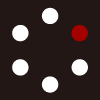
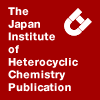
HETEROCYCLES
An International Journal for Reviews and Communications in Heterocyclic ChemistryWeb Edition ISSN: 1881-0942
Published online by The Japan Institute of Heterocyclic Chemistry
e-Journal
Full Text HTML
Received, 13th August, 2009, Accepted, 25th September, 2009, Published online, 25th September, 2009.
DOI: 10.3987/COM-09-S(S)111
■ Synthesis of Zanthoxyline and Its Related Compounds: Revision of the Reported Structure
Hitoshi Abe,* Naoko Kobayashi, Yasuo Takeuchi, and Takashi Harayama*
Department of Applied Chemistry, Faculty of Engineering, Toyama University, Gofuku 3190, Toyama 930-8555, Japan
Abstract
Benzo[c]phenanthridine alkaloid, zanthoxyline, was synthesized through an intramolecular biaryl coupling reaction of N-naphthylbenzamide derivative using a palladium reagent. Comparison of the NMR data for the synthetic product with that reported previously revealed that the proposed structure was incorrect. Synthesis of the related compounds suggested that the correct structure of zanthoxyline is the previously known decarine.Zanthoxyline (1) is a benzo[c]phenanthridine alkaloid that was isolated in 1997 from Zanthoxylum rhoifolium; it possesses four oxygen functional groups at positions 2, 3, 9 and 10 (Figure 1).1 This substituent pattern is a very rare case in the class of natural benzo[c]phenanthridines.2 Thus, we were interested in the biosynthesis of zanthoxyline (1), and in order to investigate the detailed features of this natural alkaloid, its chemical synthesis was necessary.
We have studied intensively the palladium-mediated intramolecular biaryl coupling reaction of benzanilide derivatives,3 and this technique has been used for the total synthesis of various natural benzo[c]phenanthridine alkaloids and their related compounds.4 Thus, we reasoned that the same strategy could also be useful for the synthesis of 1.
Initially, naphthamide 2 was prepared by the simple amidation between benzoic acid and naphthylamine,5 followed by the protection of the amide nitrogen with the MOM group (Scheme 1). The intramolecular coupling6 of 2 was successful for the generation of tetracyclic compound 3 when Pd(OAc)2 (20% mol) was used with PPh3 (40% mol) and Ag2CO3 (200% mol) as a ligand and a base.7 The reduction of the amide moiety and successive deprotection of the MOM and isopropyl groups were carried out to complete the synthesis of zanthoxyline (1).
Unfortunately, the NMR data for the synthetic 1 were not identical with the reported data (vide infra); thus, we supposed that the reported structure of zanthoxyline was incorrect. The plausible structure of the correct zanthoxyline was expected to be 10-hydroxy-9-methoxy compound 6, so we attempted the synthesis of 6. The same synthetic method (Scheme 2) was employed to obtain compound 6: the intramolecular biaryl coupling of amide 4, reduction of the lactam 5 with LiAlH4, and the deprotection of the MOM and isopropyl groups.
A comparison of the NMR data for 6 with the reported data (vide infra) revealed that 6 is different from the natural zanthoxyline.
At this stage, since neither 1 nor 6 were the correct structure of zanthoxyline, we were convinced that the position of the oxygen functional group was incorrect for the reported zanthoxyline. In this context, the spectral data for the two known compounds, isodecarine (7)8 and decarine (8),9 needed to be examined (Figure 2).
The synthesis of isodecarine (7) was performed via the synthetic strategy illustrated in Scheme 3. The N-protected amide (9) was subjected to the Pd-mediated intramolecular biaryl coupling reaction, and the generated lactam (10) was transformed into 7 by LiAlH4 reduction and the deprotection sequence. We already had a sample of decarine (8) in hand, since we synthesized this compound previously.4c
In order to compare the NMR data for synthetic compounds 1, 6, 7, and 8 with the reported values, all chemical shifts for the 1H- and 13C-NMR data were compiled in Table 1 and Table 2, respectively. While the values for 1, 6, and 7 are obviously different from those of the reported zanthoxyline, the δ values for 8 are very similar to the reported values.
From the above results, we propose that the correct structure of natural zanthoxyline is not 1, but 8, which is the known decarine.
In conclusion, we have synthesized the reported zanthoxyline and its related compounds, and compared all chemical shifts from their NMR data. As a result, the correct structure of the natural zanthoxyline is the 8-hydroxy-7-methoxy compound, decarine.
ACKNOWLEDGEMENTS
The authors thank the SC-NMR Laboratory of Okayama University for the NMR experiments.
