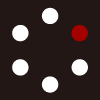
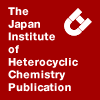
HETEROCYCLES
An International Journal for Reviews and Communications in Heterocyclic ChemistryWeb Edition ISSN: 1881-0942
Published online by The Japan Institute of Heterocyclic Chemistry
e-Journal
Full Text HTML
Received, 5th June, 2009, Accepted, 6th August, 2009, Published online, 7th August, 2009.
DOI: 10.3987/COM-09-S(S)32
■ Synthesis of 3-Aryl-1,2-dicarba-closo-dodecaboranes by Suzuki-Miyaura Coupling Reaction
Koei Aizawa, Kiminori Ohta, and Yasuyuki Endo*
Tohoku Pharmaceutical University, 4-4-1 Komatsushima, Aoba-ku, Sendai 981-8558, Japan
Abstract
In contrast to conventional syntheses of 3-aryl-o-carborane derivatives, which involve reconstruction of the o-carborane cage or Pd-catalyzed coupling reaction of 3-iodo-o-carborane with Grignard reagents, the Suzuzki-Miyaura coupling reaction of 3-iodo-o-carborane proceeds with a variety of aryl boronic acids, providing a new route to 3-aryl-o-carborane derivatives bearing easily transformable functional groups, including CH3O-, NO2-, CHO- and CN-. This approach provides easy access to a variety of compounds with a 3-aryl-o-carborane scaffold.INTRODUCTION
Because of their high boron content and excellent chemical and thermal stability, 1,2-dicarba-closo-dodecaborane (o-carborane; 1) clusters are useful in many fields, from medicinal chemistry to materials sciences.1 Especially, aryl-o-carborane skeleton was an useful building block in these fields. 3-Aryl-o-carborane derivatives (2) are used in the fields of molecular recognition and medicinal chemistry and have been synthesized in two different ways.2 One involves reconstruction of the o-carborane cage through the decomposition of 1 to nido-7,8-C2B9H12- cluster with sodium hydroxide (NaOH), followed by reaction with aryl dichloroboranes, Ar-BCl2,3 and the other is palladium (Pd)-catalyzed coupling of 3-iodo-o-carborane (3)4 with Grignard reagents (Scheme 1).5 However, available substituents on the aromatic ring in these reactions are strictly limited because of the high reactivities of holoboranes and Grignard reagents. In this paper, we report the synthesis of 2 with various sensitive functional groups, including methoxy (CH3O), nitro (NO2), formyl (CHO) and cyano (CN) groups, by means of the Suzuki-Miyaura coupling reaction of 3 with the corresponding aryl boronic acids (Scheme 1). The above functional groups are easily transformable into a variety of substituents. Thus, this new route to 2 is expected to be useful in the development of o-carborane chemistry.
RESULTS AND DISCUSSION
Initially, the coupling reaction of 3-iodo-o-carborane 3 with phenylboronic acid was investigated under the standard condition of Suzuki-Miyaura coupling: a solution of 3 (0.37 mmol), phenylboronic acid (PhB(OH)2, 1.5 eq), tetrakis(triphenylphosphine)palladium (0) (Pd(PPh3)4, 10 mol%) and potassium carbonate (K2CO3, 3.0 eq) in 4 mL of toluene was heated at 90oC for 7 h.6 The reaction proceeded smoothly to afford 3-phenyl-o-carborane 2a in 86% yield (Scheme 2).
Table 1 summarizes the results of the reaction of several arylboronic acids with synthetically useful functional groups with 3 under the same reaction conditions. 4-Methoxyphenylboronic acid readily reacted with 3 to afford 3-(4-methoxyphenyl)-o-carborane 2b in 85% yield (entry 1). However, the coupling reactions of 3 with other phenylboronic acids bearing an electron-withdrawing group hardly proceeded affording the desired compounds in yields of only a few percent, even if the reaction time was increased (entries 2-5). Thus, the electronic properties of the aromatic ring of the boronic acids affect the product yields in this reaction.
