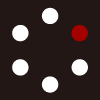
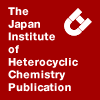
HETEROCYCLES
An International Journal for Reviews and Communications in Heterocyclic ChemistryWeb Edition ISSN: 1881-0942
Published online by The Japan Institute of Heterocyclic Chemistry
e-Journal
Full Text HTML
Received, 3rd April, 2009, Accepted, 14th May, 2009, Published online, 14th May, 2009.
DOI: 10.3987/COM-09-S(S)21
■ Hetero Diels-Alder Reaction of β-Trifluoroacetylated Vinyl Ethers with Vinyl Ethers to Access Fluorine-Containing Dihydropyran Derivatives — A Molecular Orbital Calculation Study
Norio Ota, Yasuhiro Kamitori,* Dai Shibata, and Etsuji Okada*
Department of Chemical Science and Engineering, Graduate School of Engineering, Kobe University, 1-1 Rokkodai-cho, Nada-ku, Kobe 657-8501, Japan
Abstract
The reactivity differences between β-trifluoroacetylvinyl ethers (1), β,β-bis(trifluoroacetyl)vinyl ethers (2), β-acetylvinyl ethers (3), and β,β-diacetylvinyl ethers (4) in the hetero Diels-Alder reaction with vinyl ethers giving dihydropyrans (5, 6, 7, and 8, respectively) are discussed on the basis of molecular orbital calculations. Frontier orbital interactions and activation energies of these reactions were estimated using DFT calculations.INTRODUCTION
In recent years, research efforts have focused on developing new methodologies for the syntheses of fluorine-containing heterocycles. These compounds are widely recognized as important organic materials, showing specific functions as well as biological activities that are of interest.1-4 Previously, we reported efficient and convenient synthetic methods to obtain fluorine-containing dihydropyrans (5, 6) from β-trifluoroacetylvinyl ethers (1)5 and β,β-bis(trifluoroacetyl)vinyl ethers (2).6 We found that the hetero Diels-Alder reaction of 1 with vinyl ethers readily occurred at 80 °C and that of 2 with vinyl ethers proceeded smoothly below 40 °C.5,6 On the other hand, the hetero Diels-Alder reaction of β-acetylvinyl ethers (3) bearing no fluorine atoms with vinyl ethers required high reaction temperatures (190-210 °C) to access the corresponding dihydropyrans (7).7 Even in the case of β,β-diacetylvinyl ethers (4) with two electron-withdrawing acetyl groups, elevation of the reaction temperature (over 100 °C) was necessary for the corresponding cycloaddition to afford dihydropyrans (8).8
In general, Lewis acid catalysis is necessary for inverse-electron-demand Diels-Alder reaction of heterodienes with electron-rich alkenes under mild conditions.9 Therefore, it is worth noting that the reaction of 2 with vinyl ethers proceeded, even at ambient temperatures, in the absence of a catalyst.6 We were thus very interested in the effect of trifluoromethyl and trifluoroacetyl groups on the present hetero Diels-Alder reaction.
The hetero Diels-Alder reaction to afford 5 and 6 is a potential route to prepare carbohydrates and related compounds bearing a CF3 component. Furthermore, dihydropyrans (5, 6) are potentially useful intermediates to access a variety of fluorine-containing organic compounds including heterocycles. We found the specific ring-opening reactions of 5 and 6 with nucleophiles, such as thiols,10,11 amines,12 and aromatic compounds13 afford novel fluorine-containing dienone derivatives, which are expected to be versatile building blocks for various CF3-containing heterocycles. Despite its importance for the synthesis of various dihydropyran derivatives including 5 and 6, there have been no reports of molecular orbital calculations elucidating the hetero Diels-Alder reaction of electron-deficient heterodienes (1-4) with electron-rich alkenes, such as vinyl ethers.
Here, we present hetero Diels-Alder reaction of these systems along with the results of molecular orbital calculations. The reactivity differences between compounds 1-4 in the hetero Diels-Alder reaction with electron-rich alkenes are elucidated on the basis of DFT calculations.
