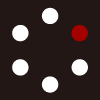
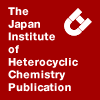
HETEROCYCLES
An International Journal for Reviews and Communications in Heterocyclic ChemistryWeb Edition ISSN: 1881-0942
Published online by The Japan Institute of Heterocyclic Chemistry
e-Journal
Full Text HTML
Received, 14th July, 2009, Accepted, 31st August, 2009, Published online, 31st August, 2009.
DOI: 10.3987/COM-09-S(S)52
■ Glycosides from Vallaris solanaceae with TRAIL-Resistance-Overcoming Activity
Firoj Ahmed, Samir Kumar Sadhu, Takashi Ohtsuki, Amina Khatun, and Masami Ishibashi*
Graduate School of Pharmaceutical Science, Chiba University, 1-33 Yayoi-cho, Inage-ku, Chiba 263-8522, Japan
Abstract
A new cardenolide glycoside, vallarisoside (1), and a known one, 3β-O-(α-acofriosyl)-16-anhydrogitoxigenin (2) along with a new glycoside, benzyl 2-O-β-apiofuranosyl-(1→2)-β-D-glucopyranosyl-2, 6-dihydroxy-benzoate (3) were isolated from Vallaris solanaceae. Structures of the isolates were elucidated by 1D and 2D NMR and mass spectroscopic analysis. Of the isolates, vallarisoside (1) showed potent TRAIL-resistance-overcoming activity in human gastric adenocarcinoma (AGS) cells and cell-growth-inhibitory activity against HeLa and SW480 cells.INTRODUCTION
Vallaris solanaceae Kuntze (syn.: Vallaris heynei) (Apocynaceae), locally known as ‘agarmoni’ grows in Bangladesh and in other Southeast Asian countries. It is a creeper with fragrant white flowers, traditionally used against ring worms and skin infections.1 Previous chemical investigation of its leaves reported to isolate β-sitosterol, β-amyrin, ursolic acid,2 and triterpenes;3 vallaroside, solanoside, vallarosolanoside, and acoshimperosid P.4-5 In our continuous investigation on traditional medicinal plants of Southeast Asia, we isolated a new cardenolide glycoside, vallarisoside (1), and a known one, 3β-O-(α-acofriosyl)-16-anhydrogitoxigenin (2) along with a new glycoside, benzyl 2-O-β-apiofuranosyl-(1→2)-β-D-glucopyranosyl-2, 6-dihydroxy-benzoate (3) from this herb. We report here the isolation and structure determination together with the cytotoxic activity and TRAIL-resistance-overcoming activity of the isolated compounds.
RESULTS AND DISCUSSION
The MeOH extract of the dried aerial parts (without flower) of V. solanaceae was partitioned between n-hexane, EtOAc and n-BuOH, followed by repeated column chromatography and preparative HPLC to afford compounds 1-3.