The effects of the solvent and base were investigated in the reaction of 3 with 3-formylphenylboronic acid (Table 2). When N,N-dimethylformamide (DMF) was used as a solvent, the starting material 3 completely disappeared in a short time in comparison to the use of toluene or xylene as a solvent, but the yield of 3-(3-formylphenyl)-o-carborane 2c was unchanged (entries 1-3). Decomposition of 3 to nido-carborane was observed in these reactions. Because the boron atoms at the 3- and 6-position, especially the unsubstituted boron atom of o-carborane, have the lowest electron density in the cage, these boron atoms are vulnerable to nucleophilic attack by base, resulting in decomposition to the corresponding nido-carborane.7 The nucleophilic attack by base at the 6-position of 3 appeared to be greatly accelerated in DMF. To evaluate the effects of base, sodium carbonate (Na2CO3), potassium phosphate (K3PO4) and cesium fluoride (CsF) were examined in this reaction. Although the yield of 2c were little changed with any base or solvent, the required reaction time was altered. Neither the product nor the recovery yield was influenced by K3PO4 (entry 4). The use of a polar solvent, such as a mixture of toluene/ethanol (EtOH), also caused decomposition of 3, even if a mild base, Na2CO3, was used (entry 5). When CsF was used as a base, the recovery yield of 3 was greatly decreased, even when toluene was used as a solvent (entry 6). It seems likely that decomposition of the o-carborane cage arose through nucleophilic attack of F- anion, which is known to have high nucleophilicity.8
As the desired product 2c was prepared quantitatively or less with respect to the catalytic amount of Pd-catalyst, it appeared that the catalytic cycle of Pd was inactive in the coupling reaction (Table 1 and 2). In addition, insoluble Pd black was precipitated during the reaction with the electron-deficient boronic acids, suggesting that the intermediate resulting from oxidative addition of Pd(0) to 3 is relatively unstable and that the rate of transmetalation was greatly affected by the electronic properties of the boronic acids. It is conceivable that transmetalation is the rate-determining step in the reaction. Therefore, we investigated the effects of bidentate ligands and Pd species on the coupling reaction. The results are summarized in Table 3. However, neither bidentate ligands such as 1,4-bis(diphenylphsphino)butane (dppb), 1,3-bis(diphenylphosphino)propane (dppp), and rac-bis(diphenylphosphino)-1,1’-binaphthalene (rac-BINAP) nor Pd species affected the yield of 2c (entries 1-3 and 4). With the aims of increasing the basicity of K2CO3 and the solubility of the Pd(II) catalyst, a mixed solvent, toluene:MeOH 10:1, was used for this reaction (entry 5). Surprisingly, the starting material 3 totally disappeared and 2c was produced in 52% yield in the reaction time (20 h).
The effects of a mixed solvent, toluene:MeOH were examined on the coupling reaction of 3 with 3-cyanophenylboronic acid in stead of 3-formylphenylboronic acid (Table 4). When a mixed solvent, toluene:MeOH 10:0.5, was used for this reaction, there was a remarkable decrease in the reaction rate (entry 1 vs 2). On the other hand, a mixed solvent, toluene:MeOH 10:2, increased in the degradation reaction rate of 3 (entry 2 vs 3). Thus, we found that a mixed solvent, toluene:MeOH 10:1 is the best for this reaction (entry 2). Using of i-PrOH instead of MeOH under the conditions, the reaction did not proceed and give the desired product, 2f (data not shown). It is not clear why MeOH was a good additive for this reaction, but one possibility is that the reducing character of MeOH assists the catalytic turnover of Pd. Since the use of a polar solvent such as MeOH leads to decomposition of the o-carborane cage by the base, the added amount of MeOH must be carefully controlled in this coupling reaction.
