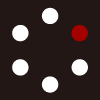
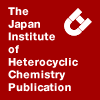
HETEROCYCLES
An International Journal for Reviews and Communications in Heterocyclic ChemistryWeb Edition ISSN: 1881-0942
Published online by The Japan Institute of Heterocyclic Chemistry
e-Journal
Full Text HTML
Received, 26th February, 2009, Accepted, 14th May, 2009, Published online, 14th May, 2009.
DOI: 10.3987/COM-09-11690
■ An Enantioselective Approach to Synthesis of Advanced Chiral Templates for the Construction of Indolic and Indolizidinic Alkaloids
Radouane Affani, Sébastien Comesse,* Adam Daïch,* Louis Hamon, and Catherine Kadouri-Puchot*
URCOM, EA 3221, CNRS-INC3M FR 3038, UFR of Sciences & Technology, Laboratory of Chemistry, University of Le Havre, 25 Rue Philippe Lebon, BP 540, F-76058 Le Havre Cedex, France
Abstract
A new approach for the syntheses of chiral indolic and indolizidinic templates is presented. They were obtained in three steps starting from a chiral primary amino-vinylsilane. The key step was the N-acyliminium π-cyclization, leading diastereoselectively to the formation of the six membered ring templates.Indolizidines or their unsaturated analogues are among of the most important structural motifs found abundantly in nature. Their sources are extremely divers varying from marine and terrestrial materials to organisms, such as plants, bacteria, fungi, vertebrates and invertebrates.1 For example, alkaloids 1,2 (Scheme 1) are well-known for their ability to function as excellent inhibitors of biologically important pathways. These include the binding and processing of glycoproteins, potent glycosidase inhibitory activities,2 activity against AIDS virus HIV and some carcinogenic cells as well as against other important pathologies.3 In addition, gephyrotoxins such as 3a-d show significant biological activities as exemplified by the ability of many of them to act as non-competitive blockers of neuromuscular transmission by interacting with nAChRS (nicotinic acetylcholine receptors) of the CNS.4
Although a vast amount of work towards these bicyclic alkaloids in both chiral and racemic syntheses has been appeared in the literature, the challenges of a plethora of the innovative strategies developed and used for this purpose rest the introduction of chirality and several functional groups to the indolizidine scaffold. The latter fact is often problematic and consequently leads to the use of multistep sequences.
In light of the limitations encountered during these investigations and taking into account the interesting biological profiles and potential of these structures as valuable candidates for new therapeutic agents, the demand for direct and straightforward methods has become increasingly clear. In this context, the design of simple aza-bicyclic templates capable of driving by simple additional manipulations to structural diversity and complexity including subgroups of these alkaloids is of high interest in organic synthesis.5
Our objective herein was directed towards the enantioselective synthesis of the aza-bicyclic systems 4A,B (Scheme 2). The latter, equipped with appropriates functionalities, could tolerate numerous and known synthetic manipulations6 to provide a large number of polyhydroxylated indoizidine alkaloids, gephyrotoxins and corresponding benzoindolizidines with different degrees of unsaturations.
Accordingly, the N-acyliminium ions chemistry using enantiopure amino-vinylsilane7 and imide as starting materials would appear to provide an ideal solution of this dilemma. Retrosynthetically, we envisioned that we could establish the R/S stereochemistry at C8a-position selectively through π-cationic cyclization of precursor 5, which can be prepared easily in a two step-sequence from amine 6 (Scheme 2).
