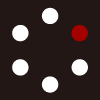
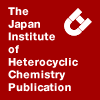
HETEROCYCLES
An International Journal for Reviews and Communications in Heterocyclic ChemistryWeb Edition ISSN: 1881-0942
Published online by The Japan Institute of Heterocyclic Chemistry
e-Journal
Full Text HTML
Received, 25th February, 2009, Accepted, 23rd April, 2009, Published online, 23rd April, 2009.
DOI: 10.3987/COM-09-S(S)14
■ Characterization of Various O-Bisguanidinobenzene-Benzoic Acid Complexes in Solid and in Solution States
Takuya Kumamoto, Kazuaki Shikii, Masatoshi Kawahata, Kentaro Yamaguchi, Waka Nakanishi, Tsutomu Ishikawa, and Hiroko Seki*
Chiba University, 1-33 Yayoi-cho, Inage-ku, Chiba 263-8522, Japan
Abstract
Stoichiometry-controlled complexation of o-phenylenebis(N,N’-dimethyl-N,N’-ethylene)guanidine (BG) and benzoic acid (BA), which is forming 1 : n (n = 1 – 4) BG + BA systems, was observed in the solid state by X-ray structure analysis and in solution by using cold-spray ionization mass spectrometry (CSI-MS) and pulsed field gradient (PFG)-NMR diffusion. The relation of observed diffusion coefficient in solution phase and the volume of the corresponding complexes estimated by calculation of the solid state structure showed good linearity, which implies that the volume of the complexes would increase depend on the ratio of BA to BG.INTRODUCTION
The creation of desired molecular complex by controlling the cluster formation attracted considerable interest in the field of supramolecular chemistry.1 The cluster formation is one of the most important steps to generate supramolecular systems, however, detailed observation of this phenomenon in solution is generally difficult. NMR is one of the most effective methods to elucidate the dynamic nature of organic molecules in solution.2 Other instrumentational analysis including MS helps to reveal the role of hydrogen bond and other kinds of weak bond formation in solution.3 Recently, we reported the structure analysis, in solution, of steroid compounds by means of cold-spray ionization (CSI)-MS3c and pulse field-gradient (PFG) NMR.4 Narcissistic aggregation (formation of large-scale aggregated chain structure or cluster) based on the intermolecular hydrogen bonding at the hydroxyl and carboxyl functionalities in the steroid compounds observed in crystal structures was confirmed with further CSI-MS analysis and accurate NMR diffusion experiments.5
We have alternatively reported that o-phenylenebis(N,N’-dimethyl-N,N’-ethylene)guanidine (BG) acts as a powerful hydrogen acceptor in hydrogen bonded complexation with hydrogen donor aromatics such as benzoic acid (BA), phenol, and benzyl alcohol and formed the expected stoichiometry-controlled crystalline complexes with BA in the molecular ratio of 1 : 1 to 1 : 4.6 As a next stage, we have approached to the structural analysis of BG itself and BG + n BA complexes to confirm the formation of such a stoichiometry-controlled complexation in solution. In this article, we described the detail of our trials for the structural analysis of BG and the BG + n BA complexes constructed through weak bonds such as hydrogen bonds and/or CH-π interactions in solid by X-ray crystallography as well as in solution by CSI-MS and PFG-NMR6b including rationalization of the diffusion coefficients and the size of complexes estimated by computational analysis of the crystal structures.
RESULTS AND DISCUSSION
In Figure 2, the crystal structures of BG itself7 and the BG + n BA (n = 1 - 4)6 are presented. No representative difference was observed on the structure between BG itself (Figure 2a) and BG part of complexes except BG + 4 BA (Figure 2b-d). Only one guanidinyl group is involved hydrogen bonding with BA (red and orange dotted line, respectively) in BG + n BA (n = 1 and 2) (Figure 2b and c). A second BA (pink) interacts with the first BA (orange) through hydrogen bond (pink dotted line) in 1 : 2 complex (Figure 2c). Interestingly, 1 : 3 complex of BG and BA (Figure 2d) exhibits a combined structure of 1 : 1 and 1 : 2 complexes. The left part (blue BG and red BA) corresponds to the 1 : 1 system whereas the right part comes from the 1 : 2 system (light blue BG and two BAs). Three strong hydrogen bonds are observed in this system: C-O···H-N (O···N distance 2.54 Å red dotted line in the left part), C-O···H-N (O···N distance 2.73 Å orange dotted line) and C-O-H···O-C (O···O distance 2.50 Å pink dotted line in the right). The crystal structure of BG + 4 BA, which possesses C2 symmetry, is presented in Figure 2e. Two strong hydrogen bonds (red dotted line) in addition to T-shaped C-H-interactions (orange dotted line) are clearly observed between BG and BA.
