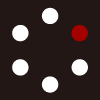
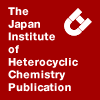
HETEROCYCLES
An International Journal for Reviews and Communications in Heterocyclic ChemistryWeb Edition ISSN: 1881-0942
Published online by The Japan Institute of Heterocyclic Chemistry
e-Journal
Full Text HTML
Received, 4th March, 2009, Accepted, 13th April, 2009, Published online, 13th April, 2009.
DOI: 10.3987/COM-09-S(S)17
■ Total Synthesis of (+)-Cacospongionolide B
Motoko Oshida, Misaki Ono, Atsuo Nakazaki, and Susumu Kobayashi*
Faculty of Pharmaceutical Sciences, Science University of Tokyo, 2641 Yamazaki, Noda, Chiba 278-8510, Japan
Abstract
Total synthesis of (+)-cacospongionolide B was achieved. The synthesis involved highly stereoselective C-glycosidation of a glycal derived from D-arabinose with 3-furyl boronic acid in the presence of a palladium catalyst and B-alkyl Suzuki–Miyaura coupling of in situ generated alkylborane prepared by the reaction of vinyl trans-decalin with alkenyl triflate.INTRODUCTION
(+)-Cacospongionolide B (1), isolated from the Mediterranean sponge Fasciospongia cavernosa, is a sesterterpenoid that exhibits antibacterial and cytotoxic activities,1 and an inhibition of secretory phospholipase A2 (sPLA2) with anti-inflammatory properties (Figure 1).2 Some related compounds such as cacospongionolide3a and cacospongionolides D,3b E,3c and F3d have been reported to exhibit the above mentioned activities. Intensive studies conducted by De Rosa and José Alcaraz have shown that (+)-1 controls nitric oxide, prostaglandin E2 (PGE2), and tumor necrosis factor-α (TNF-α) production in vitro and in vivo, effects likely dependent on NF-κB inhibition.4 Its ability to control NF-κB-dependent gene expression and regulate cellular functions would hold a potential therapeutic application for inflammatory diseases.
Cacospongionolides are composed of two units: (i) a hydrophobic moiety such as decalin or a C16 acyclic side chain and (ii) a dihydropyran moiety bearing γ-hydroxybutenolide. Although the latter one, especially the γ-hydroxybutenolide motif, has been considered to be a pharmacophore, Snapper and co-workers have revealed that γ-hydroxybutenolide is not the sole structural feature affecting its biological activities.5a
Because of their structural features as well as the important biological activities exhibited by them, cacospongionolides have attracted considerable attention, and their total synthesis has been reported. Snapper and co-workers have reported the total syntheses of (+)- and (–)-cacospongionolides B5a and E5c, which involved ring closing metathesis for constructing a dihydropyran moiety. Forsyth and co-workers have reported the total synthesis of (–)-cacospongionolide F5b by diastereochemically divergent synthesis using a racemic dehydrodecalin moiety and an enantiopure γ-hydroxybutenolide moiety that was masked as a furyl group. Herein, we report the total synthesis of (+)-1. The synthesis involved highly stereoselective C-glycosidation of a glycal6 derived from D-arabinose with 3-furyl boronic acid in the presence of a palladium catalyst and B-alkyl Suzuki–Miyaura coupling7 of in situ generated alkylborane prepared by the reaction of vinyl trans-decalin with alkenyl triflate.
RESULTS AND DISCUSSION
The synthetic strategy of (+)-1 is depicted in Scheme 1. According to the literatures,5,8 the γ-hydroxybutenolide moiety in (+)-1 would be synthesized from the 3-furyl group by using singlet oxygen through an endoperoxide intermediate. The corresponding precursor of (+)-1 bearing a 3-furyl group could be obtained by assembling vinyl trans-decalin 15 and alkenyl triflate 10 via B-alkyl Suzuki–Miyaura coupling.7 Alkene 15 would be synthesized from ketoester 12, which in turn is readily available from known enone 119 derived from an enantiomerically pure 5-methyl Wieland–Miescher ketone. The asymmetric carbon centers located at C9 and C10 (numbered according to the cacospongionolide B) in 12 could be controlled by means of reductive alkylation. Triflate 10, which is another Suzuki–Miyaura coupling partner, would be synthesized by the conjugate reduction of the enone 8 and successive triflation of in situ generated enolate or the regioselective enolate formation of a saturated ketone 9 followed by triflation. These ketones would be prepared from allyl ester 5. On the basis of the recent reports on aryl C-glycosidation of a glycal in the presence of a palladium catalyst,6b,c we believed that the regio- and stereoselective C-glycosidation of a glycal derived from D-arabinose10 with 3-furyl boronic acid11 would be successful.
The synthetic routes to key substrates glycals 2 and 3-furyl boronic acid 4 are summarized in Scheme 2. We first prepared acetyl glycal 2a from D-arabinose according to the procedure given in the literature.10 Treatment of 2a with K2CO3 and MeOH gave diol 2b in 98% yield, which was then transformed into benzoyl glycal 2c and pivaloyl glycal 2d. 3-Furyl boronic acid 4 was synthesized from 3-bromofuran, according to the procedure stated in the literature,12 through bromine-lithium exchange, successive addition of triisopropyl borate, and acid hydrolysis.