Compound 1 was isolated as optically active, colorless solid, and was assigned the molecular formula C32H46O9 on the basis of HRFABMS (m/z 597.3008 [M+Na]+, ∆ mmu − 3.2) and 1H and 13C NMR spectroscopic data (Table 1). The IR spectrum showed the presence of an α, β-unsaturated lactone (1740 cm−1), a strong hydroxyl absorption (3450 cm−1), and carbon-carbon double bond(s) (1620 cm−1). In the 1H NMR spectrum, two double doublets (each 1H) at δ 5.14 (J = 16.5 and 1.6 Hz) and 5.00 (J = 16.5 and 1.6 Hz) were attributed to H-21a and H-21b, and a 1H broad singlet at δ 6.00 was assigned to H-22, whereas, two methyl singlets at δ 1.27 and 1.00 were attributed to H3-18 and H3-19, respectively. The presence of an α, β-unsaturated γ-lactone with further conjugation was also evident from its UV absorption maxima at 270 nm.6 The double bond was placed at C-16/17 position, both on the basis of HMBC correlation from H-16 (δ 6.25, t, J = 2.7 Hz) to C-13 (δ 53.4), C-14(δ 86.7), and C-20 (δ 161.9), and considering that the UV maximum was at 270 nm. This assignment was further confirmed from two double doublets at δ 2.77 (dd, J = 18.3 and 3.3 Hz, H-15a) and 2.30 (dd, J = 18.3 and 3.3 Hz, H-15b). A broad singlet at δ 3.96 was assigned to the carbinylic proton H-3. The 1H and 13C NMR data of 1, thus revealed an identical aglycone to that of 16-anhydrogitoxigenin.7
In addition, the 1H and 13C NMR data (Table 1) indicated the presence of one molecule of sugar because of an anomeric proton at δ 4.81 for H-1’ (d, J = 1.7 Hz) connected to the anomeric carbon at δ 97.3 in the HMQC spectrum. The INEPT data for J C1’- H1’ was observed to be 170 Hz indicating the α-linkage of the sugar.8 The connectivity of all carbons with their corresponding protons of the sugar moiety was assigned by the HMBC and COSY spectrum (Figure 2a), and from the coupling constants. H-2’ proton, connected to C-2’(δ 70.3), showed a double doublet at δ 5.18 (J = 3.4 and 1.7 Hz); H-3’, connected to C-3’(δ 80.7), appeared as a 1H double doublet at δ 3.46 (J = 9.5 and 3.4 Hz); H-4’, connected to C-4’(δ 73.2), appeared as a 1H triplet at δ 3.35 (J = 9.5 Hz); H-5’, connected to C-5’(δ 70.0), appeared as a doublet of quartet at δ 3.70 (J = 9.5 and 6.4 Hz) whereas H3-6’, connected to C-6’(δ 18.0), resonated as a 3H doublet at δ 1.23 (J = 6.4 Hz). The OMe group (δC 58.0; δH 3.40, s) was assigned to be linked to C-3’ on the basis of the HMBC correlation from the methoxy protons at δ 3.40 to C-3’ at δ 80.7. From these data the structure of the sugar was determined as α-acofriose (3-O-methyl-α-rhamnose).9 Besides, presence of an acetyl moiety was confirmed from the HMBC correlation from the methyl singlet at δ 2.07 to the carbonyl carbon at δ 172.0. The HMBC correlation from H-2’ to this carbonyl carbon indicated that the acetyl group was connected at C-2’ of acofriose. Thus, the sugar was characterized as 2’-O-acetyl-α-acofriose.
Based on the identical 1H and 13C NMR data, aglycone of 1 was proposed to confer the same configuration as that of 16-anhydrogitoxigenin (3β-O, 5β, 8β, 9α, 14β-OH, 10β-CH3, and 13β- CH3) and was further confirmed by differential NOE experiments (Figure 2b). Irradiation of both H3-18 (δ 1.27) and H3-19 (δ 1.00) showed NOEs in H-8 (δ 1.76, m), and irradiation of H3-19 (δ 1.00) showed NOEs in H-5 (δ 1.63, m) and H-8 indicating their same orientation. The upfield chemical shift of C-9 (δ 37.6) was due to the steric interaction between its axial hydrogen and C(14) - C(15) bond. Due to the equatorial β-effect of the C-14β hydroxyl group, C-8 was resonated at a relatively lower field at δ 42.1.10 Thus, compound 1 was assigned the structure 3β-O- (2’-O-acetyl-α-acofriosyl)-16-anhydrogitoxigenin.
Compound 2, a cardenolide glycoside, was isolated as optically active, colorless solid, and was assigned the molecular formula C30H44O8 on the basis of FABMS (m/z 571 [M+K]+), 1H and 13C NMR
spectroscopic data (Table 1). These data were similar to those of compound 1 except for the absence of signals due to a 2’-O-acetyl moiety (carbonyl carbon at δC 172.0, a methyl singlet at δC 20.8 with its corresponding proton at δH 2.07 observed for 1) and an up-field shift of H-2’at δH 4.02 (m) compared to the H-2’ (δ 5.18, J = 3.4, 1.7 Hz) of 1 (Table 1). Thus, 2 was identified as 3β-O-(α-acofriosyl)- 16- -anhydrogitoxigenin, which was formerly reported as 16-desacetyl-16-anhydroacoshimperosid P by Kaufmann.5 Here, we report, for the first time the 1H and 13C NMR spectroscopic data of this compound (Table 1).
