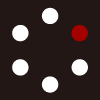
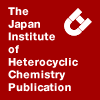
HETEROCYCLES
An International Journal for Reviews and Communications in Heterocyclic ChemistryWeb Edition ISSN: 1881-0942
Published online by The Japan Institute of Heterocyclic Chemistry
e-Journal
Full Text HTML
Received, 27th February, 2009, Accepted, 13th April, 2009, Published online, 14th April, 2009.
DOI: 10.3987/COM-09-11693
■ α’-Oxoperakensimines A - C, New Bisbenzylisoquinoline Alkaloids from Alseodaphne perakensis (Gamble) Kosterm
Mat Ropi Mukhtar, Mohd Azlan Nafiah, Khalijah Awang, Noel F. Thomas, Kazumasa Zaima, Hiroshi Morita, Marc Litaudon, and A. Hamid A. Hadi*
Department of Chemistry, Faculty of Science, University of Malaya, Pantai Valley, 50603 Kuala Lumpur, Malaysia
Abstract
Three new bisbenzylisoquinolines, α′-oxoperakensimines A – C (1 – 3) have been isolated from the bark of Alseodaphne perakensis (Gamble) Kosterm (Lauraceae). Their structures were elucidated by two-dimensional NMR techniques. α′-Oxoperakensimines A – C (1 – 3) showed vasorelaxant activity on rat aorta.INTRODUCTION
Plants belonging to Lauraceae are known to produce various bisbenzylisoquinoline alkaloids with pharmacological activities.1 A large number of bisbenzylisoquinoline alkaloids have been isolated from various plant resources and the medicinal properties such as antimalarial,2 antihypotensive,3 antitumor4 and anti-inflammatory5 effects, have been reported.
In continuation of our investigation on this family, we have embarked a study on the dichloromethane extract of the bark of Alseodaphne perakensis (Gamble) Kosterm which led to the isolation of three new bisbenzylisoquinolines, α′-oxoperakensimines A – C (1 – 3). This paper reports the structural elucidation of α′-oxoperakensimines A – C (1 – 3) and their vasorelaxant activity on rat aorta.
RESULTS AND DISCUSSION
The dichloromethane extract of the bark was subjected to silica gel column chromatography and PTLC yielding three new bisbenzylisoquinolines, α′-oxoperakensimines A – C (1 – 3).
α′-Oxoperakensimine A (1), [α]D26 -25 (c 1.0, MeOH), was isolated as a brown amorphous powder. The HRFABMS showed the pseudomolecular ion peak at m/z 623.2730 (M+H)+, corresponding to the molecular formula of C37H38N2O7 thus suggesting a dimer of bisbenzylisoquinoline type. IR absorption showed the presence of hydroxyl (3696 cm-1) and imine (1737 cm-1) groups. The 1H NMR spectrum showed the presence of two aromatic singlets at δH 6.41 and 6.74 characteristic of H-5 and H-5′, and an N-methyl singlet (δH 2.46).6 Another two singlets at δH 6.38 and 6.47 which were assignable to H-8 and H-10, respectively, were also observed. In addition two sets of doublet with coupling constant of 8.1 Hz which were apparent at δH 6.87 and 7.97. These signals may be attributed to H-10′, H-11′, H-13′, and H-14′, thus indicating that ring C′ is para disubstituted (AA′BB′ system).7 The overlapped signals of H-8′ and H-14 were observed at δH 6.95. Another doublet at δH 6.86 (J = 8.0 Hz) was corresponding to proton H-13. The presence of four methoxy groups was indicated by four singlets at δH 3.75 (6-OCH3), 3.77 (12-OCH3), 3.79 (7′-OCH3), and 3.92 (6′-OCH3).
The 13C NMR spectrum showed the chemical shifts of eleven aromatic carbons between δC 132.7 and 109.8. Four methoxy carbons were assigned at δC 55.5, 55.9, 56.0, and 56.1, as well as one methyl carbon atom at δC 42.9. The 13C NMR spectrum also indicated the presence of a carbonyl carbon at δC 192.5 (C-α′) and an imine carbon at δC 164.5 (C-1′). Base on the HMBC correlation of H-14' and H-10', it showed that C-12' resonated at δC 162.5. The NMR spectrum also revealed the presence of fifteen quaternary and ten aliphatic carbons.
