chen, and A. Beatty, J. Am. Chem. Soc., 2003, 125, 26; CrossRef W. R. Roush, T. D. Bannister, M. D. Wendt, J. A. Jablonowski, and K. A. Scheidt, J. Org. Chem., 2002, 67, 4275. CrossRef
5. W. Yu, Y. Zhang, and Z. Jin, Org. Lett., 2001, 3, 1447. CrossRef
6. W. Yu, Y. Mei, Y. Kang, Z. Hua, and Z. Jin, Org. Lett., 2004, 6, 3217. CrossRef
7. Analytical reversed-phase HPLC was performed on a Hewlett-Packard HPLC series 1100 system with 254 nm UV detection, using Kromasil C18 (UG 100 Å, 5 ㎛, 4.6 mmØ × 15 cm). A solvent system consisting of 0.05 M Ammonium formate (at adjusting pH 4.0) in 50% water - 30% in MeOH and 20% acetonitrile was used. The samples volumes of 10.0 µL were injected. The column oven was controlled at 35℃. The flow rate was 1.0 mL/min.
8. R. Criegee, B. Marchaud, and H. Wannowlus, Justus Liebigs Ann. Chem., 1942, 550, 99 and their references therein. CrossRef
9. M. Schroder, Chem. Rev., 1980, 80, 187 and references therein. CrossRef
10. We did not try to characterize each side products.
11. W. C. Still, M. Khan, and A. Mitra, J. Org. Chem., 1978, 43, 2923. CrossRef
12. N,N-Dimethyl cinnamoylfumagillol substrate 16 was prepared as follow: To a stirred suspension of a N,N-dimethylaminocinnamic acid (386 mg, 1.64 mmol) in toluene was added SOCl2 (390 mg, 3.28 mmol) and the mixture was refluxed for 2 h. Toluene was removed under vacuum evaporation, and the residue was used without further purification for the next step. To the residue in DMF (10 mL) was added NaH (236 mg, 3.94 mmol) at ice-water temperature. Fumagillol (185 mg, 0.66 mmol) was added to the mixture, maintaining at that temperature. After addition, the reaction mixture was allowed to warm to ambient temperature and stirred 2.5 h. The mixture was quenched with saturated NH4Cl (20 mL) and washed with EtOAc (25 mL). The organic layer was washed with saturated NH4Cl (25 mL) five times, and brine (30 mL) in sequence. The separated organic layer was dried over MgSO4 and concentrated in reduced pressure, giving a residue, which was subjected to column chromatography (SiO2, EtOAc-n-hexane, 1:1, v/v) to afforded the desired product (256 mg, 78%) product was used without further purification in the next step. 1H NMR (CDCl3) δ: 1.06-1.18 (m, 1H), 1.24 (s, 3H), 1.66 (s, 3H), 1.75 (s, 3H), 1.81-2.06 (m, 6H), 2.37 (s, 6H), 2.56 (d, 1H, J = 4.3 Hz), 2.58 (t, 1H, J = 6.4 Hz), 2.79 (t, 2H, J = 5.6 Hz), 3.01 (d, 1H, J = 4.3 Hz), 3.45 (s, 3H), 3.71 (dd, 1H, J = 2.8 Hz), 4.12 (t, 2H, J = 5.6 Hz), 5.22 (br, 1H), 5.73-5.79 (m, 1H), 6.36 (d, 1H, J = 15.9 Hz), 6.91 (d, 2H, J = 8.7 Hz), 7.46 (d, 2H, J = 8.7 Hz), 7.62 (d, 1H, J = 15.9 Hz). Fumagillol substrate 18 was prepared as follow: To fumagillin (120 mg, 0.26 mmol), 0.1 N NaOH (12 mL, 1.2 mmol) was added and the reaction mixture was stirred at room temperature for 6.5 h. the reaction mixture was diluted with EtOAc (50 mL) and brine (30 mL), and dried over MgSO4. After filtered, the solvent was removed by evaporating under reduced pressure. The residue was purified by silica gel column chromatography (eluent: EtOAc-n-hexane, 1:1, v/v) to obtained 51 mg (52%) as colorless oil. 1H NMR (CDCl3) δ: 1.03-1.12 (m, 1H), 1.14 (s, 3H), 1.65 (s, 3H), 1.74 (s, 3H), 1.78-2.27 (m, 7H), 2.33-2.42 (m, 1H), 2.39-2.49 (m, 1H), 2.52 (d, 1H, J = 4.4 Hz), 2.70 (dd, 1H, J = 7.1 and 5.9 Hz), 2.86 (d, 1H, J = 4.4 Hz), 4.27-4.38 (m, 1H), 5.15-5.19 (m, 1H).