Finally, the coupling reaction of 3 with arylboronic acid with electron-withdrawing groups was refined under the following conditions. A solution of compound 3 (0.37 mmol), arylboronic acid (1.5 eq), Pd(PPh3)4 (10 mmol%) and K2CO3 (3.0 eq) in 4 mL of mixed solvent of 10:1 toluene:methanol was heated at 90 oC. The starting material 3 readily reacted with 3-formylphenylboronic acid to afford 1c in 66% yield. Arylboronic acid with 4-NO2, 3-NO2, and 3-CN groups also reacted with 3 to afford the corresponding compounds 1d, 1e and 1f in 20, 32 and 34% yields, respectively. Since the reaction of 3 with these boronic acids under the conditions used was always accompanied with decomposition of the o-carborane cage, the coupling products were obtained in only moderate yields.
In conclusion, we found that the Suzuki-Miyaura coupling reaction is a promising new route to 3-aryl-o-carborane derivatives 2 from 3-iodo-o-carborane 3. Phenylboronic acid and 4-methoxyphenylboronic acid reacted readily with 3 to afford the corresponding 2a and 2b in high yields. When arylboronic acids with an electron-withdrawing group were used for the coupling reaction, a mixed solvent of toluene:MeOH 10:1 accelerated the reaction to afford the corresponding compounds 2c-2f in moderate yields. It is noteworthy that the derivatives 2 with functional groups such as CH3O-, NO2-, CHO- and CN- were readily obtainable from 3. We believe these findings represent a breakthrough in the development of o-carborane chemistry.
EXPERIMENTAL
General Considerations. 1H NMR (400 MHz), 13C NMR (100 MHz) and 10B NMR (127 MHz) spectra were recorded with JEOL JNM-LA-400 spectrometer. Chemical shifts for 1H NMR spectra were referenced to tetramethylsilane (0.0 ppm) as an internal standard. Chemical shifts for 13C NMR spectra were referenced to residual 13C present in deuterated solvents. Chemical shift values for 11B spectra were referenced relative to external BF3-OEt2 (0.0 ppm, with negative values upfield). The chemical shifts are reported in parts per million (δ scale), and all coupling constants (J) values are given in hertz (Hz). The splitting patterns are designated as follows: s (singlet), d (doublet), t (triplet), m (multiplet), and br (broad). Melting points were determined with a Yanaco micro melting point apparatus without correction. Mass and IR spectra were recorded on JEOL JMS-DX-303 and Perkin-Elmer SpectrumOne FT-IR spectrometers, respectively. Elemental analyses were performed on a Perkin-Elmer 2400 CHN apparatus. All reactions were carried out under an argon atmosphere.
Materials. Unless otherwise noted, the reagents and solvents were purchased from Aldrich Chemical Co., Kanto Chemicals, Tokyo Kasei, or Wako Chemicals, Inc. and were used as received. o-Carborane was purchased from Katchem s.r.o. (Prague, Czech Republic). 3-Iodo-o-carborane 3 was synthesized according to the literature.3
A typical procedure of Suzuki-Miyaura coupling reaction. A solution of 3-iodo-o-carborane 3 (100 mg, 0.37 mmol), 3-formylphenylboronic acid (84 mg, 0.56 mmol), anhydrous K2CO3 (153 mg, 1.11 mmol) in toluene:MeOH 10:1 was degassed, and Pd(PPh3)4 (43 mg, 0.037 mmol) was added to the solution. The mixture was degassed again, heated at 90 oC for 8 h under an Ar atmosphere, then poured into water and extracted with AcOEt. The organic phase was washed with brine, dried over Na2SO4, and concentrated. The residue was purified by column chromatography on silica gel with n-hxane:CH2Cl2 5:2 to afford 60 mg (66%) of 3-(3-formylphenyl)-o-caborane (2c) as a colorless solid. Colorless leaflets (CH2Cl2-n-hexane), mp 102-104 oC, 1H NMR (CDCl3) δ 1.0-3.5 (brm, 9H), 3.77 (s, 2H), 7.57 (t, J = 7.7 Hz, 1H), 7.92 (dd, J = 1.5 Hz, 7.7 Hz, 2H), 8.05 (s, 1H), 10.04 (s, 1H); 13C NMR (CDCl3) δ 56.5, 129.1, 131.4, 133.2, 136.0, 139.3, 192.1; 11B NMR (CDCl3) δ -13.4 (3B), -13.0 (2B), -11.8 (1B), -8.7 (1B), -6.1 (1B), -2.2 (2B); IR (KBr) cm-1 1184, 1702, 2596, 2633, 3049, 3067; MS (EI) m/z 248 (M+, 100%); HRMS Calcd. for C9H16B10O: 248.2209, Found: 248.2213.