RESULTS AND DISCUSSION
Previously, we reported that the hetero Diels-Alder reaction of β-trifluoroacetylvinyl ethers (1b,d) with vinyl ethers (9b-d) readily occurred at 80 °C to give the corresponding 3,4-dihydro-2H-pyrans (5bb,bc,db,dd) in excellent yields (Scheme 1, Table 2, Entries 2, 3, 5 and 6).5 In contrast, the corresponding reaction of 4-methoxy-3-buten-2-one (3a) with isobutyl vinyl ether (9c), affording 2-isobutoxy-6-methyl-3,4-dihydro-2H-pyran (7ac), proceeded much slower, even at 190-210 °C, and the conversion did not exceed 45% (Scheme 1, Table 2, Entry 8).7 In order to understand the reactivity difference between 1 and 3 for the hetero Diels-Alder reaction with vinyl ethers (9), we carried out 6-31G* level DFT calculations (RB3LYP/6-31G*) on the reactions shown in Scheme 1.
The optimized structures and their energy values were computed for β-trifluoroacetylvinyl ethers (1a,b,d), 4-methoxy-3-buten-2-one (3a), and vinyl ethers (9a-d). The values of total energy and frontier orbital energy of these substrate molecules are summarized in Table 1.
The energy level gaps (ΔEf1) between the HOMO of 9a-d and the LUMO of 1a,b,d and 3a, and those (ΔEf2) between the HOMO of 1a,b,d and 3a and the LUMO of 9a-d were calculated for all hetero Diels-Alder reactions depicted in Scheme 1 (Table 2). In all the cases, the values of ΔEf1 were much smaller than those of ΔEf2, clearly indicating that the cycloaddition reactions in Scheme 1 are tentative inverse electron-demand Diels-Alder reaction of electron-deficient heterodienes (1 and 3) with electron-rich alkenes (9).
The calculated ΔEf1 for the hetero Diels-Alder reactions of β-trifluoroacetylvinyl ethers (1a,b,d) with vinyl ethers (9a-d) were 3.896 - 4.123 eV (Entries 1-6) and the difference between each ΔEf1 did not exceed 0.23 eV. On the other hand, ΔEf1 for the reactions of 4-methoxy-3-buten-2-one (3a) with vinyl ethers (9a,c) were 4.970 - 4.991 eV (Entries 7, 8). The ΔEf1 values for the reaction of 1a, 1b, and 1d were ca. 1 eV smaller than those for the reaction of 3a.
The smaller ΔEf1 causes a stronger frontier orbital interaction between the heterodienes and alkenes, thus promoting the hetero Diels-Alder reaction more effectively. The results are compatible with the experimental results showing that cycloaddition of β-trifluoroacetylvinyl ethers (1b,d) with vinyl ethers (9b-d) occurs more readily than that of β-acetylvinyl ether (3a) with isobutyl vinyl ether (9c).5,7 The strong electron-withdrawing fluorine substituents effectively lower the LUMO level of 1 (Table 1), thereby decreasing ΔEf1 (Table 2) and making the hetero Diels-Alder reaction of 1 with 9 more facile than that of non-fluorinated 3 with 9.
The hetero Diels-Alder reaction of β,β-bis(trifluoroacetyl)vinyl ether (2) with vinyl ether (9) occurs more readily than that of β-trifluoroacetylvinyl ether (1) with 9.6 The reaction of alkyl β,β-bis(trifluoroacetyl)vinyl ethers (2b,c) with 9b and 9d and that of aryl β,β-bis(trifluoroacetyl)vinyl ether (2d) with 9d proceeded successfully at ambient temperature and 40 °C, respectively, to afford the corresponding dihydropyrans (6bb,cb,bd,dd) in moderate to high yields (Scheme 2, Table 4).6 Furthermore, cycloaddition of 2b with 1,1-diphenoxyethylene (10d) and that of 2b and 2c with ethyl vinyl sulfide (11b) also proceeded successfully under similar conditions to afford the corresponding 12bd, 13bb, and 13cb, respectively (Figure 1, Table 4).6 In contrast, the hetero Diels-Alder reaction of β,β-diacetylvinyl ethers (4a,b) with ethyl vinyl ethers (9b) in Scheme 2 required a much higher reaction temperature (140 °C).8
The energy values and the frontier orbital energy levels of the most stable structures of β,β-bis(trifluoroacetyl)vinyl ethers (2a-d) and β,β-diacetylvinyl ethers (4a,b) are summarized in Table 3. As expected, the LUMO energies of 2a-d, 4a, and 4b (Table 3) were lower than those of 1a-d and 3a (Table 1). These results clearly indicate that the hetero Diels-Alder reaction of 2a-d, 4a, and 4b with electron-rich alkenes, such as 9a-d, is also of the inverse electron-demand type reaction.