Key aryl C-glycosidation of 2 with 4 was carried out using the reported method (Table 1).6b,c Thus, 2a was treated with 2 equiv of 4 in acetonitrile in the presence of 10 mol% of Pd(OAc)2 at rt under an oxygen atmosphere; this afforded desired furyl C-glycoside 5a as a single diastereomer but only in 2% yield (entry 1). Ring-opened compound 6 was mainly obtained, which would be generated by a mechanism similar to the one reported by de la Figuera.6c A coupling product bearing two furyl groups which generates from 5 was not observed. Increasing the temperature or amount of Pd(OAc)2 was not effective in this case (entries 2 and 3); decomposition of 2a was observed in the latter case. A similar reaction in the presence of Et3N or Cu(OAc)2 did not proceed (entries 4 and 5). When a mixed solvent system of toluene and EtOH, which has been found to prevent ring-opening reactions,6b was used, 5a was produced in quite low yield (entry 6). Although rather polar solvents such as DMSO and DMI (1,3-dimethyl-2-imidazolidinone) were ineffective, DMF was found to afford 5a in 21% yield (entry 9). In addition, a similar reaction using 0.2 M of 2a in NMP (N-methylpyrrolidone) provided 5a in 29% yield (entry 10), but the yield was observed to be low when higher concentration of 2a was used (0.5 M, entry 11). The stereochemical determination of glycoside 5a was confirmed by large coupling constants (J = ~10 Hz) of acetate 7, which indicates that the acetyl group and the 3-furyl group were located at the equatorial position (Scheme 3). A similar reaction using the benzoyl surrogate 2c resulted in the formation of corresponding C-glycoside 5c in the best yield (38%, entry 12). Unfortunately, 2d, which was protected by pivaloyl groups, was found to be an unsuitable substrate for the reaction (entry 13).
Scheme 4 summarizes the transformation of the allyl acetate 5a into alkenyl triflate 10. The hydrolysis and oxidation of the resulting allyl alcohol gave requisite enone 8 in 90% yield. The direct formation of triflate 10 from enone 8 by means of conjugate reduction and successive triflation of the transient enolate with PhNTf213 was attempted; however, a trace amount of 10 was observed. Therefore, we chose regioselective enolate formation and triflation using ketone 9, which was prepared from enone 8 via hydrogenation under conventional conditions. Treatment of 9 with a slight excess of KHMDS in THF at –78 °C followed by treatment with the same amount of Comins’ reagent14 led to 10 in 71% yield as a single regioisomer. When PhNTf2 was used as a triflation reagent with a variety of bases (KHMDS, LHMDS, or LDA), the reaction was found to provide 10 in low yield (up to 33%).
Indispensable vinyl trans-decalin 15 was synthesized from enantioenriched 5-methyl Wieland-Miescher ketone derivative 11 (Scheme 5). Treatment of enone 11 (97.4% ee), which was prepared from 2-methyl cyclohexan-1,3-dione,9 with lithium in liquid ammonia, and H2O followed by ethyl bromoacetate afforded trans-decalin 12 in moderate yield with high stereoselectivity.15 The newly generated stereogenic centers were confirmed from the nOe correlations of ester 13, as shown in Figure 2. A ketone carbonyl group in 12 was transformed into an exo-methylene group, which was then hydrogenated under several conditions. Hydrogenation using Pd/C or Adams’ catalyst either yielded 13 with almost no diastereoselectivity (ca. 1:1) or was irreproducible. In contrast, hydrogenation at 10 atm with Wilkinson’s catalyst resulted in the formation of the desired 13 along with epi-13 in 73% and 17% yields, respectively. Ester 13, in turn, was reduced using LiAlH4 to obtain primary alcohol 14 in 95% yield. The employment conditions reported by Grieco and co-workers16,7c resulted in the clean conversion of alcohol 14 to desired vinyl decalin 15.
To union trans-decalin 15 and alkenyl triflate 10, we next carried out the crucial Suzuki–Miyaura coupling (Scheme 6).7c A degassed solution of 15 in THF was treated with 2 equiv of 9-BBN dimer. The in situ generated alkylborane was then treated with a degassed solution of 10, PdCl2(dppf)·CH2Cl2, and Cs2CO3 in 1,4-dioxane-H2O to afford 16 in 83% yield and having spectral characteristics identical to those reported by Snapper and co-workers.5a
With all carbon skeleton bearing requisite stereogenic centers in hand, we followed Snapper’s protocol to complete the total synthesis. Deprotection and one-carbon homologation of the resulting ketone 17 provided 18 in good overall yield. The photo-oxidation of the furan moiety with singlet oxygen in the presence of a hindered base was finally achieved to obtain the final product (+)-1 as a mixure of epimers. The 1H and 13C NMR spectra and the specific rotation of 1 synthesized by our method were consistent with those of 1 synthesized by a previous method.5a
In conclusion, we have achieved the total synthesis of (+)-cacospongionolide B via highly stereoselective aryl C-glycosidation and B-alkyl Suzuki–Miyaura coupling. This approach offers a potentially useful synthetic route to other cacospongionolides.
EXPERIMENTAL
General Techniques. 1H and 13C NMR spectra were recorded on a JEOL JNM-LD400 spectrometer operating at either 400 MHz (1H) or 100 MHz (13C) or on a JEOL AL-300 operating at either 300 MHz (1H) or 75 MHz (13C). Chemical shifts are reported in δ units and are referenced to the solvent, i.e., 7.26/77.1 for CDCl3. Multiplicities are indicated as: br (broadened), s (singlet), d (doublet), t (triplet), q (quartet), quint (quintet), sept (septet) or m (multiplet). Coupling constants (J) are reported in Hertz (Hz). Infrared spectra were recorded on a Jasco FT-IR410 spectrometer. Electron impact mass spectra were performed on a Hitachi M-80B mass spectrometer. Electrospray ionization mass spectra were recorded on an Applied Biosystems API QSTAR pulsar i as high resolution, using poly(ethylene glycol) as internal standard. Thin-layer chromatography (TLC) was performed on silica gel 60 F254 (Merck 1.05715.0009) plates. Flash column chromatography was performed on a PSQ100B silica gel (Fuji Silysia Co., Ltd., Japan). THF and Et2O were purchased from Wako Pure Chemical Industries Ltd. in anhydrous grade. CH2Cl2 was distilled from CaH2 immediately before use. NMP, benzene, DMSO and DMF were distilled from CaH2 and stored over activated MS 4A. Pyridine, Et3N, i-Pr2NEt and i-Pr2NH were distilled from CaH2 and stored over KOH. Other reagents were used as received. All moisture sensitive reactions were performed under a static argon atmosphere in flame-dried glassware.