3-Phenyl-1,2-dicarba-closo-dodecaborane (2a). Colorless powder (CH2Cl2-n-hexane), mp 109-110 oC, 1H NMR (CDCl3) δ 1.0-3.5 (brm, 9H), 3.71 (s, 2H), 7.30-7.50 (m, 6H), 7.60 (d, J = 6.8 Hz, 2H); 13C NMR (CDCl3) δ 56.6, 128.3, 129.7, 133.1; 11B NMR (CDCl3) δ -13.0 (3B), -12.3 (2B), -11.6 (1B), -7.9 (1B), -4.4 (1B), -1.8 (2B); IR (KBr) cm-1 1435, 2563, 2572, 2609, 3053, 3066; MS (EI) m/z 220 (M+, 100%).
3-(4-Methoxyphenyl)-1,2-dicarba-closo-dodecaborane (2b). Colorless powder (CH2Cl2-n-hexane), mp 95-96 oC, 1H NMR (CDCl3) δ 1.0-3.5 (brm, 9H), 3.67 (s, 2H), 3.83 (s, 3H), 6.90 (d, J = 8.8 Hz, 2H), 7.52 (d, J = 8.4 Hz, 2H); 13C NMR (CDCl3) δ 55.2, 56.7, 113.9, 134.5, 161.0; 11B NMR (CDCl3) δ -13.1 (3B), -12.3 (3B), -7.9 (1B), -4.0 (1B), -1.9 (2B); IR (KBr) cm-1 1241, 1287, 1514, 1605, 2558, 2575, 2599, 2609, 2634, 3061; MS (EI) m/z 250 (M+, 100%); Anal. Calcd for C9H18B10O: C, 43.18; H, 7.25. Found: C, 43.35; H, 7.30.
3-(4-Nitrophenyl)-1,2-dicarba-closo-dodecaborane (2d). Pale yellow plates (CH2Cl2-n-hexane), mp 200-203 oC, 1H NMR (CDCl3) δ 1.0-3.5 (brm, 9H), 3.76 (s, 2H), 7.78 (d, J = 8.6 Hz, 2H), 8.21 (d, J = 8.6 Hz, 2H); 13C NMR (CDCl3) δ 56.5, 123.0, 134.1, 148.9; 11B NMR (CDCl3) δ -12.3 (5B), -10.7 (1B), -7.8 (1B), -5.9 (1B), -1.3 (2B); IR (KBr) cm-1 1351, 1515, 2557, 2591, 2607, 3054, 3068; MS (EI) m/z 265 (M+, 100%); Anal. Calcd for C8H15B10NO2: C, 36.21; H, 5.70; N, 5.28. Found: C, 36.17; H, 5.60; N, 5.21.