Selected 2D NMR correlations for α′-oxoperakensimine A (1) were shown in Figure 1. The position of Δ1′-N′ double bond was confirmed by the HMBC correlation of H-3′ to C-1′ (δC 164.5). Another peak at δC 162.5 was assignable to C-12′ by the HMBC correlations from H-10′ (J3), H-11′ (J2), H-13′ (J2), and H-14′ (J3). The presence of a carbonyl group at C-α′ was confirmed based on the HMBC correlations of H-10′ (δH 7.97) and H-14′ (δH 7.97) to C-α′ (δC 192.5). HMBC correlations of the methyl peak at δH 2.46 to C-1 (δC 64.4) and C-3 (δC 48.4) suggested that the methyl group was attached to N-2. Two methoxy groups at δC 55.9 and δC 55.5 were attached to C-6′ (δC 151.8) and C-7′ (δC 147.6) by the HMBC correlations of H-5′ to C-7′ (J3) and C-6′ (J2), and H-8′ to C-6′ (J3) and C-4a (δC 131.2, J3), respectively. The presence of a methoxy group at δC 56.1 which was attached to C-6 (δC 145.3) was confirmed based on the HMBC correlations of H-8 (δH 6.38) to C-6, C-4a (δC 129.8), and C-1. In the ring-C, the presence of another methoxy group at δC 56.0 attached to C-12 was indicated by the HMBC correlations of H-10 to C-α (δC 39.4), C-12 (δC 149.1), and C-14 (δC 126.8), and H-14 to C-α, C-12, and C-10 (δC 122.1).
The NOESY spectrum showed correlations of an N-CH3 /H-14 and H-5. The relative configuration at C-1 was deduced by the NOESY correlations among H-1, H-8, Hβ-α, and H-14. For the protons at ring B′, the NOESY spectrum showed the correlations of H-5′ with H-4′ and 6′-OCH3. At ring C′, the NOESY spectrum showed a correlation between H-10′ and 12-OCH3. Thus, the relative configuration of 1 was depicted as in Figure 2. The absolute configuration of N-methylbenzyl isoquinoline alkaloids such as pecrassipines A and B,8 karakoramine,9 and vietanamine,10 has already been determined as R configuration. The negative sign of the specific rotation indicated that the absolute configuration of α′-Oxoperakensimine A (1) at C-1 was R.
α′-Oxoperakensimine B (2), [α]D26 -44 (c 1.0, MeOH), revealed the pseudomolecular ion peak at m/z 623.2739 (M+H)+ in the HRFABMS, corresponding to the molecular formula of C37H38N2O7. IR spectrum showed the presence of hydroxyl group (3696 cm-1). The 1H and 13C NMR data of 2 were reminiscent to those of 1. The chemical shift at C-7 bearing a methoxy group was observed at δC 146.5, which was correlated with H-5 (δH 6.52) in the HMBC spectrum. In addition, an HMBC cross peak between H-5′ and C-7′ bearing a hydroxyl group indicated the presence of a hydroxyl group at C-7′ (δC 144.3). α′-Oxoperakensimine C (3), [α]D26 -11 (c 1.0, MeOH), has a molecular formula of C36H36N2O7 determined by the pseudomolecular ion peak at m/z 609.2582 (M+H)+ in the HRFABMS. The 1H and 13C NMR spectra were analogous to those of 1. However, the hydroxyl group was detected instead of a methoxy group at C-7′ (δC 144.4). This was confirmed by the presence of three methoxy protons at δH 3.75 (6-OCH3), 3.76 (12-OCH3) and 3.92 (6′-OCH3), and the cross peak of the HMBC correlations of H-5′ (δH 6.71) to C-4′ (δC 25.5), C-6′ (δC 149.4), C-7′, and C-8′a (δC 120.0).