Benzoyl glycal 2c. Acetyl glycal 2a was prepared from D-arabinose according to the literature procedure.10
To a solution of acetyl glycal 2a (300 mg, 1.5 mmol) in MeOH (1.9 mL) was added K2CO3 (2 mg, 0.02 mmol), and the resulting mixture was stirred for 26 h at rt. The solvent was evaporated under reduced pressure and the residue was purified by silica gel column chromatography (hexane/EtOAc = 1:1) to give diol 2b (163 mg, 93%) as a white solid.
To a solution of diol 2b (61 mg, 0.53 mmol) in pyridine (0.13 mL, 1.58 mmol) at 0 °C was added benzoyl chloride (0.13 mL, 1.16 mmol). The resulting solution was allowed to warm to rt and stirred at that temperature for 19 h. The reaction mixture was diluted with CH2Cl2 and H2O, extracted with CH2Cl2. The combined organic layers were washed with brine, dried over Na2SO4, filtered and concentrated under reduced pressure. Purification by silica gel column chromatography (hexane/EtOAc = 30:1) gave benzoyl glycal 2c (168 mg, 98%) as a colorless oil: Rf 0.67 (hexane/EtOAc = 2:1); [α]D23 +211.7 (c 0.39, CHCl3); 1H NMR (400 MHz, CDCl3) δ 8.03-7.92 (m, 4H), 7.58-7.34 (m, 6H), 6.62 (d, J = 6.1 Hz, 1H), 5.81 (dd, J = 5.1, 5.1 Hz, 1H), 5.54 (dt, J = 5.1, 9.0 Hz, 1H), 5.07 (dd, J = 5.1, 6.1 Hz, 1H), 4.29-4.21 (m, 2H); 13C NMR (100 MHz, CDCl3) 165.9, 165.4, 148.1, 133.3, 133.1, 130.1, 129.8 (2 carbons), 129.6 (2 carbons), 129.4, 128.4 (4 carbons), 97.7, 66.8, 63.6, 63.0; IR (neat, cm–1) 1716, 1644, 1523, 1276; HRMS (FAB+) calcd for C19H16O5Na [M+Na]+ 347.0998, found: 347.0896.
Furyl C-glycoside 5c. To a mixture of glycal 2c (200 mg, 0.62 mmol) and furylboronic acid 4 (139 mg, 1.24 mmol) in NMP (3.1 mL) was added Pd(OAc)2 (14 mg, 0.06 mmol). The resulting suspension was refilled with oxygen and stirred at rt for 12 h. The reaction mixture was diluted with hexane/EtOAc (4:1, 2.0 mL) and filtered through a pad of silica gel. The filtrate was washed with H2O and brine, dried over Na2SO4, filtered and concentrated under reduced pressure. Purification by silica gel column chromatography (hexane/EtOAc = 20:1) gave furyl C-glycoside 5c (64 mg, 38%, >95% dr determined by 1H NMR analysis) as a yellow oil: Rf 0.57 (hexane/EtOAc = 4:1); [α]D24 +265.2 (c 0.25, CHCl3); 1H NMR (400 MHz, CDCl3) δ 8.09 (m, 2H), 7.57 (tt, J = 1.5, 7.3 Hz, 1H), 7.47 (s, 1H), 7.40 (m, 2H), 7.39 (s, 1H), 6.45 (s, 1H), 6.22 (m, 1H), 6.14 (ddd, J = 1.9, 3.9, 10.3 Hz, 1H), 5.40 (dt, J = 3.9, 3.9 Hz, 1H), 5.24 (br s, 1H), 4.10 (dd, J = 3.9, 12.4 Hz, 1H), 3.86 (dd, J = 3.9, 12.4 Hz, 1H); 13C NMR (100 MHz, CDCl3) 166.2, 143.6, 140.9, 133.1, 133.0, 130.0, 129.8 (2 carbons), 128.4 (2 carbons), 124.1, 123.6, 109.8, 67.6, 65.0, 64.0; IR (neat, cm–1) 1714; HRMS (FAB+) calcd for C16H14O4Na [M+Na]+ 293.0893, found: 294.0970.
Furyl C-glycoside 5a. Isolated as a yellow oil: Rf 0.30 (hexane/EtOAc = 4:1); [α]D24 +219.2 (c 0.31, CHCl3); 1H NMR (400 MHz, CDCl3) δ 7.42 (dd, J = 1.7, 1.7 Hz, 1H), 7.35 (br s, 1H), 6.41 (br s, 1H), 6.18 (ddd, J = 1.2, 3.0, 10.2 Hz, 1H), 6.03 (dddd, J = 0.7, 2.2, 4.2, 10.2 Hz, 1H), 5.19 (br s, 1H), 5.13 (ddt, J = 1.2, 3.6, 4.2 Hz, 1H), 3.96 (dd, J = 3.6, 12.4 Hz, 1H), 3.72 (ddd, J = 0.7, 3.6, 12.4 Hz, 1H), 2.11 (s, 3H); 13C NMR (100 MHz, CDCl3) 170.7, 143.6, 141.0, 132.9, 123.9, 123.5, 109.9, 67.5, 64.5, 63.7, 21.1; IR (neat, cm–1) 1731; HRMS (ESI) calcd for C11H12O4Na [M+Na]+ 231.0627, found: 231.0633.
Enone 8. To a solution of furyl C-glycoside 5a (76 mg, 0.36 mmol) in MeOH (2.4 mL) was added NaOMe (49 mg, 0.91 mmol), and then the resulting mixture was stirred for 15 min at rt. The reaction was quenched with a saturated aqueous solution of NH4Cl at 0 °C, extracted with CH2Cl2, dried over Na2SO4, filtered and concentrated under reduced pressure.