3-(3-Nitrophenyl)-1,2-dicarba-closo-dodecaborane (2e). Pale yellow needles (CH2Cl2-n-hexane), mp 133-134 oC, 1H NMR (CDCl3) δ 1.0-3.5 (brm, 9H), 3.78 (s, 2H), 7.58 (t, J = 7.8 Hz, 1H), 7.99 (d, J = 7.4 Hz, 1H), 8.28 (d, J = 8.2 Hz, 1H), 8.38 (s, 1H); 13C NMR (CDCl3) δ 56.5, 124.6, 127.2, 129.5, 139.5, 147.9; 11B NMR (CDCl3) δ -12.4 (5B), -10.8 (1B), -7.9 (1B), -5.8 (1B), -1.3 (2B); IR (KBr) cm-1 1347, 1519, 1530, 2583, 3066; MS (EI) m/z 265 (M+, 100%); Anal. Calcd for C8H15B10NO2: C, 36.21; H, 5.70; N, 5.28. Found: C, 35.93; H, 5.58; N, 5.16.
3-(3-Cyanophenyl)-1,2-dicarba-closo-dodecaborane (2f). Colorless needles (CH2Cl2-n-hexane), mp 155-156 oC, 1H NMR (CDCl3) δ 1.0-3.5 (brm, 9H), 3.73 (s, 2H), 7.50 (t, J = 7.7 Hz, 1H), 7.71 (d, J = 7.7 Hz, 1H), 7.84 (d, J = 7.8 Hz, 1H), 7.87 (s, 1H); 13C NMR (CDCl3) δ 56.5, 112.6, 118.5, 129.0, 133.1, 136.4, 137.4; 11B NMR (CDCl3) δ -12.5 (5B), -10.9 (1B), -7.9 (1B), -5.7 (1B), -1.3 (2B); IR (KBr) cm-1 2220, 2587, 3057; MS (EI) m/z 245 (M+, 100%); Anal. Calcd for C9H15B10N: C, 44.06; H, 6.16; N, 5.71. Found: C, 44.18; H, 6.24; N, 5.68.
ACKNOWLEDGEMENTS
This work was supported by a Grant-in-Aid for Scientific Research (B) (No. 20390035) from the Ministry of Education, Culture, Sports, Science, and Technology of Japan.
References
1. A. F. Armstrong and J. F. Valliant, Dalton Trans., 2007, 4240; CrossRef P. C. Andrews, M. J. Hardie, and C. L. Raston, Coord. Chem. Rev., 1999, 189, 169; CrossRef M. F. Hawthorne and Z. Zheng, Acc. Chem. Res., 1997, 30, 267. CrossRef
2. B. M. Ramachandran, C. B. Knobler, and M. F. Hawthorne, J. Mol. Struct., 2006, 785, 167; CrossRef T. Ogawa, K. Ohta, T. Yoshimi, H. Yamazaki, T. Suzuki, S. Ohta, and Y. Endo, Bioorg. Med. Chem. Lett., 2006, 16, 3943. CrossRef
3. M. F. Hawthorne and P. A. Wegner, J. Am. Chem. Soc., 1968, 90, 896. CrossRef
4. J. Li, C. F. Logan, and M. Jones, Jr., Inorg. Chem., 1991, 30, 4866; CrossRef G. Barberà, C. Viñas, F. Teixidor, G. M. Rosair, and A. J. Welch, J. Chem. Soc., Dalton Trans., 2002, 3647. CrossRef
5. Z. Zheng, W. Jiang, A. A. Zinn, C. B. Knobler, and M. F. Hawthorne, Inorg. Chem., 1995, 34, 2095. CrossRef
6. A. Suzuki, J. Organomet. Chem., 1999, 576, 147. CrossRef
7. R. A. Weisboeck and M. F. Hawthorne, J. Am. Chem. Soc., 1964, 86, 1642. CrossRef
8. M. L. Bender and W. A. Glasson, J. Am. Chem. Soc., 1959, 81, 1590; CrossRef R. F. Hudson and M. J. Green, J. Chem. Soc., 1962, 1055; CrossRef W. P. Jencks and M. J. Gilchrist, J. Am. Chem. Soc., 1968, 90, 2622. CrossRef