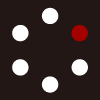
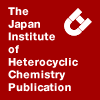
HETEROCYCLES
An International Journal for Reviews and Communications in Heterocyclic ChemistryWeb Edition ISSN: 1881-0942
Published online by The Japan Institute of Heterocyclic Chemistry
e-Journal
Full Text HTML
Received, 15th July, 2009, Accepted, 18th August, 2009, Published online, 19th August, 2009.
DOI: 10.3987/COM-09-S(S)55
■ Substituent Effects on the Electron Transfer-Initiated Photochemical Transformation of 1,2,4-Triazole-substituted α-Dehydroarylalaninamides into 2(1H)-Quinolinone Derivatives
Yuichi Yazawa, Minoru Suzuki, Tetsutaro Igarashi, and Tadamitsu Sakurai*
Material and Life Chemistry, Faculty of Engineering, Kanagawa University, 3-27-1 Rokkakubashi, Kanagawa-ku, Yokohama 221-8686, Japan
Abstract
An investigation was undertaken to elucidate substituent effects on the photoreactivity of 1,2,4-triazole-substituted α-dehydroarylalaninamides [(Z)-1] as well as on the selectivity of 2(1H)-quinolinone derivatives (2) from a synthetic point of view. It was found that photoinduced electron transfer-initiated cyclization of (Z)-1 bearing a meta-substituted phenyl or a 4-substituted naphthalen-1-yl group in methanol proceeds with a moderate to good efficiency affording the corresponding product 2 in a selectivity ranging from 33 to 100%.Excited-state chemistry for organic molecules has continued to contribute to the development of convenient methods for synthesizing pharmaceutically useful heterocyclic compounds.1 Particularly, photoinduced electron transfer (PET) reactions have attracted much recent attention owing to the potential that these reactions are able to construct many types of heteroatom-containing ring systems with high efficiencies.1a,c,d In the course of a systematic study on the PET reaction of N-acyl-α-dehydroarylalanines, we found that irradiation of (Z)-2-(3,5-dimethyl-1,2,4-triazol-4-yl)-3-(1-naphthyl)-2-propenamide and related derivatives [hereafter, referred to as 1,2,4-triazole-substituted α-dehydro(1-naphthyl)alaninamides] in methanol-triethylamine (TEA) afforded benzo[f]quinolinones in high selectivities, along with minor amounts of dihydrobenzo[f]quinolinones.2 In addition, anion radicals derived from triazole-substituted α-dehydro(1-naphthyl)alaninamides were shown to have a strong tendency to dissociate into the corresponding triazole anion and α-dehydronaphthylalanyl radicals, which readily cyclize to 2(1H)-benzo[f]quinolinones in the presence of the TEA cation radical. Although there are several synthetic studies aiming at the construction of a pharmaceutically useful quinolinone ring,3 convenient photochemical routes to quinolinone and its derivatives are scarcely known.4 It is, thus, of significance to develop a novel method for synthesizing 2(1H)-quinolinone derivatives through the PET-initiated cyclization of triazole-substituted α-dehydroarylalaninamides. In this communication we synthesized (Z)-2-(3,5-dimethyl-1,2,4-triazol-4-yl)-3-aryl-2-propenamides [(Z)-1a–i] and (Z)-2-(3-methyl-5-phenyl-1,2,4-triazol-4-yl)-3-aryl-2-propenamides [(Z)-1j–n] to examine substituent effects on the photoreactivity of (Z)-1 as well as on the selectivity of substituted 2(1H)-quinolinone as one of the (Z)-1-derived photoproducts (Chart 1).