To a solution of the crude allyl alcohol in DMSO (2.4 mL) was added IBX (122 mg, 0.44 mmol), and then the solution was stirred for 2.5 h at rt. The reaction was quenched with H2O, and the aqueous layer was extracted with Et2O. The combined organic layers were dried over Na2SO4 and concentrated under reduced pressure. Purification by silica gel column chromatography (hexane/EtOAc = 5:1) gave enone 8 (54 mg, 90% for the two steps) as a yellow oil: Rf 0.73 (hexane/EtOAc = 1:1); [α]D23 +117.4 (c 0.93, CHCl3); 1H NMR (400 MHz, CDCl3) δ 7.47 (m, 1H), 7.44 (m, 1H), 7.13 (dd, J = 2.7, 10.2 Hz, 1H), 6.46 (br s, 1H), 6.24 (dd, J = 2.0, 10.2 Hz, 1H), 5.39 (br s, 1H), 4.27 (d, J = 16.6 Hz, 1H), 4.17 (d, J = 16.6 Hz, 1H); 13C NMR (100 MHz, CDCl3) 194.3, 149.3, 144.0, 140.9, 127.1, 122.1, 109.5, 69.8, 68.1; IR (neat, cm–1) 1696, 1617; HRMS (ESI) calcd for C9H8O3Na [M+Na]+ 187.0365, found: 187.0360.
Ketone 9. A solution of Pd/C (10 wt%, 9 mg) and enone 8 (53 mg, 0.32 mmol) in EtOH (3.2 mL) was refilled with hydrogen. The reaction mixture was stirred for 3.5 h at rt and then passed directly through a short pad of Celite. The filtrate was concentrated under reduced pressure. Purification by silica gel column chromatography (hexane/EtOAc = 20:1) gave ketone 9 (49 mg, 92%) as a yellow oil: Rf 0.67 (hexane/EtOAc = 1:1); [α]D24 +12.8 (c 0.94, CHCl3); 1H NMR (400 MHz, CDCl3) δ 7.44 (br s, 1H), 7.42 (t, J = 1.7 Hz, 1H), 6.43 (br s, 1H), 4.77 (dd, J = 3.4, 10.0 Hz, 1H), 4.21 (dd, J = 1.5, 16.4 Hz, 1H), 4.11 (d, J = 16.4 Hz, 1H), 2.70-2.55 (m, 2H), 2.38-2.31 (m, 1H), 2.28-2.18 (m, 1H); 13C NMR (100 MHz, CDCl3) 207.1, 143.5, 139.3, 125.5, 108.7, 73.9, 70.5, 37.0, 30.5; IR (neat, cm–1) 1725; HRMS (ESI) calcd for C9H10O3Na [M+Na]+ 189.0527, found: 189.0525.
Alkenyl triflate 10. To a solution of ketone 9 (83 mg, 0.50 mmol) in THF (5.0 mL) was added Comins’ reagent (205 mg, 0.52 mmol), and then the resulting mixture was cooled to −78 °C. To this mixture was added dropwise KHMDS (0.5 M solution in toluene, 1.1 mL, 0.52 mmol) and the resulting mixture was stirred at that temperature for 2 h. The reaction was quenched with a saturated aqueous solution of NH4Cl at 0 °C, and extracted with Et2O. The combined organic layers were dried over Na2SO4, filtered and concentrated under reduced pressure. Purification by silica gel column chromatography (hexane/ EtOAc = 20:1) gave alkenyl triflate 10 (106 mg, 71%) as a pale yellow oil: Rf 0.87 (hexane/EtOAc = 2:1); [α]D23 +45.2 (c 0.73, CHCl3); 1H NMR (400 MHz, CDCl3) δ 7.44 (br s, 1H), 7.42 (br s, 1H), 6.42 (br s, 1H), 5.79 (br s, 1H), 4.63 (dd, J = 4.2, 9.0 Hz, 1H), 4.36 (br d, J = 15.8 Hz, 1H), 4.24 (br d, J = 15.8 Hz, 1H), 2.63-2.47 (m, 2H); 13C NMR (100 MHz, CDCl3) 145.6, 143.4, 139.5, 124.6, 118.3 (q, J = 318.3 Hz), 115.3, 108.6, 68.2, 63.8, 30.3; IR (neat, cm–1) 1601, 1213, 1140; HRMS (ESI) calcd for C10H9F3O5SNa [M+Na]+ 321.0015, found: 321.0027.
Ketoester 12. Enone 11 was prepared from 2-methylcyclohexan-1,3-dione according to the literature procedure,9 and the enantiomeric excess of 5-methyl Wieland-Miescher ketone was determined by chiral HPLC analysis (Daicel, Chiralpak AD-H, 1.0 mL/min, hexane/i-PrOH = 90:10, 254 nm, 30 °C) to be 97.4% ee.
To a stirred mixture of lithium (144 mg, 20.8 matom) in liquid ammonia (ca. 100 mL) at −78 °C was added dropwise a solution of enone 11 (981 mg, 4.15 mmol) in THF (2.0 mL). After refluxing for 1 h at −30 °C, the reaction was quenched with H2O (3.9 M in THF, 1.1 mL, 4.3 mmol). After refluxing the resulting mixture for another 1 h, ethyl bromoacetate (2.3 mL, 20.8 mmol) was then added as rapidly as possible, and the reaction mixture was refluxed for 2 h. The ammonia was evaporated and the reaction was quenched with a saturated aqueous solution of NH4Cl. The resulting mixture was then extracted with Et2O, and the combined organic layers were dried over Na2SO4, filtered and concentrated under reduced pressure. Purification by silica gel column chromatography (hexane/EtOAc = 20:1) gave ketoester 12 (638 mg, 47%, >95% dr determined by 1H NMR analysis) as a colorless oil: Rf 0.47 (hexane/EtOAc = 2:1); [α]D25 +23.8 (c 1.00, CHCl3); 1H NMR (400 MHz, CDCl3) δ 4.18-4.12 (m, 2H), 3.94-3.86 (m, 5H), 2.90 (d, J = 13.9 Hz, 1H), 2.50 (dd, J = 4.6, 9.8 Hz, 2H), 2.39 (d, J = 16.9 Hz, 1H), 2.31 (dd, J = 3.6, 11.0 Hz, 1H), 2.19-2.01 (m, 1H), 1.78-1.63 (m, 3H), 1.58-1.51 (m, 1H), 1.47-1.37 (m, 2H), 1.24 (t, J = 7.1 Hz, 3H), 1.21 (s, 3H), 1.04 (s, 3H); 13C NMR (100 MHz, CDCl3) 215.3, 171.8, 112.6, 65.3, 65.0, 60.4, 48.7, 44.3, 43.4, 42.6, 34.7, 30.4, 27.1, 22.9, 22.3, 22.1, 15.4, 14.1; IR (neat, cm–1) 1723, 1702, 1184; HRMS (ESI) calcd for C18H28O5Na [M+Na]+ 347.1828, found: 347.1821.