We focused in the first place our study on the synthesis of the phtalimide derivative 4A (Schemes 2, 3). Indeed, we anticipated that our approach would be less problematic using an aromatic ring in place of the double bond on the five membered ring system (phtalimide compared to maleimide). The primary amine 6 was converted in two steps, condensation with phtalic anhydride followed by the reduction of the resulting imide with LiEt3BH, to the hydroxylactam 5Aa in 74% overall yield. This one was isolated in a one to one ratio of the two diastereoisomers, implying no stereocontrol from the stereocenter presents on the side chain. The cyclization was first performed in the presence of two equivalents of BF3.OEt2 as Lewis acid and led to the tricyclic system 4A in 70% yield. Recently, an efficient method using bismuth (III) triflate as a Lewis acid catalyst was reported by some of us to generate the formation of N-acyliminium species.8 In order to use easier and cleaner conditions, we applied this new experimental procedure to the acetoxylactam 5Ab obtained from the corresponding imide by a simple one-pot reduction/acetylation methodology in 67% yield.9 As a matter of fact, when we performed this catalytic acid-mediated π-cationic cyclization with Bi(OTf)3 (1 mol%), we were pleased to isolate compound 4A in similar yield than with BF3.OEt2 (69% instead of 70%).10 This result shows that the use of 1 mol% of Bi(OTf)3 is as efficient as the use of a stoechiometric amount of BF3.OEt2 (200 mol%). In addition the catalytic sequence can be applied with success to cyclizations involving vinylsilane moiety providing 6-membered ring possessing an unsaturation. In both cases, only one diastereoisomer was formed during the cyclization process thanks to the phenyl substituent presents on the side chain.11 A syn relationship between the angular H of the newly formed stereogenic center and the phenyl substituent was firstly deduced from previously published works.12,13
With the aim to confirm the stereochemistry of the newly created stereocenter and to study the possibility of epimerization of this one in order to reach the trans relationship presents in gephyrotoxins (Scheme 1, 3a,c,d) we investigated the migration of the double bond and its reduction. Treated in basic conditions (MeONa in MeOH), compound 4A gave the conjugate more stable isomer 7 in 84% yield. The hydrogenation process was applied on both 4A and 7 and provided the reduced products 8 and 9 in 63% and 69%, respectively, as two diastereoisomers, epimers at the carbon of the ring junction (Scheme 3). The total diastereoselectivity observed during the reduction of 7 can be explained by the steric hindrance of the phenyl substituent, involving the approach by the hydrogen on the more reachable face and implying the formation of the trans diastereomer. Thus, we obtained the same relative stereochemistry than the one in gephyrotoxins and that result confirmed, by comparison, the cis relationship in product 8.
Encouraged by these results, we turned our attention to the maleimide derivative in view to synthesis our main target, the bicyclic system 4B (Scheme 4). So, condensation of amine 6 onto maleic anhydride led to the imide 5Ba in 88% yield. The selective 1,2 reduction of the C=O bond versus the 1,4 was found to be troublesome, only the treatment by the couple NaBH4/CeCl3 in methanol produced the desired hydroxylactam 5Bb in 86% yield. When reacted with two equivalents of BF3.OEt2, 5Bb led to the formation of the expected bicyclic compound 4B but with a low yield of 25% partially explained by the presence of the allylsilane derivative 10 in 8% yield and other non-characterized side products. The diene 4B was obtained as a single diastereoisomer11 and despite the low yield this result legitimates our strategy. For its part, the compound 10 was a result and an evidence of the aza-Cope transposition between the N-acyliminium ions I and J, this last one giving after hydrolysis the debenzylated molecule 10.
A dramatic change in the course of the π-cyclizations between 5Aa,b and 5Bb was observed, the first giving good yields and the second providing poor yields and side reactions. This significant difference is the direct consequence of the presence or not of an aromatic ring on the pyrrolidine one. In order to get an idea about these results, we calculated14 the transition state energies between the six possible cationic species I-N in accordance with the results published earlier on iminium series (Scheme 5).17 The cation products N or J would be obtained from L or I by the way of M or K common intermediates for both the aza-Cope process but also the formation of the cyclic systems 4A,B. The passage from M to N is higher in energy than the one from M to L (1.83 Kcal.mol-1) while the energies between K and J is lower than the one between K and I (-11.39 Kcal.mol-1). This huge difference in energy could explain the dramatic change in reactivity. As a consequence, the phtalimidic derivative leading cleanly to the desired tricyclic product 4A and the maleimidic derivative giving way to the competitive aza-Cope reaction.
In conclusion, we have demonstrated that chiral amino-vinylsilane is suitable for the synthesis of aza-bicyclic templates. The efficiency of the N-acyliminium ions chemistry for the α-amidocyclization was shown to be substrate dependant, giving or not an aza-Cope rearrangement. The templates 4A,B obtained in three steps from the primary amines should be convenient intermediates for the access to both polyhydroxylated indolizidines and gephyrotoxins. Works are currently underway in our group in order to avoid the aza-Cope competitive reaction and to apply this methodology to the synthesis of alkaloids.
ACKNOWLEDGEMENTS
The authors thank the University of Le Havre, France, for the financial support for this research program.