The starting (Z)-isomers [(Z)-1a–n] were prepared in good yields by the ring-opening reactions of (Z)-4- arylmethylene-2-methyl-5(4H)-oxazolones with equimolar amounts of the corresponding N-substituted hydrazides in acetonitrile, followed by the condensation of the resulting 1,2,4-triazole-substituted 2-propenoic acids with methyl amine in 1,4-dioxane or dimethyl formamide.5 After a nitrogen-saturated methanol solution of (Z)-1a (4.0´10–3 mol dm–3, 500 mL) containing TEA (0.10 mol dm–3) was irradiated at wavelengths longer than 280 nm from a 400 W high-pressure Hg lamp for 60 min at room temperature (Pyrex glass filter, conversion 95%), the reaction mixture was subjected to preparative thin layer chromatography over silica gel (eluent: EtOAc). Usual workup allowed us to isolate 1-methyl-6-methoxy-2(1H)-quinolinone (2a; isolated yield, 20%), the regional isomer of which [1-methyl-8-methoxy-2(1H)-quinolinone] was not detected.6 On the other hand, a comparison of the 1H NMR spectrum of this reaction mixture with those of 3,4-dihydro-3-(3,5-dimethyl-1,2,4-triazol-4-yl)-1-methyl-2(1H)-benzo[f]quinolinone (3i) and 3,5-dimethyl-1,2,4-triazole isolated in a previous study showed that the corresponding dihydroquinolinone (3a) and triazole derivatives were produced along with (E)-1a and 4a (Scheme 1).2 The arylalaninamide derivative 4a was isolated by repeated preparative thin layer
chromatography and the 1H NMR spectral data substantiated the structure of this reduction product.7 The result that the photocyclization reaction of (Z)-1a proceeds without forming any byproducts enabled us to monitor this reaction by means of 1H NMR spectroscopy (Scheme 1). However, prolonged irradiation (>60 min) resulted in a side reaction to a definitely detectable extent depending on the structure of (Z)-1a–h and, hence, the 1H NMR composition of each compound was determined and compared after 30 min irradiation to analyze substituent effects on the photoreactivity of 1 and the selectivity of 2 (Table 1).
The data collected in Table 1 demonstrate that substituents introduced into the benzene ring of (Z)-1e exert great electronic and steric effects (which are beyond our expectations) on both the photoreactivity and the product composition. Replacement of hydrogens at the para- and meta-positions on the ring by methoxy or chloro groups increases the conversion of 1 but replacement at the latter position lowers the composition ratio of 2 to 3. In addition. the presence of a bulky methoxy group at the ortho-position (1c) or an electron-withdrawing cyano group at the meta-position (1h) resulted in a considerable decrease in photoreactivity. The finding that irradiation of (Z)-1i under the same conditions enables the complete conversion into the corresponding photoproducts 2i (isolated yield, 72%; selectivity, 76%) and 3i (R4= H, R5= Me in Scheme 2) confirms that the phenyl moiety in 1e has a much lower ability to accept an electron from TEA in its excited state, as compared to the naphthyl in 1i. As mentioned above, either a methoxy or a chloro substituent introduced at the meta-position on the benzene ring of (Z)-1e has a tendency to enhance the electron-accepting ability of this ring to exhibit the so-called “meta effect” although the selectivity of the quinolinone derivative 2 is decreased by this effect.8
In a previous study we found (through analysis of the effects of substituents attached to the triazole ring on the product composition) that the phenyl substituent increases the composition ratio of 2 to 3 by a factor of about 2 with keeping the conversion of 1 nearly constant.2 Additionally, taking into account the fact that methoxy and benzo groups increase both the photoreactivity of 1 and the selectivity of 2, we synthesized (Z)-1j–n to explore the meta effect as well as the effect of substituent (attached to the naphthalene ring) on the reactivity and selectivity. In Table 2 are summarized the conversion of 1 and the product composition, obtained under the same irradiation conditions as those for (Z)-1a–i. Replacement of one of the two methyl groups attached to the triazole ring by the phenyl increased the selectivity of 2 from 74% (2i) to 84% (2m), being consistent with our previous results (76%®86%).2 Interestingly, substitution of two methoxy groups for the meta-hydrogens (1j) enhanced not only the photoreactivity (conversion) of 1a (24%®79%) but also the selectivity of 2a (33%®71%). However, the presence of the fluoro-substituted naphthalene ring in 1n (R4= F, R5= Ph in Scheme 2) fairly reduced both the selectivity of the benzoquinolinone derivative 2m (84% for 2m and 40% for 2n) and the photoreactivity of 1m (R4= H, R5= Ph). Furthermore, the introduction of an electron-donating methyl group (1l) exerted only a very minor effect on these two parameters but replacement of this methyl group by a methoxy (1k) substantially reduced the conversion of 1m (100% for 1m and 13% for 1k) with the selective formation of 2k. As seen from these considerations, the selectivity of the benzoquinolinone derivative 2 has a clear tendency to increase with an increase in the electron-donating ability of the substituent R4 in (Z)-1k–n, whereas this substituent has a complicated influence on the excited-state reactivity of the triazole-substituted 1-naphthylalaninamide derivative 1.