References
1. N. F. DeMoura, H. B. Ribeiro, E. C. S. Machado, E. M. Ethur, N. Zanatta, and A. D. Morel, Phytochemistry, 1997, 46, 1443. CrossRef
2. K. W. Bentley, Nat. Prod. Rep., 1999, 16, 367. CrossRef
3. (a) H. Nishioka, C. Nagura, H. Abe, Y. Takeuchi, and T. Harayama, Heterocycles, 2006, 70, 549; CrossRef (b) T. Harayama, A. Hori, H. Abe, and Y. Takeuchi, Heterocycles, 2006, 67, 385; CrossRef (c) T. Harayama, Yakugaku Zasshi, 2006, 126, 543; CrossRef (d) T. Harayama, T. Sato, A. Hori, H. Abe, and Y. Takeuchi, Heterocycles, 2005, 66, 527; CrossRef (e) T. Harayama, Y. Kawata, C. Nagura, T. Sato, T. Miyagoe, H. Abe, and Y. Takeuchi, Tetrahedron Lett., 2005, 46, 6091; CrossRef (f) H. Nishioka, Y. Shoujiguchi, H. Abe, Y. Takeuchi, and T. Harayama, Heterocycles, 2004, 64, 463; CrossRef (g) T. Harayama, A. Hori, H. Abe, and Y. Takeuchi, Tetrahedron, 2004, 60, 1611; CrossRef (h) T. Harayama, H. Toko, A. Hori, T. Miyagoe, T. Sato, H. Nishioka, H. Abe, and Y. Takeuchi, Heterocycles, 2003, 61, 513; CrossRef (i) T. Harayama, A. Hori, H. Abe, and Y. Takeuchi, Heterocycles, 2003, 60, 2429; CrossRef (j) T. Harayama, H. Toko, K. Kubota, H. Nishioka, H. Abe, and Y. Takeuchi, Heterocycles, 2002, 58, 175; CrossRef (k) T. Harayama, A. Hori, Y. Nakano, T. Akiyama, H. Abe, and Y. Takeuchi, Heterocycles, 2002, 58, 159; CrossRef (l) T. Harayama, T. Akiyama, Y. Nakano, H. Nishioka, H. Abe, and Y. Takeuchi, Chem. Parm. Bull., 2002, 50, 519. CrossRef
4. (a) T. Harayama, Heterocycles, 2005, 65, 697; CrossRef (b) T. Harayama, T. Sato, A. Hori, H. Abe, and Y. Takeuchi, Synthesis, 2004, 1446; CrossRef (c) T. Harayama, T. Sato, Y. Nakano, H. Abe, and Y. Takeuchi, Heterocycles, 2003, 59, 293; CrossRef (d) T. Harayama, T. Akiyama, Y. Nakano, K. Shibaike, H. Akamatsu, A. Hori, H. Abe, and Y. Takeuchi, Synthesis, 2002, 237; CrossRef (e) T. Harayama, T. Akiyama, H. Akamatsu, K. Kawano, H. Abe, and Y. Takeuchi, Synthesis, 2001, 444; CrossRef (f) T. Harayama, H. Akamatsu, K. Okamura, T. Miyagoe, T. Akiyama, H. Abe, and Y. Takeuchi, J. Chem. Soc., Perkin Trans. 1, 2001, 523. CrossRef
5. J. Smidrkal, Coll. Czech. Chem. Commun., 1988, 53, 3184.
6. For recent reviews of biaryl coupling reaction, see: (a) L.-C. Campeau, D. R. Stuart, and K. Fagnou, Aldricimica Acta, 2007, 40, 35; (b) J. Hassan, M. Sevignon, C. Gozzi, E. Schulz, and M. Lemaire, Chem. Rev., 2002, 102, 1359. CrossRef
7. Typical procedure for the Pd-mediated biaryl coupling reaction: A mixture of amide (0.18 mmol), PPh3 (0.072 mmol), Ag2CO3 (0.36 mmol), Pd(OAc)2 (0.036 mmol), and dry DMF (2 mL) was heated under reflux for 1 h. After cooling, the mixture was diluted with ethyl acetate and the solid material was removed by filtration. The filtrate was washed with brine, dried over MgSO4, and evaporated. After the residue was subjected to silica gel column chromatography, recrystallization from ethyl acetate was carried out to afford yellow needles.
8. (a) K. Kohno, S. Azuma, T. Choshi, J. Nobuhiro, and S. Hibino, Tetrahedron Lett., 2009, 50, 590; CrossRef (b) J. Styskala, P. Cankar, M. Soural, J. Hlavac, P. Hradil, J. Vicar, and V. Simanek, Heterocycles, 2007, 73, 769. CrossRef
9. (a) S. V. Kesser, Y. P. Gupta, P. Balakrishnan, K. K. Sawal, T. Mohammad, and M. Dutt, J. Org. Chem., 1988, 53, 1708; CrossRef (b) S. V. Kesser, Y. P. Gupta, A. Khurana, and K. K. Sawal, Heterocycles, 1984, 22, 2723; CrossRef (c) J. Vaquette, J.-L. Pouset, R.-R. Paris, and A. Cave, Phytochemistry, 1974, 13, 1257. CrossRef