Compound 3 was isolated as optically active, colorless oil, and was assigned the molecular formula C25H30O13 on the basis of HRFABMS (m/z 561.1593 [M+Na]+, ∆ mmu +0.9), and 1H and 13C NMR spectroscopic data (Table 2). The 1H NMR spectrum showed three aromatic protons as two double doublets at δ 6.65 and 6.55, and a triplet at δ 7.24, along with five further aromatic protons in the 7.55–7.30 ppm range. Besides, three singlets at δ 5.43 and 3.51, two anomeric protons at δ 5.08 (d, J=7.2 Hz) and δ 5.37 (d, J=2.0 Hz), three multiplets at δ 3.88, 3.82 and 3.66, one triplet at δ 3.56, and five overlapping protons in the δ 3.45–3.33 ppm range were also present in the spectrum. The 13C NMR exhibited 23 signals, including one carbonyl carbon at δ 170.1. Protons along with their respective carbons were assigned by HMQC data. On the basis of HMQC and HMBC experiments the structure of aglycon was determined as benzyl 2, 6-dihydroxybenzoate which was previously reported as verimol K.11 Accordingly, enzymatic hydrolysis with β-glucosidase conferred verimol K.12 In addition, the presence of two sugar moieties was also evident from the 1H and 13C NMR data. The anomeric proton resonated at δ 5.08 (d, J= 7.2 Hz) with its corresponding carbon at δ 100.6. Additional proton signals at δ 3.56 (m, 2H), overlapping protons in the δ 3.45–3.33 ppm range, and one methylene signal at δ 3.82 and 3.66, along with five carbon signals at δ 78.2, 78.0, 71.2, 79.9, and 62.5 implied that one of the sugars was a glucose. The orientation of anomeric proton for glucose (δ 5.08, d, J=7.2 Hz) was deduced as β.13 The position of attachment of the disaccharide moiety was established by HMBC correlation between H-1’’ (δ 5.08, d, J= 7.2 Hz) and C-3 (δ 157.9). The remaining five 13C NMR signals at δ 111.2, 78.1, 80.4, 75.2, and 65.7 could be assigned to the carbons of another sugar, apiose14 from the HMBC and COSY correlation (Figure 3). The orientation of anomeric proton (δ 5.37, d, J=2.0 Hz) for apiose was deduced as β, from its smaller coupling constant (J=2.0 Hz)15 compared to that for α (J=4.5 Hz).13 The apiofuranosyl terminal sugar was shown to be linked to glucose through a (1’’’→2’’) bond on the basis of HMBC correlation observed from H-1’’’ proton of apiose to the C-2’ of glucose at δ 78.2 together with the comparison of the 13C NMR data of 3 with that of benzyl 2-O-β-D-glucopyranosyl-2, 6-dihydroxybenzoate12 which revealed that C-2’’ signal was shifted to downfield by 3.0 ppm, whereas C-1’’ appeared upfield by 2.5 ppm in 3. Identification of sugars in 3 was confirmed as glucose and apiose by comparing the Rf values of the authentic sugars on TLC after enzymatic hydrolysis with β-glucosidase followed by acid hydrolysis. As β-glucosidase selectively catalyzes the hydrolysis of β-D-glucosides, the D-configuration was assigned for glucose. Thus the structure of 3 was determined as benzyl 2-O-β-apiofuranosyl (1→2)-β-D-glucopyranosyl-2, 6-dihydroxy-benzoate.
The cell growth inhibitory activity of the isolated compounds (1 - 3) was examined by a fluorometric
microculture cytotoxicity assay (FMCA). As shown in Table 3, 1 exhibited inhibitory activity against human cervical carcinoma (HeLa) cells, and human colon carcinoma (SW480) cells with IC50 values of 6.5 and 26.3 µM, respectively. The cytotoxic potential of 1 was almost similar to mitomycin C16-17 in HeLa cells (IC50: 5.1 µM), and little bit less in SW480 cells. Compound 2 and 3 did not show cell growth inhibitory activity against the aforementioned cells.