We calculated the energy level gaps (ΔEf1) between the HOMO of 9a, 9b, and 9d and the LUMO of 2a-d, 4a, and 4b for all cases of cycloaddition in Scheme 2 (Table 4). Moreover, for the cycloaddition of 2a and 2b with 1,1-diphenoxyethylene (10d) and vinyl sulfides (11a,b), which afforded the dihydropyrans (12ad,bd and 13aa-cb; Figure 1), we evaluated the ΔEf1 values (Table 4).
The values of ΔEf1 for the hetero Diels-Alder reaction of β,β-bis(trifluoroacetyl)vinyl ethers (2a-d) with vinyl ethers (9a-d) were in the range of 3.319 – 3.486 eV (Entries 1-6). These ΔEf1 values were ca. 0.8 eV smaller than those for the reaction of β,β-diacetylvinyl ethers (4a,b) with 9b (Entries 7, 8), and ca. 0.6 eV smaller than those for the reaction of β-trifluoroacetylvinyl ethers (1a,b,d) with 9a-d (Entries 1-6 in Table 2). These results are consistent with the experimental results, showing that the cycloaddition of β,β-diacetylvinyl ethers (4a,b) with ethyl vinyl ether (9b) requires elevated reaction temperature up to 140 °C8 and that of β-trifluoroacetylvinyl ethers (1b,d) with vinyl ethers (9b-d) occurs at 80 °C,5 whereas the corresponding cycloaddition of β,β-bis(trifluoroacetyl)vinyl ethers (2b-d) with 9b-d readily occurs below 40 °C.6
The values of ΔEf1 for the cycloaddition of 2a and 2b with 1,1-diphenoxyethylene (10d; Entries 9, 10) were slightly (ca. 0.2 eV) larger than those for the corresponding cycloaddition of 2a and 2b with phenyl vinyl ether (9d; Entries 4,5). In contrast, ΔEf1 for the cycloaddition of 2a-c with vinyl sulfides (11a,b; Entries 11-13) were slightly (ca. 0.2 eV) smaller than those for the corresponding cycloaddition of 2a-c with vinyl ether (9a,b; Entries 1-3). However, differences in the ΔEf1 smaller than 0.3 eV would show no significant effect on the reaction. These results are also in good agreement with experimental results, showing that the cycloaddition of β,β-bis(trifluoroacetyl)vinyl ether (2) with ketene acetals (10d) and vinyl sulfides (11a,b) occurs as readily as that of 2 with vinyl ethers (9).6
In order to confirm these findings on the hetero Diels-Alder reactions of heterodienes (1-4) with electron-rich alkenes (9-11), we computed transition state structures for representative cases of these reactions and estimated their energies. Two pairs of optimized transition state structures (endo-TS5bb and exo-TS5bb; endo-TS6bb and exo-TS6bb) corresponding to the endo- and exo-[4+2]cycloaddition of 1b with 9b and those of 2b with 9b are depicted in Figure 2 together with their energy values. The transition state structure, endo-TS5bb, was estimated to be 0.8 kcal/mol more stable than exo-TS5bb, and endo-TS6bb was estimated to be 2.1 kcal/mol more stable than exo-TS6bb. These findings are in good agreement with our experimental results, in which endo-[4+2]cycloaddition occurs preferentially in both cases of the reaction of 1b with 9b and 2b with 9b to give dihydropyrans (cis-5bb and cis-6bb, respectively) as the major products together with trans-5bb and trans-6bb as the minor products, respectively.5,6
Some interatomic distances in endo-TS5bb and endo-TS6bb are also indicated in Figure 2. In both cases, the C(1’)−O(4) bond was longer (0.398 Å and 0.542 Å, respectively) than the C(2’)−C(1) bond.