exo-Methylene. A mixture of methyltriphenylphosphonium bromide (1.20 g, 3.34 mmol) and t-BuOK (1.0 M solution in THF, 3.3 mL, 3.3 mmol) was stirred for 1 h at rt. To the resulting mixture was added a solution of ketoester 12 (361 mg, 1.11 mmol) in THF (7.8 mL), and then the reaction mixture was stirred at that temperature for 3.5 h. The reaction was quenched with H2O, and the aqueous layer was extracted with Et2O. The combined organic layers were dried over Na2SO4, filtered and concentrated under reduced pressure. Purification by silica gel column chromatography (hexane/EtOAc = 30:1) gave exo-methylene (237 mg, 65%) as a colorless oil: Rf 0.73 (hexane/EtOAc = 2:1); [α]D25 −4.0 (c 1.27, CHCl3); 1H NMR (400 MHz, CDCl3) δ 4.79 (s, 1H), 4.76 (s, 1H), 4.09 (q, J = 7.1 Hz, 2H), 3.94-3.89 (m, 3H), 3.86-3.81 (m, 1H), 2.49 (d, J = 2.9 Hz, 2H), 2.40 (dt, J = 5.2, 12.7 Hz, 1H), 2.22-2.16 (m, 2H), 1.75-1.59 (m, 3H), 1.52-1.45 (m, 2H), 1.42-1.36 (m, 2H), 1.27-1.20 (m, 1H), 1.25 (t, J = 7.1 Hz, 3H), 1.14 (s, 3H), 1.07 (s, 3H); 13C NMR (100 MHz, CDCl3) 171.7, 154.0, 113.3, 107.0, 65.2, 64.8, 60.0, 44.8, 44.0, 43.5, 42.8, 31.2, 30.3, 29.4, 23.6, 22.8, 21.8, 17.6, 14.2; IR (neat, cm–1) 1734, 1637, 1185; HRMS (ESI) calcd for C19H30O4Na [M+Na]+ 345.2036, found: 345.2035.
Ester 13. To a 50 mL stainless steel autoclave were introduced a solution of the exo-methylene (163 mg, 0.51 mmol) in toluene (5.1 mL) and (Ph3P)3RhCl (94 mg, 0.10 mmol). The autoclave was purged three times with hydrogen, and then the pressure of hydrogen was set to 10 atm. The resulting mixture was stirred at rt for 3 days, and then the hydrogen was released carefully. The solvent was removed under reduced pressure. Purification by silica gel chromatography (hexane/EtOAc = 30:1) gave the desired ester 13 (121 mg, 73%, >95% dr) and epi-13 (29 mg, 17%, >95% dr) as a yellow oil. The relative stereochemistry of ester 13 was established by the nOe correlations as shown in Figure 2: Rf 0.60 (hexane/i-Pr2O = 2:1); [α]D25 +23.2 (c 0.56, CHCl3); 1H NMR (400 MHz, CDCl3) δ 4.10 (dq, J = 3.7, 7.1 Hz, 1H), 4.08 (dq, J = 3.7, 7.1 Hz, 1H), 4.00-3.90 (m, 3H), 3.87-3.80 (m, 1H), 2.35 (d, J = 14.9 Hz, 1H), 2.13 (d, J = 14.9 Hz, 1H), 1.95-1.87 (m, 2H), 1.76 (dt, J = 2.9, 11.7 Hz, 2H), 1.68-1.60 (m, 1H), 1.52-1.44 (m, 3H), 1.49-1.35 (m, 2H), 1.32-1.25 (m, 1H), 1.24 (t, J = 7.1 Hz, 3H), 1.13 (s, 3H), 1.10 (s, 3H), 1.08-1.07 (m, 1H), 1.03 (d, J = 6.8 Hz, 3H); 13C NMR (100 MHz, CDCl3) 172.7, 113.5, 65.3, 64.9, 59.7, 44.3, 42.3, 38.8, 35.5, 31.6, 30.3, 24.9, 23.5, 23.0, 22.6, 21.1, 17.4, 15.1, 14.2; IR (neat, cm–1) 1734, 1188; HRMS (ESI) calcd for C19H32O4Na [M+Na]+ 347.2198, found: 347.2210.