The isolated compounds (1–3) were also evaluated for their activity in overcoming TRAIL-resistance in AGS cells. Recently, this cell line has been widely used as a model system for evaluating cancer cell apoptosis,18-19 and is reported to be refractory to apoptosis induction by TRAIL.20 To assess the effects of 1-3, TRAIL, or their combined treatment on cell viability, AGS cells were treated with the indicated agents and subjected to FMCA. As shown in Figure 4, treatment with 100 ng/mL TRAIL for 24 h resulted in only a slight decrease in cell viability (89 ± 3%). Luteolin,21 used as a positive control, produced about 50% more inhibition along with TRAIL than the agent alone. Compound 1 at 5, 10, and 20 µM reduced cell viability to 75 ± 6.0%, 48 ± 3.0%, and 35 ± 2.5% of control levels, whereas, combined treatment along with 100 ng/mL TRAIL further reduced the cell viability to 43 ± 6.0%, 11 ± 2.0%, and 4 ± 0.2% of control levels (p < 0.01), respectively, which was 32, 37, and 31% more than the agent alone. These results suggested a possible synergism between 1 and TRAIL. From the results it was evident that, 1 abrogated TRAIL-resistance of about 32% at 5 µM without any remarkable cytotoxicity. Compounds 2 and 3, however, did not produce any synergistic activity against AGS cell lines (data not shown).
Resistance of cancer cells toward TRAIL may occur at different points in the TRAIL-induced apoptotic pathways. Understanding the mechanisms of such resistance and developing strategies to overcome it are important for the successful use of TRAIL in cancer therapy.22-23 Combined treatment of TRAIL and chemotherapeutic agents including some natural products can overcome such resistance, and sensitize TRAIL-resistant cells to enhance the therapeutic potential of TRAIL against the cancer cells. Therefore, a natural product producing synergistic activity with TRAIL would be a new tool for cancer therapy.24
It has been reported that some cardiac glycosides are proved to be potent cytotoxic agents against many cancer cell lines.25 For example, 7,8-dehydrocerberin, isolated from Cerbera manghas, showed potent cytotoxic activity against KB (oral human epidermoid carcinoma), BC (human breast cancer), and NCI-H187 (human small cells lung cancer) cell lines.26 Some cardiac glycosides, for example, digoxin, digitoxin, oleandrin, lanatoside C, oubain, and bufalin are reported to sensitize TRAIL-resistant Calu1 (lung cancer) cells to Apo2L/TRAIL-induced apoptosis.27
Although, compound 2 was structurally similar to 1, except for the absence of an acetyl moiety at C-2’ of 1. But it was devoid of cytotoxicity and TRAIL-resistance-overcoming activity. Thus, the presence of an acetyl group at C-2’ in compound 1, may have contributed to its potent cytotoxic activity against HeLa and SW480 cells, and TRAIL-resistance overcoming activity in AGS cells.
EXPERIMENTAL
General Experimental Procedures. UV spectra were obtained on a Shimadzu UV mini-1240 spectrometer. Optical rotations were measured with a JASCO P-1020 polarimeter. IR spectra were measured on ATR in a JASCO FT-IR 230 spectrophotometer. The NMR spectra were recorded on JEOL A 500 and ECP 600 spectrometers with deuterated solvents, the chemical shift of which was used as an internal standard. FABMS was measured on a Jeol JMS-AX500 and HR-FABMS using a Jeol HX-110A spectrometer.
Plant Material. Aerial parts of V. solanaceae without flower was collected from Satkhira, Bangladesh in June, 2005 after flowering and taxonomically validated by the experts at Bangladesh National Herbarium (accession number: 31287), where a voucher specimen was also deposited for future reference. The air-dried plants were subjected to grinding before extraction.
Chemicals. Luteolin (purity 99%) and mitomycin C (purity 85%) were purchased from Wako Pure Chemical Industries Ltd., Japan.