The structures of the BG + n BA (n = 1 - 4) system in solution are studied by means of MS.8 Conventional electrospray ionization (ESI) MS shows no ion peak corresponding to the complexes observed in the solid state. The ion peak of [BA-H]- in the negative mode and [BG+H]+ in the positive mode were detected. On the other hand, the molecular ratio-dependent complexes are clearly observed by negative mode CSI-MS measurement (Figure 3). In all the system, the negative ion [BA-H]- is attached to the neutral BG + n BA complexes, giving rise to the ([(BG + n BA) + (BA-H)]- signals. The molecular ion peak of 1 : n systems [(BG + n BA) + (BA-H)]- (n = 1; m/z 543; n = 2; m/z 665, n = 3; m/z 787, n = 4; m/z 909) are unambiguously observed. In the cases of BG + n BA (n = 2 – 4), other ion peak(s) corresponding to lower stoichiometric systems [(BG + m BA) + (BA-H)]- (1 < m < n) are also observed.
1H-NMR chart of BG itself, BG in the presence of various equivalent of BA (BG : BA = 1 : 0 to 1 : 4), and BA itself in CD2Cl2 were shown in Figure 4.8 Two kinds of protons on benzene ring of BG were observed overlapped for each other in BG itself and BG + 4 BA complex, but separated signals were observed in BG + n BA (n = 1 – 3). Signal at higher field shift (6.96 ppm) on aromatic region of BG was assigned as protons at the ortho position of guanidine nitrogen based on 1H-15N HMBC correlation of
BG + 2 BA.9 Continuous shift of the signals were observed depend on the equivalents of BA in BG + n BA complexes. On the aromatic region of BG, lower-field shift of the resonance of aromatic protons was found with increasing of the equivalent of BA up to BG : BA = 1 : 2, and only slight shifts were observed up to BG : BA = 1 : 4. These lower-field shifts can be explained by the protonation on guanidine imino nitrogens. On the other hand, the signals of ethylene moiety on BG showed upfield shift dependent on the equivalent of BA. The reason is not clear, however, the BA would locate beside of BG through hydrogen bonds, of which ethylene parts would be more shielded by the anisotropic effect of benzene ring of BA. No obvious shift was observed for methyl groups of BG, which would be the result of the sum of the two opposite factors (vide infra). The higher field shift of aromatic protons of BA would be due to the generation of negative charge on acid part of BA.
We also performed diffusion study of BA and BG themselves and the BG + n BA (n = 1 - 4) complexes by PFG-NMR for these samples. The pure BA and BG solutions exhibited large diffusion coefficients (entries 1, 3 in Table 1). For BG + n BA complexes, the observed diffusion coefficients decreased in accordance with the increasing the number of attached BA (n) (entries 3-7). Only slight decrease of the coefficient was observed on high concentration (5 mM) of BA itself (entry 2), which is negligible compared with that observed in BG + 4 BA complex (entry 7). These results clearly support the ordered formation of the complexes between BG and BA in solution phase as observed by CSI-MS.