References
1. A. S. Howard and J. P. Michael, ‘The Alkaloids: Simple indolizidine and quinolizidine alkaloids,’ Vol. 28; ed. by A. Brossi, Academic Press: New York, 1986, p. 183; H. Takahata and T. Momose, ‘The Alkaloids: Simple indolizidine alkaloids,’ Vol. 44, ed. by G. Cordell, Academic Press: San Diego, 1993, p. 189; J. P. Michael, Nat. Prod. Rep., 2007, 24, 191 and references cited therein. CrossRef
2. P. C. Das, J. D. Roberts, S. L. White, and K. Olden, Oncol. Res., 1995, 7, 425; P. E. Goss, C. L. Reid, D. Bailey, and J. W. Dennis, Clin. Cancer Res., 1997, 3, 1077; S. Wang, K. E. Panter, G. R. Holyoak, R. J. Molyneux, G. Lui, R. C. Evans, and T. D. Bunch, Anim. Reprod. Sci., 1999, 56, 19; CrossRef T. D. Heihtman, and A. T. Vasella, Angew. Chem., Int. Ed., 1999, 38, 750; CrossRef J. P. Michael, Nat. Prod. Rep., 2000, 17, 579; CrossRef V. H. Lillelund, H. H. Jensen, X. F. Liang, and M. Bols, Chem. Rev., 2002, 102, 515; CrossRef J. P. Michael, Nat. Prod. Rep., 2003, 20, 458; CrossRef J. P. Michael, Nat. Prod. Rep., 2005, 22, 603; CrossRef E. B. de Melo, A. de Silveria Gomes, and I. Carvalho, Tetrahedron, 2006, 62, 10277. CrossRef
3. N. Asano, R. J. Nash, R. J. Molyneux, and G. W. J. Fleet, Tetrahedron: Asymmetry, 2000, 11, 1645; CrossRef A. Watson, G. W. J. Fleet, N. Asano, R. J. Molyneux, and R. J. Nash, Phytochemistry, 2001, 56, 265; CrossRef N. Asano, Glycobiology, 2003, 13, 93R; CrossRef N. Asano, Curr. Top. Med. Chem., 2003, 3, 471; CrossRef L. Cipolla, B. L. Ferla, and F. Nicotra, Curr. Top. Med. Chem., 2003, 3, 485; CrossRef P. Greimel, J. Spreitz, A. E. Stütz, and T. M. Wrodnigg, Curr. Top. Med. Chem., 2003, 3, 513; CrossRef V. L. Schramm and P. C. Tyler, Curr. Top. Med. Chem., 2003, 3, 525; CrossRef P. Compain and O. R. Martin, Curr. Top. Med. Chem., 2003, 3, 541; CrossRef T. D. Butters, R. A. Dwek, and F. M. Platt, Curr. Top. Med. Chem., 2003, 3, 561; CrossRef S. Gerber-Lemaire and L. Juillerat-Jeanneret, Mini-Rev. Med. Chem., 2006, 6, 1043. CrossRef
4. J. W. Daly and T. F. Sande, ‘Alkaloids: Chemical and Biological Perspectives,’ Vol. 4, ed. by S. W. Pelletier, New York; 1986, Chapter 1; J. W. Daly, Cell Mol. Neurobiol., 2005, 25, 513. CrossRef
5. Š. Marchalín, J. Žúžiová, K. Kadlečíková, P. Šafář, P. Baran, V. Dalla, and A. Daïch, Tetrahedron Lett., 2007, 48, 697; CrossRef P. Šafář, J. Žúžiová, M. Bobošíková, Š. Marchalín, N. Prónayová, V. Dalla, and A. Daïch, Tetrahedron: Asymmetry, 2008, 19, 467 and references cited therein. CrossRef
6. B. H. Lee and M. F. Clothier, J. Org. Chem., 1997, 62, 1795; CrossRef K. Kiewel, M. Tallant, and G. A. Sulikowski, Tetrahedron Lett., 2001, 42, 6621; CrossRef M. Lennartz and E. Steckhan, Tetrahedron, 2001, 57, 675; CrossRef C. Gill, D. A. Greenhalgh, and N. S. Simpkins, Tetrahedron Lett., 2003, 44, 7803; CrossRef K. Kadlečíková, V. Dalla, Š. Marchalín, B. Decroix, and P. Baran, Tetrahedron, 2005, 61, 4743; CrossRef R. Schobert and A. Wicklein, Synthesis, 2007, 1499; CrossRef C. Camarero, I. González-Temprano, E. Lete, and N. Sotomayor, Synlett, 2007, 1101; CrossRef G. Lesma, A. Colombo, N. Landoni, A. Sacchetti, and A. Silvani, Tetrahedron: Asymmetry, 2007, 18, 1948. CrossRef
7. T. A. Blumenkopf and L. E. Overman, Chem. Rev., 1986, 86, 857; CrossRef C. Flann, T. C. Malone, and L. E. Overman, J. Am. Chem. Soc., 1987, 109, 6097; CrossRef G. W. Daub, D. A. Heerding, and L. E. Overman, Tetrahedron, 1988, 44, 3919; CrossRef S. Comesse, B. Bertin, and C. Kadouri-Puchot, Tetrahedron Lett., 2004, 45, 3807 and references cited therein. CrossRef