Extraction and Isolation. The dried and ground plant parts of V. solanaceae (1.1 kg) was extracted with MeOH (20 L) for 7 days at room temperature followed by evaporation and vacuum desiccation to get the crude extract (70 g). The extract was then chromatographed over Diaion HP-20 (4.5 × 35 cm; particle size 250-850 µm) to exclude the chlorophyll content. The Chlorophyll free fraction (35 g) was then suspended in 10% aq. MeOH (400 mL) and partitioned between n-hexane, EtOAc, and n-BuOH (200 mL × 4). EtOAc extract (2.64 g) was subjected to Silica gel PSQ100B Column chromatography (4.2 × 30 cm, 300 g) using CHCl3-MeOH as solvent system with increasing polarity to afford seven fractions, A-G. Fraction B (450 mg) was chromatographed over silica gel PSQ-100B (4.2 × 30 cm, 80 g) using hexane-acetone (9:1 - 0:1) to afford five fractions, B1- B5. Fraction B2 was determined as β-sitosterol (15 mg). Compound 1 (4.5 mg) was isolated from fraction B4 (150 mg) after performing ODS HPLC [Senshu Pak ODS-5251-S, 2.0 × 25 cm; MeCN:H2O (2:1); flow rate: 5 mL/min; UV254 detection; tR 20 min). Fraction C (800 mg) was subjected to ODS flash column chromatography (1.0 × 40 cm, particle size: 100-200 mesh; 12 g) using MeOH:H2O (2:1) as eluent to get four fractions, C1-C4. Compound 2 (2.8 mg) was isolated from fraction C2 (300 mg) after chromatographed over Sephadex LH20 (1.0 × 40 cm) with MeOH followed by Silica gel PSQ-100B column chromatography (1.0 × 40 cm; 5 g) using hexane-acetone (1:1 - 2:3) as eluent. Fraction E (400 mg) was subjected to Silica gel PSQ-100B column chromatography (3.0 × 50 cm; 20 g) using CHCl3:MeOH (13:1 - 0:1) to afford fractions E1-E3. Fraction E2 (40 mg) underwent ODS flash column chromatography (2.5 × 40 cm; particle size: 100-200 mesh) with MeOH:H2O (1:2) to yield compound 3 (2.6 mg).
Hydrolysis of 3. Compound 3 (1.5 mg), was treated with β-glucosidase (7.0 units/mg, Sigma Chemical Co., USA, 2.0 mg), capable to catalyze the hydrolysis of β-D-glucosides, in an AcOH–AcONa buffer solution (0.1 M, pH 5.0, 2.5 mL). The mixture was stirred at 37 °C for 23 h, extracted with an equal amount of EtOAc (×3), then the EtOAc layer was evaporated under reduced pressure. The residue was dried to give the aglycone, verimol K (3a, 0.6 mg) which was previously identified by Sy et al.11 Aqueous layer (0.9 mg) containing sugar mixture (3b) was subjected to hydrolysis using 5% aqueous H2SO4 at 95 °C for 90 min. The mixture was then neutralized by Amberlite IRA96SB AG, and was dried by rotary evaporator at reduced pressure. Then the residue was examined by Silica gel TLC (Silica gel 60 F254, MERCK) using the eluent EtOAc:MeOH (7:1) to identify glucose and apiose by comparing with authentic specimens. D-Apiose was obtained from its 1,2; 2,3-di-O-isopropylidene derivative (Funakoshi Co. Ltd., Japan) after acid hydrolysis28 whereas D-glucose was purchased from Wako Pure Chemical Industries Ltd., Japan.
Vallarisoside (1): Colorless solid; [α] +24 (c 0.43, MeOH); UV (MeOH) λmax (log ε) 270 (5.0) nm; IR vmax (ATR) 3420 (br), 2930, 1740, 1375, 1238, and 1050 cm-1. 1H- and 13C NMR spectroscopic data, see Table 1; FABMS (NBA) m/z 597 [M+Na]+; HR-FABMS (NBA) m/z 597.3008 for C32H46O9Na ( ∆ −3.2 mmu).