Alcohol 14. To a suspension of LiAlH4 (133 mg, 3.51 mmol) in THF (10.0 mL) was added a solution of ester 13 (285 mg, 0.88 mmol) in THF (12.0 mL) at 0 °C, and then the resulting mixture was stirred at that temperature for 5.5 h. The mixture was carefully diluted with a saturated aqueous solution of NH4Cl, and the aqueous layer was extracted with Et2O. The combined organic layers were dried over Na2SO4, filtered, and concentrated under reduced pressure. Purification by silica gel column chromatography (hexane/EtOAc = 10:1) gave alcohol 14 (235 mg, 95%) as a colorless oil: Rf 0.30 (hexane/EtOAc = 2:1); [α]D23 +31.4 (c 0.43, CHCl3); 1H NMR (400 MHz, CDCl3) δ 4.00-3.90 (m, 3H), 3.85-3.79 (m, 1H), 3.75-3.63 (m, 2H), 1.87 (tt, J = 3.6, 13.4 Hz, 1H), 1.77 (dd, J = 3.2, 13.4 Hz, 1H), 1.71-1.62 (m, 4H), 1.60-1.58 (m, 1H), 1.52-1.37 (m, 5H), 1.28-1.22 (m, 2H), 1.09 (s, 3H), 1.07-1.06 (m, 1H), 1.00 (d, J = 7.1 Hz, 3H), 0.95 (s, 3H); 13C NMR (100 MHz, CDCl3) 113.6, 65.3, 64.8, 59.3, 43.8, 43.0, 42.6, 37.4, 36.0, 30.3, 24.9, 23.6, 23.1, 21.2, 20.0, 17.6, 14.9; IR (neat, cm–1) 3347, 1454; HRMS (ESI) calcd for C17H30O3Na [M+Na]+ 305.2195, found: 305.2187.
Vinyl trans-decalin 15. To a stirred solution of alcohol 14 (305 mg, 1.08 mmol) in THF (5.4 mL) were added 2-nitrophenyl selenocyanate17 (367 mg, 1.62 mmol) and n-Bu3P (0.54 mL, 2.16 mmol) at 0 °C. The mixture was stirred at rt for 22.5 h and concentrated under reduced pressure. Purification by silica gel column chromatography gave selenoether.
To a stirred solution of the selenoether in THF (21 mL) were added pyridine (0.44 mL, 5.39 mmol) and 31% H2O2 (0.28 mL, 2.56 mmol) at 0 °C, and then the mixture was stirred at rt for 1 h. The reaction mixture was diluted with ice water, and the aqueous layer was extracted with EtOAc. The combined organic layers were washed with brine, dried over Na2SO4, filtered and concentrated under reduced pressure. Purification by silica gel column chromatography (hexane/CH2Cl2 = 20:1) gave vinyl trans-decalin 15 (233 mg, 82% for the two steps) as a yellow oil: Rf 0.87 (hexane/EtOAc = 2:1); [α]D23 +14.7 (c 1.26, CHCl3); 1H NMR (400 MHz, CDCl3) δ 5.74 (dd, J = 11.0, 17.6 Hz, 1H), 4.94 (dd, J = 1.5, 11.0 Hz, 1H), 4.88 (dd, J = 1.5, 17.6 Hz, 1H), 3.99-3.91 (m, 3H), 3.87-3.82 (m, 1H), 1.94 (tt, J = 3.7, 13.6 Hz, 1H), 1.84-1.74 (m, 2H), 1.68-1.58 (m, 2H), 1.52-1.40 (m, 3H), 1.31-1.23 (m, 3H), 1.12 (s, 3H), 1.10-1.08 (t, J = 3.7 Hz, 1H), 1.05 (s, 3H), 1.00 (d, J = 7.1 Hz, 3H); 13C NMR (100 MHz, CDCl3) 150.2, 113.6, 110.7, 65.3, 64.9, 43.3, 41.9, 41.3, 39.9, 30.8, 25.6, 23.9, 23.1, 21.7, 19.5, 17.6, 16.0; IR (neat, cm–1) 1631; HRMS (ESI) calcd for C17H28O2Na [M+Na]+ 287.2089, found: 287.2087.
B-Alkyl Suzuki-Miyaura coupling of 10 and 15. To a solution of vinyl trans-decalin 15 (25 mg, 0.09 mmol) in THF (0.9 mL) that was degassed by sparging with Ar through a submerged needle for 15 min was added 9-BBN dimer (46 mg, 0.19 mmol). The resulting mixture was stirred at rt for 7 h to give a THF solution of alkylborane. To a degassed solution of alkenyl triflate 10 (49 mg, 0.16 mmol), PdCl2(dppf)⋅CH2Cl2 (8 mg, 0.01 mmol) and a solution of Cs2CO3 (1.0 M solution in H2O, 0.28 mL, 0.28 mmol) in 1,4-dioxane (0.5 mL) was added the solution of above-mentioned alkylborane via cannula, and rinse (1,4-dioxane, 0.4 mL) of the flask of alkylborane was added to the reaction mixture. The resulting mixture was stirred at rt for 14.5 h and diluted with H2O and Et2O. The aqueous layer was extracted with EtOAc. The combined organic layers were dried over Na2SO4, filtered and concentrated under reduce pressure. Purification by silica gel column chromatography (hexane/EtOAc = 80:1) gave coupling product 16 (31 mg, 83% from 15) as a white solid: Rf 0.50 (hexane/EtOAc = 5:1); [α]D23 +43.3 (c 1.07, CHCl3); 1H NMR (400 MHz, CDCl3) δ 7.41 (s, 1H), 7.39 (t, J = 1.7 Hz, 1H), 6.42 (s, 1H), 5.55 (br s, 1H), 4.50 (dd, J = 3.6, 9.8 Hz, 1H), 4.23 (br d, J = 15.9 Hz, 1H), 4.10 (br d, J = 15.9 Hz, 1H), 4.00-3.90 (m, 3H), 3.85-3.80 (m, 1H), 2.38-2.18 (m, 2H), 1.89-1.41 (m, 12H), 1.29-1.16 (m, 3H), 1.10 (s, 3H), 1.08 (m, 1H), 0.95 (d, J = 7.1 Hz, 3H), 0.89 (s, 3H); 13C NMR (100 MHz, CDCl3) 143.8, 139.2, 138.1, 126.8, 117.0, 113.7, 108.9, 68.7, 68.4, 65.3, 64.8, 43.8, 42.8, 38.0, 37.4, 35.1, 31.4, 30.3, 26.1, 24.9, 23.7, 23.1, 20.3, 19.9, 17.6, 14.6; IR (neat, cm–1) 1454, 1382, 1062; HRMS (ESI) calcd for C26H38O4Na [M+Na]+ 437.2771, Found: 437.2766.