We previously proposed (through a detailed analysis of solvent and substituent effects on the composition ratio of 2i to 3i) that the relative stability of the (E)-1i-derived anion radical intermediates (E)-IA and (E)-IB (which are in equilibrium with each other) determines the magnitude of this ratio (Scheme 3).2 Since electron-donating substituents such as methoxy and methyl is considered to shift the equilibrium to the
(E)-IB side by destabilizing the former intermediate, the finding that these substituents tend to enhance the selectivity of 2 provides additional evidence in support of our proposal. Taking into account that the photocyclization eventually yielding 2 and 3 is initiated by ET from TEA to the excited-state (E)-1, we propose that the photoreactivity of this (E)-isomer may be determined by the relative rate for ET to the (E)-isomer as well as by the relative rate for back ET from (E)-IA and (E)-IB to the TEA cation radical. It is thus likely that substituents introduced into the benzene or naphthalene ring of 1 exert their electronic and steric effects on these ET and back ET processes in a complicated manner. Negligible formation of the cyano-substituted products 2h and 3h may be due to either the exclusive deactivation of the excited-state (E)-1h or the exclusive back ET in (E)-IA. On the other hand, it seems strange that 1a and 1j underwent PET-initiated reduction to give 4a and 4j as minor products (Schemes 1 and 3). The meta effect of electron-donating methoxy groups in these two α-dehydroarylalanines might be responsible for the increased reactivity of the anion radical intermediate (E)-IA toward hydrogen abstraction from methanol.
As described above, the PET-initiated cyclization reactions of (Z)-1 bearing a methoxy group attached at the meta- or the para-position on the benzene ring (as well as an electron-donating or an electron-attracting group introduced into the naphthalene ring) were found to proceed with moderate to good efficiencies affording the corresponding quinolinone derivatives 2 in 33–100% selectivities. Taking the relatively high photostability of these derivatives into account, we may conclude that the photocyclization reactions provide a novel synthetic method for the construction of several substituted 2(1H)-quinolinone rings although the introduction of an electron-attracting group into the benzene or the naphthalene ring renders this method less attractive.
ACKNOWLEDGMENTS
This research was partially supported by a “Scientific Frontier Research Project” from the Ministry of Education, Sports, Culture, Science and Technology, Japan.