3β-O-(α-acofriosyl)-16-anhydrogitoxigenin (2): Colorless solid; [α] +6.7 (c 0.03, CHCl3); FABMS (NBA) m/z 571 [M+K]+; UV (MeOH) λmax (log ε) 269 (5) nm; For 1H and 13C NMR spectroscopic data, see Table 1.
Benzyl 2-O-β-apiofuranosyl-(1→2)-β-D-glucopyranosyl-2,6-dihydroxybenzoate (3): Colorless amorphous solid; [α] −106 (c 0.13, MeOH); UV (MeOH) λmax (log ε) 280 (4.0) nm; IR vmax (ATR) 3370 (br), 2930, 1715, 1610, 1460, and 1070 cm-1. 1H- and 13C NMR spectroscopic data, see Table 2; FABMS m/z 561 [M+Na]+ and 577 [M+K]+; HR-FABMS m/z 561.1593 for C25H30O13Na ( ∆ +0.9 mmu).
Cell growth inhibitory activity. Cell growth inhibitory activity of the isolates was determined using a previously described method.29 Briefly, HeLa and SW480 cells were seeded in a 96-well culture plate (6 × 103 cells per well) in 200 µL of IMDM containing 10% FBS and DMEM containing 5% FBS, respectively. Cells were incubated at 37 °C in a 5% CO2 incubator for 24 h. The seeded cells were then treated with different concentrations of each isolated compound for 24 h at 37 °C. After the medium containing the samples was removed, cell growth inhibitory activity was determined by the FMCA method using a fluorescence plate reader.30 The ratio of the living cells was determined as the fluorescence in sample wells expressed as a percentage of that in the control wells, and inhibitory activity was indicated as IC50 values.
TRAIL-resistance-overcoming activity. TRAIL-resistance-overcoming activity of the isolated compounds was assessed by comparison of cell growth inhibitory activity in the presence and absence of TRAIL against TRAIL-resistant human gastric adenocarcinoma (AGS) cell lines.31 Briefly, AGS cells were seeded in a 96-well culture plate (6 × 103 cells per well) in 200 µL of RPMI medium containing 10% FBS. Cells were incubated at 37 °C in a 5% CO2 incubator for 24 h. Then the test samples with or without TRAIL (100 ng/mL) at different doses were added to each well. After 24 h incubation, the cells were washed with PBS, and 200 µL of PBS containing fluorescein diacetate (10 µg/mL) was added to each well. The plates were then incubated at 37 °C for 1 h, and fluorescence was measured in a 96-well scanning spectrofluorometer at 538 nm, following excitation at 485 nm.
ACKNOWLEDGEMENTS
This work was supported by Grants-in-Aid for Scientific Research from the Japan Society for the Promotion of Science (JSPS), and by Grants-in-Aid from the Takeda Science Foundation, and from the Mitsubishi Chemical Corporation Fund.