Ketone 17. Ketal 16 (43 mg, 0.10 mmol) was stirred in 1 N HCl/THF (v/v = 1:2, 1.5 mL) at rt for 18 h. The mixture was diluted with Et2O, and washed consecutively with water, a saturated aqueous solution of NH4Cl, and brine. The organic layer was dried over Na2SO4, filtered and concentrated under reduced pressure. Purification by silica gel column chromatography (hexane/EtOAc = 10:1) gave ketone 17 (36 mg, 92%) as a white solid: Rf 0.40 (hexane/EtOAc = 5:1); [α]D23 +54.3 (c 0.44, CHCl3); 1H NMR (300 MHz, CDCl3) δ 7.41 (br s, 1H), 7.39 (t, J = 1.8 Hz, 1H), 6.42 (br s, 1H), 5.57 (br s, 1H), 4.51 (dd, J = 3.7, 9.7 Hz, 1H), 4.23 (br d, J = 16.1 Hz, 1H), 4.11 (br d, J = 16.1 Hz, 1H), 2.59 (td, J = 7.0, 13.7 Hz, 1H), 2.39-2.18 (m, 3H), 2.07 (m, 1H), 1.90-1.46 (m, 8H), 1.37 (dd, J = 3.1, 10.8 Hz, 2H), 1.28 (m, 2H), 1.19 (s, 3H), 1.15 (m, 1H), 1.00 (s, 3H), 0.90 (d, J = 7.0 Hz, 3H); 13C NMR (100 MHz, CDCl3) 215.7, 143.1, 139.2, 137.8, 126.7, 117.3, 108.9, 68.7, 68.3, 49.3, 47.2, 38.5, 38.0, 37.4, 34.8, 31.3, 26.2, 26.1, 25.9, 24.58, 20.7, 20.1, 19.6, 14.4; IR (neat, cm–1) 1702, 1506, 1455, 1382, 1159, 1066, 1025, 875; HRMS (ESI) calcd for C24H34O3Na [M+Na]+ 393.2508, Found: 393.2505.
exo-Methylene 18. NaH (55% dispersion in mineral oil, 60 mg, 1.27 mmol) was stirred in dry DMSO (5.0 mL) at 75 °C for 1 h, then the solution was cooled to −5 °C. To the resulting mixture was added a solution of methyltriphenylphosphonium bromide (453 mg, 1.27 mmol) in warm DMSO (2.5 mL) dropwise over 30 min, and then the mixture was stirred at rt for 1 h.
To the Wittig reagent in DMSO (0.17 M, 2.8 mL, 0.48 mmol) was added ketone 17 (36 mg, 0.10 mmol), and the resulting mixture was stirred at 75 °C for 1 h. The reaction was quenched with a saturated aqueous solution of NH4Cl, and extracted with Et2O. The combined organic layers were washed with brine, dried over Na2SO4, filtered and concentrated under reduced pressure. Purification by silica gel column chromatography (hexane/EtOAc = 30:1) gave exo-methylene 18 (30 mg, 84%) as a white solid: Rf 0.67 (hexane/EtOAc = 7:1); [α]D23 +82.3 (c 0.61, CHCl3); 1H NMR (400 MHz, CDCl3) δ 7.40 (s, 1H), 7.38 (t, J = 1.7 Hz, 1H), 6.42 (s, 1H), 5.56 (br s, 1H), 4.52 (s, 1H), 4.50 (m, 1H), 4.48 (s, 1H), 4.22 (br d, J = 15.9 Hz, 1H), 4.11 (br d, J = 15.9 Hz, 1H), 2.17-2.38 (m, 3H), 2.09 (d, J = 13.4 Hz, 1H), 1.99 (tt, J = 3.4, 13.7 Hz, 1H), 1.90-1.78 (m, 4H), 1.60 (m, 2H), 1.53-1.22 (s, 5H), 1.17 (dd, J = 2.2, 12.2 Hz, 1H), 1.10 (s, 3H), 1.08 (m, 1H), 0.93 (s, 3H), 0.91 (d, J = 7.1 Hz, 3H); 13C NMR (100 MHz, CDCl3) 160.6, 143.1, 139.2, 138.1, 126.8, 117.1, 108.9, 102.0, 68.7, 68.4, 47.8, 40.4, 38.0, 37.9, 35.4, 32.9, 31.3, 30.2, 28.7, 26.1, 25.5, 21.3 (2 carbons), 20.5, 14.8; IR (neat, cm–1) 1637, 1454, 1378, 1159; HRMS (ESI) calcd for C25H36O2Na [M+Na]+ 391.2715, found: 391.2711.