From the energy values of the endo type transition state structures, we estimated the activation energies (ΔE≠)14 of hetero Diels-Alder reactions of β-acylvinyl ethers (1a,b,d and 3a) and β,β-diacylvinyl ethers (2a,b and 4a,b) with electron-rich alkenes (9a-d, 10a, and 11a,b) (Table 5). The estimated ΔE≠ values of the cycloaddition of β-trifluoroacetylvinyl ethers (1a,b,d) with vinyl ethers (9a-c; Entries 1-5) were 12-14 kcal/mol, which was over 6 kcal/mol lower than that of the reactions of β-acetylvinyl ethers (3a) with 9a and 9c (Entries 6, 7). The ΔE≠ for the cycloaddition of β,β-bis(trifluoroacetyl)vinyl ethers (2a,b) with 9a and 9b (Entries 8, 9) was 7-8 kcal/mol, which was over 7 kcal/mol lower than that of the corresponding reaction of β,β-diacetylvinyl ethers (4a,b; Entries 11,12), and at least 4 kcal/mol lower than the corresponding reaction of β-trifluoroacetylvinyl ethers (1a,b,d; Entries 1-5). The ΔE≠ for the cycloaddition of 2b with phenyl vinyl ether (9d; Entry 10) was estimated to be 12.2 kcal/mol, which was ca. 5 kcal higher than that of the corresponding reaction with ethyl vinyl ether (9b; Entry 9). This relatively high activation energy corresponds to the fact that the cycloaddition of 2b with phenyl vinyl ether 9d required a prolonged reaction time (24 h) compared to that of 2b with 9b (15 min).6
The ΔE≠ values were in decreasing order: β-acetylvinyl ethers (3; Entries 6, 7), β,β-diacetylvinyl ether (4; Entries 11, 12), β-trifluoroacetylvinyl ethers (1; Entries 1-5), and β,β-bis(trifluoroacetyl)vinyl ethers (2; Entries 8-10). This order is consistent with the decreasing order of the reaction temperature required for the hetero Diels-Alder reactions of 1-4 with vinyl ethers (9); 3: 190-210 °C,7 4: 140 °C,8 1: 80 °C,5 and 2: rt-40 °C.6
We also estimated the ΔE≠ for the hetero Diels-Alder reactions of β,β-bis(trifluoroacetyl)vinyl ethers (2a,b) with ketene acetals and vinyl sulfides instead of vinyl ethers. 1,1-Dimethoxyethylene (10a) was selected as a model compound for the ketene acetals. In the case of the cycloaddition of 2b with 10a (Entry 13), an ΔE≠ of less than 4 kcal/mol was estimated. In contrast, the predicted ΔE≠ of the reaction of 2a and 2b with vinyl sulfides (11a,b; Entries 14, 15) was relatively high in comparison with that of the corresponding reaction with vinyl ethers (9a,b; Entries 8, 9). These results explain why the reaction of 2b with ethyl vinyl sulfide (11b) proceeds more slowly than that of 2b with ethyl vinyl ether (9b),6 even when the HOMO-LUMO gap (ΔEf1) between 2b and 11b (Table 4, Entry 12) is narrower than that between 2b and 9b (Table 4, Entry 2).