For further support of the structural analysis in solution, relation of the volume of BG and BG + n BA complexes and the observed diffusion coefficients was examined. The volume of BA, BG, and the complexes was estimated of crystal structures in Figure 2 by semiempirical calculation programs ToMoCo and Winmostar10 and was found to be increased with the number of BA in the complex as expected (Table 1). Based on the Stokes-Einstein equation (D = kT/cπηr), diffusion coefficient is estimated from volume of complexes as followed:
The relationship between the diffusion coefficient D obtained in this experiment and the calculated ∛V was shown to be inversely proportional with good linearity (R2 = 0.92, Figure 5). Even though the structure in solid state is not always the same as that in solution state, these results imply that the volume of the BG + n BA complexes in solution would increase depend on the ratio of BA to BG as to the complexes in solid state, and the measurement of diffusion coefficient would be valuable for the evaluation of the size of the cluster consisted with not only single5 but also multiple species.6
To identify the importance of acid functions in BA for the complex formation in solution phase, acetophenone (AP), possessing the similar molecular weight to BA without acidic hydrogen, was used instead of BA. Almost the same diffusion coefficient for BG (12.9 x 10-10 m2/s, 21.6 x 10-10 m2/s for AP) in BG + 4 AP mixture was observed as those of BG itself (13.0 x 10-10 m2/s), which implies that AP will not constitute a complex with BG in solution phase. The same diffusion data was reprocessed for DOSY11 and clearly showed single diffusion coefficients for the BG + n BA complexes but each one for BG and AP in BG + 4 AP mixture (see Supporting information).
In consequence, stoichiometry-controlled absolute ordered cluster formation of BG and BA was confirmed by X-ray crystallography and the formation of complexes with the same ratio of BG and BA was also confirmed by CSI-MS. These results give experimental evidence that desired molecular complex in solution as well as in the solid state by simple mixing acid and base components can be obtained. The diffusion coefficient of the BG – BA complexes estimated from PFG-NMR was linearly related with the calculated volume of the complexes based on the crystal structures, which implies that the volume of the BG + n BA complexes in solution would increase depend on the ratio of BA to BG as to the complexes in solid state. Further investigation on the formation of complexes with BG and the other acid and metal ions12 is now in progress.
SUPPORTING INFORMATION
DOSY charts of BG + n BA (n = 1 – 4) and BG + 4 AP (6 pages).
EXPERIMENTAL
Apparatus
CSI-MS spectrum measurement was performed with a TOF mass spectrometer (JMS-T100LC, JEOL) equipped with the CSI source. Needle voltage, -3500 kV; ring lens voltage, -20 V; orifice1 voltage, -25 V; orifice2 voltage, -5 V; resolution (10% valley definition), 6000; sample flow, 33 µL/min; solvent, dry CH2Cl2; concentration, 1.0 mmol/L; spray temperature, -25 °C; ion source temperature, 10 °C. The 1H NMR experiments were performed using 400 MHz (JEOL ECX400) and 700 MHz NMR spectrometer (JEOL ECA700). The diffusion experiments were carried out with 700 MHz NMR on 1 h after the preparation of the sample solution at 283 K (± 0.1 K) in 3 mm NMR tubes without spinning in order to decrease the influence of thermal convection. Typical measurement conditions are as follows: pulse sequence, bipolar pulse pair stimulated echo (BPP-STE); gradient max, 26 G/cm; gradient strength, 2.0 – 2.4 ms; diffusion time, 100 ms; scans, 32. The sample concentration was 1.0 mM. The gradient strength was varied in 15 steps to obtain an approximately 90% decrease in the resonance intensity at the maximum gradient strength. The diffusion coefficients were determined by curve fitting of the peak intensity of BG’s methyl group. The DOSY data were processed using an exponential apodization function corresponding to a 1.0 Hz line broadening and Fourier transformed with the SPLMOD algorithm.11
Synthesis of BG + 3 BA complex
A solution of BA (122 mg, 0.10 mmol) in CH2Cl2 (1.0 mL) was slowly added to a solution of BG (100 mg, 0.33 mmol) in CH2Cl2 (2.0 mL) and the whole was stirred at room temperature (rt) for 3 h. Evaporation of the solvent gave a yellow viscous oil, which was crystallized by trituration with AcOEt. Recrystallization from AcOEt gave the 1 + 3 complex (203 mg, 91%) as colorless needles.
mp 112-113 °C. IR (KBr, cm-1) 3680, 1600. 1H-NMR (400 MHz, CDCl3) δ (ppm) : 2.74 (s, 12 H), 3.32 (s, 8H), 6.99-7.19 (m, 4H), 7.34-7.38 (m, 6H), 7.43-7.46 (m, 3H), 8.07-8.09 (m, 6H). 13C-NMR (100 MHz, CDCl3) δ (ppm) 34.6, 48.7, 125.4, 125.8, 128.0, 129.8, 131.4, 134.5, 135.6, 156.1, 170.9. Anal. Calcd for C23H30N6O2: C, 66.65; H, 6.35; N, 12.60. Found: C, 66.65; H, 6.34; N, 12.57.