(+)-Cacospongionolide B (1). To a solution of furan 18 (30 mg, 0.08 mmol) in CH2Cl2 (1.7 mL) was added Rose Bengal (4 mg, 0.004 mmol) and i-Pr2NEt (0.07 mL). The bright red solution was cooled to –78 °C, and irradiated with a 250 W halogen lamp while anhydrous O2 was bubbled through the solution for 15 min. The irradiation continued for a total of 4 h under a blanket of anhydrous O2. The reaction was then protected from light, and warmed to rt. The reaction was quenched with a saturated aqueous solution of oxalic acid (3.0 mL), and the resulting mixture was stirred until colorless (15 min). The aqueous layer was extracted with CH2Cl2, and the combined organic layers were dried over Na2SO4, filtered, and concentrated under reduced pressure. Purification by silica gel chromatography (hexane/Et2O = 40:1) gave (+)-cacospongionolide B (1) [13 mg, 39% (55% based on the recovered starting material), a mixture of epimers] as a white solid: Rf 0.73 (CHCl3/MeOH = 6:1); [α]D24 +122.5 (c 0.61, CHCl3); 1H NMR (400 MHz, CDCl3) δ 6.23 (d, J = 7.1 Hz, 0.5H), 6.09 (s, 1H), 6.02 (s, 0.5H), 5.55 (br s, 1H), 4.52 (s, 1H), 4.49 (s, 1H), 4.44 (dd, J = 3.2, 9.5 Hz, 0.5H), 4.39 (dd, J = 4.4, 9.5 Hz, 0.5H), 4.23-4.09 (m, 2H), 3.68 (t, J = 6.6 Hz, 1H), 2.38-2.18 (m, 3H), 2.09 (br d, J = 13.7 Hz, 1H), 1.99 (tt, J = 3.4, 14.2 Hz, 1H), 1.89-1.76 (m, 4H), 1.64-1.58 (m, 2H), 1.51-1.26 (m, 5H), 1.16 (dd, J = 2.4, 12.0 Hz, 1H), 1.10 (s, 3H), 1.08-1.03 (m, 1H), 0.93 (s, 3H), 0.91 (d, J = 7.1 Hz, 3H); 13C NMR (100 MHz, CDCl3) 170.2, 167.7, 166.9, 138.4, 138.0, 118.4, 117.4, 116.0, 115.8, 102.1, 97.5, 97.0, 69.9, 69.0, 68.4, 68.4, 47.8, 40.4, 38.0, 37.9, 35.4, 32.9, 30.3, 29.7, 29.4, 28.9, 28.7, 26.0, 25.5, 21.3, 20.5, 14.8; IR (neat, cm–1) 3345, 3083, 2931, 2854, 1760, 1637, 1454, 1380, 1130, 952; HRMS (ESI) calcd for C25H36O4Na [M+Na]+ 423.2511, found: 423.2611.
ACKNOWLEDGEMENTS
We express our gratitude to Dr. Ichiro Hayakawa, University of Tsukuba, for helpful suggestions on the preparation of 2-nitrophenyl selenocyanate and on the procedure of B-alkyl Suzuki–Miyaura coupling.
References
1. S. De Rosa, A. Crispino, A. De Giulio, C. Iodice, C. R. Pronzato, and N. Zavodnik, J. Nat. Prod., 1995, 58, 1776. CrossRef
2. P. García Pastor, S. De Rosa, A. De Giulio, M. Payá, and M. José Alcaraz, Br. J. Pharmacol., 1999, 126, 301. CrossRef
3. (a) S. De Rosa, S. De Stefano, and N. Zavodnik, J. Org. Chem., 1988, 53, 5020; CrossRef (b) S. De Rosa, A. De Giulio, A. Crispino, C. Iodice, and G. Tommonaro, Nat. Prod. Res., 1997, 10, 267; CrossRef (c) S. De Rosa, A. Crispino, A. De Giulio, C. Iodice, R. Benrezzouk, M. C. Terencio, M. L. Ferrándiz, M. José Alcaraz, and M. Payá, J. Nat. Prod., 1998, 61, 931; CrossRef (d) S. De Rosa, A. Crispino, A. De Giulio, C. Iodice, P. Amodeo, and T. Tancredi, J. Nat. Prod., 1999, 62, 1316. CrossRef
4. I. Posadas, S. De Rosa, M. C. Terencio, M. Payá, and M. José Alcaraz, Br. J. Pharmacol., 2003, 138, 1571. CrossRef
5. (a) A. K. Cheung and M. L. Snapper, J. Am. Chem. Soc., 2002, 124, 11584; CrossRef (b) D. Demeke and C. J. Forsyth, Org. Lett., 2003, 5, 991; CrossRef (c) A. K. Cheung, R. Murelli, and M. L. Snapper, J. Org. Chem., 2004, 69, 5712. CrossRef
6. For a review, see: (a) G. D. Daves, Jr., Acc. Chem. Res., 1990, 23, 201; CrossRef (b) J. Ramnauth, O. Poulin, S. Rakhit, and S. P. Maddaford, Org. Lett., 2001, 3, 2013; CrossRef (c) N. de la Figuera, P. Forns, J.-C. Fernàndez, S. Fiol, D. Fernàndez-Forner, and F. Albericio, Tertahedron Lett., 2005, 46, 7271. CrossRef
7. For reviews, see: (a) N. Miyaura and A. Suzuki, Chem. Rev., 1995, 95, 2457; CrossRef (b) S. R. Chemler, D. Trauner, and S. J. Danishefsky, Angew. Chem. Int. Ed., 2001, 40, 4544. For a recent example, see; CrossRef (c) I. Hayakawa, M. Ueda, M. Yamaura, Y. Ikeda, Y. Suzuki, K. Yoshizato, and H. Kigoshi, Org. Lett., 2008, 10, 1859. CrossRef
8. M. R. Kernan and D. J. Faulkner, J. Org. Chem., 1988, 53, 2773. CrossRef
9. H. Hagiwara and H. Uda, J. Org. Chem., 1988, 53, 2308. CrossRef
10. Z. Smiatacz, H. Myszka, and Z. Ciunik, Carbohydr. Res., 1988, 172, 171. CrossRef
11. W. J. Thompson and J. Gaudino, J. Org. Chem., 1984, 49, 5237. CrossRef
12. (a) J. Šrogl, M. Janda, and I. Stibor, Collect. Czech. Chem. Commun., 1970, 35, 3478; (b) J. K. Politis, J. C. Nemes, and M. D. Curtis, J. Am. Chem. Soc., 2001, 123, 2537. CrossRef
13. G. T. Crisp and W. J. Scott, Synthesis, 1985, 335. CrossRef
14. D. L. Comins and A. Dehghani, Tetrahedron Lett., 1992, 33, 6299. CrossRef
15. (a) A. S. Sarma and P. Chattopadhyay, J. Org. Chem., 1982, 47, 1727; CrossRef (b) J. An and D. F. Wiemer, J. Org. Chem., 1996, 61, 8775. CrossRef
16. P. A. Grieco, S. Gilman, and M. Nishizawa, J. Org. Chem., 1976, 41, 1485. CrossRef
17. Z. Časar, I. Leban, A. M. L. Maréchal, and D. Lorcy, J. Chem. Soc., Perkin Trans. 1, 2002, 1568. CrossRef