The vasodilators are useful for treatment of cerebral vasospasm and hypertension, and for improvement of peripheral circulation.11 When phenylephrine (PE) 3 × 10-7 M was applied to thoracic aortic rings with endothelium after achieving a maximal response, we added α′-oxoperakensimines A – C (1 – 3). These bisbenzylisoquinoline alkaloids showed a moderate vasorelaxant activity on isolated rat aorta (Figure 3). Among them, α′-oxoperakensimines A (1) and B (2) showed relatively potent vasorelaxant activity, whereas α′-oxoperakensimine C (3) showed slow vasorelaxant actions. Vasodilation may seem to be influenced by the hydrophobicity of C-7 and C-7′. The mode of actions of these bisbenzylisoquinoline alkaloids on vasorelaxant activities are under investigation.
EXPERIMENTAL
General Experimental Procedures. Merck silica gel 60 (230–400 mesh) and GF254 were used for column chromatography separations, silica gel 60 F254 for TLC, and silica gel 604 F254 for PTLC. NMR spectra were recorded on JEOL ECA (400 MHz) using CDCl3 as a solvent. IR spectra were measured using Perkin-Elmer 1600 Double Beam. The ultraviolet absorption spectra were recorded on UV-VIS NIR Scanning Spectrophotometer (Shimadzu UV-310 IPC) with methanol as a solvent.
Plant Material. The barks of Alseodaphne perakensis (Gamble) Kosterm were collected at Hutan Simpan Temau, Ulu Jelai, Kuala Lipis, Pahang, Malaysia. The botanical identification was made by Mr. Teo Leong Eng, Faculty of Science, University of Malaya. Voucher specimens (KL 5135) were deposited in the Herbarium of Chemistry Department, University of Malaya, Kuala Lumpur, Malaysia.
Extraction and Isolation. The dried, grounded bark of the plant (3.0 kg) was first defatted with hexane for 60 hours. The residual plant material was dried up and left for 2 h after moistening with 25% NH4OH. They were re-extracted with CH2Cl2 by Soxhlet extractor for 17 h. After filtration, the supernatant was concentrated to 500 mL at room temperature (30 oC) followed by acidic extraction with 5% HCl until a negative result obtained from the Mayer’s test. The aqueous solution was basified with NH4OH to pH 11 and re-extracted with CH2Cl2. This was followed by washing with distilled H2O, dried over anhydrous sodium sulphate, and evaporated to give an alkaloid fraction (10 g). The crude alkaloid (5.0 g) was subjected to column chromatography over silica gel using CH2Cl2 gradually enriched with methanol. Three bisbenzylisoquinoline alkaloids, α′-oxoperakensimines A - C (1 - 3), were isolated by CC on silica gel with CH2Cl2-MeOH solvent system and were purified by preparative TLC (98:2; CH2Cl2:MeOH) purified by ammonia.
α′-Oxoperakensimine A (1). Brown amorphous powder, [α]D26 -25 (c 1.0, MeOH), HRFABMS m/z 623.2730 (M+H; calcd for C37H39N2O7, 623.2757); UV (MeOH) λmax 213 and 285 nm. IR (CHCl3) νmax 3696, 3299, 2981, and 1737 cm-1; 1H and 13C NMR: Table 1.
α′-Oxoperakensimine B (2). Brown amorphous powder, [α]D26 -44 (c 1.0, MeOH), HRFABMS m/z 623.2739 (M+H; calcd for C37H39N2O7, 623.2757); UV (MeOH) λmax 213 and 285 nm. IR (CHCl3) νmax 3696, 3299, 2981, and 1727 cm-1; 1H and 13C NMR: Table 1.
α′-Oxoperakensimine C (3). Brown amorphous powder, [α]D26 -11 (c 1.0, MeOH), HRFABMS m/z 609.2582 (M+H; calcd for C36H37N2O7, 609.2601); UV (MeOH) λmax 218 and 288 nm. IR (CHCl3) νmax 3680, 3315, and 2864 cm-1; 1H and 13C NMR: Table 1.