References
1. N. Ahmed, The Wild Flower of Bangladesh, 1st edition. The University Press Ltd., Dhaka, 1997, p. 43.
2. R. L. Khosa, A. K. Wahi, and A. K. Mukherjee, J. Indian Chem. Soc., 1979, 56, 935.
3. K. W. Gopinath, P. A. Mohamed, and A. R. Kidwai, J. Indian Chem. Soc., 1963, 1, 98.
4. H. Kaufmann, W. Wehrli, and T. Reichstein, Helv. Chim. Acta, 1965, 48, 65. CrossRef
5. H. Kaufmann, Helv. Chim. Acta, 1965, 48, 83. CrossRef
6. B. S. Siddiqui, R. Sultana, S. Begum, A. Zia, and A. Suria, J. Nat. Prod., 1997, 60, 540. CrossRef
7. R. Sanduja, W. Y. R. Lo, K. L. Eular, and M. Alam, J. Nat. Prod., 1984, 47, 260. CrossRef
8. R. Kasai, M. Okihara, J. Asakawa, K. Mizutani, and O. Tanaka, Tetrahedron, 1979, 35, 1427. CrossRef
9. T. Yamauchi, F. Abe, and A. S. C. Wan, Chem. Pharm. Bull., 1987, 35, 2744.
10. K. Tori, H. Ishii, Z. W. Wolkowski, C. Chachaty, M. Sangaré, F. Piriou, and G. Lukacs, Tetrahedron Lett., 1973, 35, 1077. CrossRef
11. L.–K. Sy and G. D. Brown, J. Nat. Prod., 1998, 61, 987. CrossRef
12. B. D'Abrosca, M. DellaGreca, A. Fiorentino, P. Monaco, L. Previtera, A. M. Simonet, and A. Zarrelli, Phytochemistry, 2001, 58, 1073. CrossRef
13. Y. Lei, L.-J. Wu, H.-M. Shi, and P.- F. Tu, Helv. Chim. Acta, 2008, 91, 495. CrossRef
14. B. Ahmad, M. Hamburger, M. P. Gupta, P. N. Solis, and P. Hostettmann, Phytochemistry, 1991, 30, 3781. CrossRef
15. T. Kanchanapoom, R. Kasai, and K. Yamasaki, Phytochemistry, 2002, 59, 557. CrossRef
16. E. Mladenov, I. Tsaneva, and B. Anachkova, J. Cell. Physiol., 2006, 211, 468. CrossRef
17. I. Masahiko, H. Yufuko, T. Fuminori, S. Shiro, and K. Yoshimi, J. Interferon Res., 1987, 7, 419.
18. C.-Y. Jin, C. Park, J. Cheong, B. T. Choi, T. H. Lee, J. Lee, J.-D. Lee, W. H. Lee, G.-Y. Kim, C. H. Ryu, and Y. H. Choi, Cancer Lett., 2007, 257, 56. CrossRef
19. J.-D. Liu, S.-Y. Lin, Y.-S. Ho, P. Shian, L.-F. Hung, S.-H. Tsai, J.-K. Lin, and Y.-C. Liang, Mol. Carcinog., 2003, 37, 16. CrossRef
20. R. K. Srivastava, Neoplasia, 2001, 3, 535. CrossRef
21. M. Horinaka, T. Yoshida, T. Shiraishi, S. Nakata, M. Wakada, R. Nakanishi, H . Nishino, and T. Sakai, Biochem. Biophys. Res. Commun., 2005, 333, 833. CrossRef
22. D. R. Camidge, Clin. Lung Cancer, 2007, 8, 413. CrossRef
23. S. Baritaki, S. Huerta-Yepez, T. Sakai, D. A. Spandidos, and B. Bonavida, Mol. Cancer Ther., 2007, 6, 1387. CrossRef
24. S. Shankar and R. K. Srivastava, Drug Resist. Updat., 2004, 7, 139. CrossRef
25. J.-Z. Li, C. Qing, C.-X. Chen, X.-J. Hao, and H.-Y. Liu, Bioorg. Med. Chem. Lett., 2009, 19, 1956. CrossRef
26. S. Cheenpracha, C. Karalai, and Y. Rat-a-pa, C. Ponglimanont, and K. Chantrapromma, Chem. Pharm. Bull., 2004, 52, 1023. CrossRef
27. S. Frese, M. Frese-Schaper, A. C. Andres, D, Miescher, B. Zumkehr, and R. A. Schmid, Cancer Res., 2006, 66, 5867. CrossRef
28. D. T. Williams and J. K. N. Jones, Can. J. Chem., 1964, 42, 69. CrossRef
29. T. Ohtsuki, M. Sato, T. Koyano, T. Kowithayakorn, N. Kawahara, Y. Goda, and M. Ishibashi, Bioorg. Med. Chem., 2006, 14, 659. CrossRef
30. E. Lindhagen, P. Nygren, and R. Larsson, Nat. Protocs., 2008, 3, 1364. CrossRef
31. F. Ahmed, T. Ohtsuki, W. Aida, and M. Ishibashi, J. Nat. Prod., 2008, 71, 1963. CrossRef