ACKNOWLEDGMENTS
Dr. Jun Uzawa and Mr. Mamoru Imanari, Chemical Analytical Center, Chiba University, were acknowledged for helpful discussion. This work was supported by Grant-in-Aid for Scientific Research (C) (19590002).
References
1. (a) G. R. Desiraju, J. Mol. Struc., 2003, 656, 5; CrossRef (b) A. D. Bond and W. Jones, ‘Supramolecular Organization and Materials Design,’ ed. by W. Jones and C. N. R. Rao; Cambridge University Press: Cambridge, 2002; pp. 391-443; (c) W. Jones, ‘Organic Molecular Solids: Properities and Applications,’ CRC Press: New York, 1997; pp. 181-194; (d) G. R. Desiraju, Angew. Chem. Int. Ed., 1995, 34, 2311. CrossRef
2. (a) H. Seki, M. Nakagawa, A. Hashimoto, and T. Hino, Chem. Pharm. Bull., 1993, 41, 1173; (b) C. Dell'Erba, F. Gasparrini, S. Grilli, L. Lunazzi, A. Mazzanti, M. Novi, M. Pierini, C. Tavani, and C. Villani, J. Org. Chem., 2002, 67, 1663. CrossRef
3. (a) J. B. Fenn, M. Mann, K. Meng, S. F. Wong, and C. M. Whitehouse, Science, 1989, 264, 64; CrossRef (b) Z. Takats, S. C. Nanita, R. G. Cooks, G. Schlosser, and K. Vekey, Anal. Chem., 2003, 75, 1514; CrossRef (c) K. Yamaguchi, J. Mass Spectrom., 2003, 38, 473. CrossRef
4. P. Stilbs, Progr. Nucl. Magn. Reson. Spectrosc., 1987, 19, 1. CrossRef
5. K. Shikii, H. Seki, S. Sakamoto, Y. Sei, H. Utsumi, and K. Yamaguchi, Chem. Pharm. Bull., 2005, 53, 792; CrossRef K. Shikii, S. Sakamoto, H. Seki, H. Utsumi, and K. Yamaguchi, Tetrahedron, 2004, 60, 3487. CrossRef
6. (a) M. Kawahata, K. Yamaguchi, and T. Ishikawa, Cryst. Growth Des., 2005, 5, 373; CrossRef (b) For preliminary report: M. Kawahata, K. Shikii, H. Seki, T. Ishikawa, and K. Yamaguchi, Chem. Pharm. Bull., 2006, 54, 147. CrossRef
7. W. Nakanishi, A. Ogino, M. Kawahata, K. Yamaguchi, and T. Ishikawa, Tetrahedron Lett., 2007, 48, 8526. CrossRef
8. The solutions for MS and NMR measurement were prepared by dissolving the crystallized BG + n BA complexes.
9. Correlation on 1H-15N HMBC was observed between the signal at 6.96 ppm on 1H NMR and ca. 95 ppm on 15N NMR but not with the signal at 7.05 ppm. For the chemical shifts of protonated guanidine on 15N NMR (78.0 ppm in DMSO), see: G. A. Olah, A. Burrichter, G. Rasul, M. Hachoumy, and G. K. Surya Prakash, J. Am. Chem. Soc., 1997, 119, 12929. CrossRef
10. ToMoCo: M. Arakawa and K. Funatsu, J. Comput. Chem. Jpn., 2006, 5, 119; Winmostar: N. Senda, http://winmostar.com/index_en.html. Both calculation methods gave similar results.
11. R. Huo, R. Wehrens, J. van Duynhoven, and L. M. C. Buydens, Anal. Chim. Acta, 2003, 490, 231. CrossRef
12. Recently, wea and Himmel et al.b reported the formation and the structural analysis of BG – metal complexes. (a) K. Suda, N. Saito, T. Kumamoto, W. Nakanishi, M. Kawahata, K. Yamaguchi, Y. Ogura, K. T. Suzuki, and T. Ishikawa, Heterocycles, 2009, 77, 375; CrossRef (b) A. Peter, U. Wild, O. Hübner, E. Kaifer, and H.-J. Himmel, Chem. Eur. J., 2008, 14, 7813. CrossRef