The difference in ΔE≠ on the reactions of 9, 10, and 11 would be attributed to stronger electron-donating ability of oxygen compared to sulfur. The terminal olefin carbon of the ketene acetal (10a) bearing two methoxy groups has more negative charge than that of vinyl ether (9a). On the other hand, the terminal olefin carbon of vinyl sulfide (11a) has less negative charge than 9a. A stronger electrostatic interaction between 10a and electron deficient 2b would assist the molecular orbital interaction, in particular, that of C(2’)-C(1) on the transition state (Figure 2), thus further decreasing ΔE≠. In contrast, the reverse would be true on the transition state of the reaction of 2b with 11a.
CONCLUSION
Molecular orbital calculations suggest that the efficiency of heterodienes (1-4) on hetero Diels-Alder reaction with electron-rich alkenes (9-11) can be attributed to energy level gaps (ΔEf1) between the LUMO of 1-4 and the HOMO of 9-11. We confirmed these results by estimating the activation energies (ΔE≠) of these hetero Diels-Alder reactions. It is worth noting that one trifluoroacetyl group in the heterodienes (1) lowers the activation energy of the hetero Diels-Alder reaction more effectively than the two acetyl groups in 4. A more stable endo type transition state in the hetero Diels-Alder reaction of β-trifluoroacetylvinyl ethers (1) and β,β-bis(trifluoroacetyl)vinyl ethers (2) with ethyl vinyl ether (9) explains the formation of the major product, the cis-isomers of dihydropyrans (5, 6).
COMPUTATIONAL METHODS
All calculations employed in this paper were accomplished using the computer programs packages SPARTAN and PC SPARTAN 04.15 All calculations for geometrical optimizations were performed with the 6-31G* basis set at B3LYP 16 level. The starting geometries employed for all optimizations were resulted from molecular mechanics using SYBYL17 force field and subsequent semi-empirical PM318 optimizations. The calculations for transition state geometries and their energies were also taken with the 6-31G* basis set at B3LYP level.
References
1. R. Filler and Y. Kobayashi, ‘Biomedicinal Aspects of Fluorine Chemistry,’ Kodansha & Elsevier Biomedical, Tokyo, 1982.
2. R. Filler, ‘Organofluorine Chemicals and Their Industrial Applications,’ Ellis Horwood, London, 1979.
3. J. T. Welch, Tetrahedron, 1987, 43, 3123. CrossRef
4. R. Filler, Y. Kobayashi, and L. M. Yagupolskii, ‘Organofluorine Compounds in Medicinal Chemistry and Biomedical Applications,’ Elsevier, Amsterdam, 1993.
5. M. Hojo, R. Masuda, and E. Okada, Synthesis, 1989, 215. CrossRef
6. M. Hojo, R. Masuda, and E. Okada, Synthesis, 1990, 347. CrossRef
7. G. Berti, G. Catelani, F. Colonna, and L. Monti, Tetrahedron, 1982, 38, 3067. CrossRef
8. R. R. Schmidt and M. Maier, Tetrahedron Lett., 1982, 23, 1789. CrossRef
9. For instance, see; L. F. Tietze and G. Kettschau, Topics in Current Chemistry, 1997, 189, 1; CrossRef J. Esquivias, R. G. Arrayas, and J. C. Carretero, J. Am. Chem. Soc., 2007, 129, 1480. CrossRef
10. M. Hojo, R. Masuda, and E. Okada, Chem. Lett., 1990, 19, 113. CrossRef
11. M. Hojo, R. Masuda, and E. Okada, Synthesis, 1991, 46. CrossRef
12. M. Hojo, R. Masuda, and E. Okada, Synthesis, 1990, 425. CrossRef
13. N. Ota, E. Okada, A. Sonoda, N. Muro, D. Shibata, and M. Médebielle, Heterocycles, 2008, 76, 215. CrossRef
14. Correction for zero-point vibrational energy was not carried out.
15. Wavefunction, Inc.
16. A. D. Becke, J. Chem. Phys., 1993, 98, 5648. CrossRef
17. M. Clark, R. D. Cramer III, and N. van Opdensch, J. Computational Chem., 1989, 10, 982. CrossRef
18. J. J. P. Stewart, J. Computer Aided Molecular Design, 1992, 6, 69.