8. F. Pin, S. Comesse, B. Garrigues, Š. Marchalín, and A. Daïch, J. Org. Chem., 2007, 72, 1181. CrossRef
9. J. M. Takacs and J. J. Weidner, J. Org. Chem., 1994, 59, 6480; CrossRef F. Szemes Jr., A. Fousse, R. B. Othman, T. Bousquet, M. Othman, and V. Dalla, Synthesis, 2006, 875. CrossRef
10. Procedures for the π-cyclization. Protocol (a): BF3.OEt2 (130 μL, 1.0 mmol) was added at room temperature to a solution of hydroxy lactam 5Aa or 5Ba (0.5 mmol) in CH2Cl2 (5 mL). After one night of stirring, the reaction was cooled to 0 °C and carefully quenched by addition of a saturated aqueous solution of NaHCO3 (5 mL). The aqueous layer was extracted with CH2Cl2 (3×5 mL), the organic layers were combined, dried over MgSO4 and evaporated. The residue was then purified by chromatography on SiO2 column using AcOEt/cyclohexane as eluenting mixture and provided cycles 4A and 4B in 70% and 25% yields, respectively, for two steps. Protocol (b): Bi(OTf)3 (0.01 equiv) was added in one portion to a stirred solution of acetoxy lactam 5Ab (197 mg, 0.5 mmol) in MeCN (2.5 mL). When the reaction was over (monitored by TLC) a saturated aqueous solution with NaHCO3 was added. The aqueous layer was then extracted with CH2Cl2 (3×5 mL), the organic layers were combined, dried over MgSO4 and evaporated under vacuo. The residue was than purified by chromatography on SiO2 column using same eluent as outlined above to provide in 69% yield compound 4A. This product was isolated as a colorless gummy. (AcOEt/cyclohexane, 30:70). [α]20D -88 (c 0.5, CHCl3). 1H NMR (200 MHz, CDCl3): 7.86 (d, J=7.8Hz, 1H), 7.52-7.24 (m, 8H), 5.98 (s, 2H), 5.87 (t, J=3.9Hz, 1H), 4.70-4.63 (m, 1H), 2.72 (bs, 2H). 13C NMR (50 MHz, CDCl3): 167.2, 144.0, 140.0, 132.0, 131.7, 128.7, 128.3, 127.5, 127.3, 125.6, 125.2, 124.0, 122.1, 54.7, 48.6, 27.6. Data for 4B. This product was isolated as a colorless gummy from hydroxy lactam 5Ba by using Protocol (a). (AcOEt/cyclohexane, 30:70). 1H NMR (200 MHz, CDCl3): 7.40-7.26 (m, 5H), 7.04 (dd, J=5.4 and 2.3Hz, 1H), 6.17 (dd, J=5.4 and 2.3Hz, 1H), 5.99 (dq, J=10.1 and 2.3Hz, 1H), 5.94 (dd, J=10.2 and 2.3Hz, 1H), 5.68 (t, J=4.8Hz, 1H), 4.42-4.42 (m, 1H), 2.70-2.61 (m, 2H). 13C NMR (50 MHz, CDCl3): 167.3, 147.3, 140.8, 139.4, 133.9, 134.2, 129.1, 127.9, 122.7, 58.20, 48.79, 28.03.
11. Determined by 1H-NMR analysis on the crude mixture.
12. See for example: H. Ent, H. de Koning, and W. N. Speckamp, Heterocycles, 1988, 27, 237; CrossRef C. Y. Hong, N. Kado, and L. E. Overman, J. Am. Chem. Soc., 1993, 115, 11028; CrossRef T. K. Yang, T. F. Teng, J. H. Lin, and Y. Y.Lay, Tetrahedron Lett., 1994, 35, 3581; CrossRef W. J. Kim, E. H. Kim, N. S. Song, J. K. Shin, and B. Y. Cho, Synlett, 1998, 921. CrossRef
13. For previous work of two of us, see: C. Agami, S. Comesse, C. Kadouri-Puchot, and M. Lusinchi, Synlett, 1999, 1094; CrossRef C. Agami, S. Comesse, and C. Kadouri-Puchot, J. Org. Chem., 2000, 65, 4435. CrossRef
14. Calculations of the structures and energies of the different species were performed using the semi-empirical AM1 Hamiltonian in the AMPAC (ref. 15) or GAMESS (ref. 16) suite of programs.
15. M. J. S. Dewar, E. G. Zoebisch, E. F. Healy, and J. J. P. Stewart, J. Am. Chem. Soc., 1985, 107, 3902. CrossRef
16. M. W. Schmidt, K. K. Baldridge, J. A. Boatz, S. T. Elbert, M. S. Gordon, J. J. Jensen, S. Koseki, N. Matsunaya, K. A. Nguyen, S. Su, T. L. Windus, M. Dupuis, and J. A. Montgomery, J. Comput. Chem., 1993, 14, 1347. CrossRef
17. C. Agami, S. Comesse, and C. Kadouri-Puchot, J. Org. Chem., 2002, 67, 2424 CrossRef