References
1. a) ‘Synthetic Organic Photochemistry,’ ed. by W. M. Horspool, Plenum, New York, 1984; b) ‘Handbook of Photochemistry and Photobiology,’ Vol. 2, ed. by H. S. Nalwa, American Scientific Publishers, Stevenson Ranch, 2003; c) ‘Synthetic Organic Photochemistry,’ ed. by A. G. Griesbeck and J. Mattay, Marcel Dekker, New York, 2005; d) A. G. Griesbeck, N. Hoffmann, and K. Warzecha, Acc. Chem. Res., 2007, 40, 128. CrossRef
2. K. Maekawa, A. Tomoda, T. Igarashi, and T. Sakurai, Heterocycles, 2009, 77, 739. CrossRef
3. O. Meth-Cohn, Heterocycles, 1993, 35, 539; CrossRef C. W. Holzapfel and C. Dwyer, Heterocycles, 1998, 48, 215; CrossRef K. Y. Lee and J. N. Kim, Bull. Korean Chem. Soc., 2002, 23, 939; B. Joseph, F. Darro, A. Behard, B. Lesur, F. Collignon, C. Decaestecker, A. Frydman, G. Guillaumet, and R. Kiss, J. Med. Chem., 2002, 45, 2543; CrossRef D. V. Kadnikov and R. C. Larock, J. Organomet. Chem., 2003, 687, 425; CrossRef M. Hadjeri, C.Beney, and A. Boumendjel, Curr. Org. Chem., 2003, 7, 679; CrossRef S. K. Chattopadhyay, R. Dey, and S. Biswas, Synthesis, 2005, 403; CrossRef Q. Li, K. W. Woods, W. Wang, N.-H. Lin, A. Claiborne, W.-Z. Gu, J. Cohen, V. S. Stoll, C. Hutchins, D. Frost, S. H. Rosenberg, and H. L. Sham, Bioorg. Med. Chem. Lett., 2005, 15, 2033; CrossRef M. Asahara, M. Ohtsutsumi, M. Tamura, N. Nishiwaki, and M. Ariga, Bull. Chem. Soc. Jpn., 2005, 78, 2235; CrossRef R. Bernini, S. Cacchi, G. Fabrizi, and A. Sferrazza, Heterocycles, 2006, 69, 99; CrossRef R. Bernini, S. Cacchi, I. De Salve, and G. Fabrizi, Synlett, 2006, 2947. CrossRef
4. T. Horaguchi, N. Hosokawa, K. Tanemura, and T. Suzuki, J. Heterocycl. Chem., 2002, 39, 61. CrossRef
5. Selected data for (Z)-1a: IR (KBr) ν/cm–1= 3232, 1666; 1H NMR (600 MHz, DMSO-d6) δ= 2.03 (6H, s), 2.70 (3H, d, J= 4.6 Hz), 3.60 (3H, s), 6.18 (1H, s), 6.44 (1H, d, J= 8.0 Hz), 6.95 (1H, d, J= 7.4 Hz), 7.25 (1H, dd, J= 7.4, 8.0 Hz), 7.78 (1H, s), 8.24 (1H, q, J= 4.6 Hz); 13C NMR (150 MHz, DMSO-d6) δ= 10.8 (2C), 27.1, 55.5, 114.0, 117.2, 122.4, 125.9, 130.9, 133.5, 135.6, 150.6, 160.0, 163.3.
6. Selected data for 2a: IR (KBr) ν/cm–1= 1678; 1H NMR (600 MHz, DMSO-d6) δ= 3.56 (3H, s), 3.78 (3H, s), 6.58 (1H, d, J= 9.7 Hz), 7.20 (1H, dd, J= 2.8, 9.7 Hz), 7.23 (1H, d, J= 2.8 Hz), 7.40 (1H, d, J= 9.2 Hz), 7.79 (1H, d, J= 9.2 Hz); 13C NMR (150 MHz, DMSO-d6) δ= 29.0, 55.5, 110.5, 115.9, 119.0, 120.8, 121.6, 134.2, 138.6, 154.1, 154.8, 160.6.
7. Selected data for 4a: 1H NMR (600 MHz, DMSO-d6) δ= 2.05 (6H, s), 2.63 (3H, d, J= 4.6 Hz), 2.99 (1H, dd, J= 11.6, 14.3 Hz), 3.38 (1H, dd, J= 4.6, 14.3 Hz), 3.63 (3H, s), 4.95 (1H, dd, J= 4.6, 11.6 Hz), 6.56 (1H, s), 6.57 (1H, d, J= 7.4 Hz), 6.73 (1H, d, J= 8.0 Hz), 7.10 (1H, dd, J= 7.4, 8.0 Hz), 8.06 (1H, q, J= 4.6 Hz).
8. H. E. Zimmerman and V. R. Sandel, J. Am. Chem. Soc., 1963, 85, 915; CrossRef H. E. Zimmerman, J. Am. Chem. Soc., 1995, 117, 8988; CrossRef H. E. Zimmerman, J. Phys. Chem. A, 1998, 102, 5616. CrossRef