Vasodilation Assay.10 A male Wistar rat weighting 260 g was sacrificed by bleeding from carotid arteries under an anesthetization. A section of the thoracic aorta between the aortic arch and the diaphragm was removed and placed in oxygenated, modified Krebs-Henseleit solution (KHS: 118.0 mM NaCl, 4.7 mM KCl, 25.0 mM NaHCO3, 1.8 mM CaCl2, 1.2 mM NaH2PO4, 1.2 mM MgSO4, and 11.0 mM glucose). The aorta was cleaned of loosely adhering fat and connective tissue and cut into ring preparations 3 mm in length. The tissue was placed in a well-oxygenated (95% O2, 5% CO2) bath of 5 mL KHS solution at 37 °C with one end connected to a tissue holder and the other to a force-displacement transducer (Nihon Kohden, TB-611T). The tissue was equilibrated for 60 min under a resting tension of 1.0 g. During this time the KHS in the tissue bath was replaced every 20 min.
After equilibration, each aortic ring was contracted by treatment with 3 x 10-7 M PE. The presence of functional endothelial cells was confirmed by demonstrating relaxation to 10-5 M acetylcholine (ACh), and aortic ring in which 80% relaxation occurred, were regarded as tissues with endothelium. When the PE-induced contraction reached a plateau, each sample (1 – 3, 10-4 M) was added.
These animal experimental studies were conducted in accordance with the Guiding Principles for the Care and Use of Laboratory Animals, Hoshi University and under the supervision of the Committee on Animal Research of Hoshi University, which is accredited by the Ministry of Education, Science, Sports Culture, and Technology of Japan.
ACKNOWLEDGMENTS
This work was supported by a Science Fund (12-02-03-2063), the Grant-in-Aid for Scientific Research from the Ministry of Education, Science, Sports, Culture, and Technology of Japan, and The Open Research Center Project. The author like to thanks to Mr. Teo Leong Eng, Pok Din, Hazri, and Rafly from Herbarium Group of Chemistry Department, University Malaya, Kuala Lumpur, Malaysia.
References
1. S. Tapan and M. Biswapati, Journal of Medicinal and Aromatic Plant Sciences, 1997, 19, 32.
2. K. Likhitwitayawaid, C. K. Angerhofer, G. A. Cordell, J. M. Pezzuto, and N. Ruangrungsi, J. Nat. Prod., 1993, 56, 22. CrossRef
3. W. N. Wu, J. L. Beal, G. W. Clark, L. A. Mitscher, K. N. Salman, and P. Patil, Chem. Abstr., 1976, 39, 204.
4. Y. Xu and J. Ni, Chem. Abstr., 1986, 31, 1710.
5. W. K. Seow, A. Ferrante, D. B. H. Goh, A. H. Chalmers, S. Li, and Y.H. Thong, Int. Arch Allergy Apopl Immunol., 1988, 85, 410.
6. T. J. Hsieh, C. Y. Chen, R. Y. Kuo, F. R. Chang, and Y. C. Wu, J. Nat. Prod., 1999, 62, 1192. CrossRef
7. C. Diego and D. Henry, J. Nat. Prod., 1987, 50, 910. CrossRef
8. K. Awang, S. S. S. A. Azziz, A. H. A. Hadi, H. Morita, Y. Hirasawa, T. Iizuka, M. Litaudon, and M. R. Mukhtar, Heterocycles, 2007, 71, 2055. CrossRef
9. J. E. Leet, V. Elango, S. F. Hussain, and M. Shamma, Heterocycles, 1983, 20, 425. CrossRef
10. N. T. Nghia, I. Valka, E. Weigl, V. Simanek, D. Cortes, and A. Cave, Fitoterapia, 1991, LXII, 315.
11. H. Morita, T. Iizuka, C. Y. Choo, K. L. Chan, K. Takeya, and J. Kobayashi, Bioorg. Med. Chem. Lett., 2006, 16, 4609. CrossRef