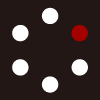
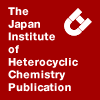
HETEROCYCLES
An International Journal for Reviews and Communications in Heterocyclic ChemistryWeb Edition ISSN: 1881-0942
Published online by The Japan Institute of Heterocyclic Chemistry
e-Journal
Full Text HTML
Received, 4th September, 2009, Accepted, 13th October, 2009, Published online, 14th October, 2009.
DOI: 10.3987/COM-09-S(S)126
■ Synthesis of Substituted Isoquinolines via Nickel-Catalyzed [2+2+2] Cycloaddition of Alkynes and 3,4-Pyridynes
Toshihiko Iwayama and Yoshihiro Sato*
Graduate School of Pharmaceutical Sciences, Hokkaido University, Kita 12 Nishi 6, Kita-ku, Sapporo, Hokkaido 060-0812, Japan
Abstract
A novel methodology for the synthesis of substituted isoquinolines via a nickel(0)-catalyzed [2+2+2] cycloaddition of 3,4-pyridynes with two molecules of alkyne has been established. In this reaction, it was found that 2-butyn-1,4-diol derivatives and 1,3-diynes are suitable as substrates and that a propargylic oxygen functionality in alkynes is essential for the reactivity and the selectivity of the products.A transition metal-catalyzed [2+2+2] cycloaddition of three components of alkynes is a useful and highly atom-economic way for constructing various aromatic compounds.1 Recently, we have succeeded in developing the first transition metal-catalyzed [2+2+2] cycloaddition of pyridynes with diynes by virtue of a nickel(0) catalyst (Scheme 1).2,3
The reaction of α,ω-diynes 1 and 3,4-pyridyne precursors 2 or diynes 3 having a 3,4-pyridyne precursor moiety in the chain with a zerovalent nickel catalyst in the presence of CsF gave the corresponding tricyclic isoquinolines 4 or tetracyclic isoquinolines 5, respectively, in good yields. In the context, we planned to develop the synthesis of substituted isoquinolines via a [2+2+2] cycloaddition of 3,4-pyridynes with two molecules of alkynes, as shown in Scheme 2. If a [2+2+2] cycloaddition of two molecules of alkyne 6 and 3,4-pyridyne 2’ derived from the precursor 2 in the presence of a fluoride anion proceeds in a similar to that in the previous study shown in Scheme 1, substituted isoquinoline derivative 7 should be produced. By using alkynes as a substrate instead of diynes in the [2+2+2] cycloaddition, it would be more difficult to control the stereochemistry of the product 7 and to prevent the formation of trimers 8 and polymers of alkynes. Thus, this would be a more challenging subject than previously reported cases.
First, a [2+2+2] cycloaddition of 3,4-pyridyne and two molecules of acetylene (6a) was examined (Table 1, run 1). Treatment of 2a with 20 mol% Ni(cod)2 and 40 mol% PPh3 in the presence of CsF (3 equivalents to 2a) under an atmosphere of acetylene (6a, 1 atm) in CH3CN at room temperature gave isoquinoline (7aa) in 40% yield accompanied by the formation of unidentified polymers. Various symmetrical di-substituted alkynes were screened as a coupling partner instead of acetylene under conditions similar to those previously reported, and it was found that 2-butyn-1,4-diol derivative 6b could be applied to the nickel-catalyzed [2+2+2] cycloaddition of 3,4-pyridyne precursor 2a. Thus, the reaction of 6b and 2a (ratio of 6b/2a=1/4) with 20 mol% Ni(cod)2 and 40 mol% PPh3 in the presence of CsF (3 equivalents to 2a) in CH3CN at room temperature gave the corresponding isoquinoline derivative 7ba in 60% yield (run 2). When the ratio of the substrates 6b and 2a was changed from 1/4 to 4/1, the yield of 7ba was improved to 72% (run 3). It was found that loading of the nickel catalyst could be reduced to 10 mol% without lowering of the yield under the conditions, producing 7ba in 73% yield (run 4). On the other hand, the yield of 7ba was decreased to 51% when the catalyst loading was reduced to 5 mol % (run 5). When non-protected 2-butyn-1,4-diol (6c) was used as an alkyne substrate, no desired product 7ca was obtained (run 6). On the other hand, the reaction of 6d, having two THP groups as protecting groups, and 2a under the same conditions gave isoquinoline 7da in 70% yield (run 7). The existence of a carbonyl group in the protecting groups also retarded the reaction, and the reaction of 6e or 6f under the same conditions gave the corresponding product 7ea or 7fa, respectively, in a low yield (runs 8 and 9).
Next, unsymmetrical alkynes having a propargylic oxygen functionality such as 6g-6i (Figure 1) were screened in the [2+2+2] cycloaddition with 2a under the similar conditions, producing none or a trace amount of desired isoquinolines as a mixture of isomers. After various attempts, it was found that 1,3-diyne 6j showed a good reactivity and selectivity.4 Thus, the reaction of 6j and 2a under the optimized conditions gave the corresponding isoquinoline derivative 7ja as a single isomer in 60% yield along with a mixture of unidentified polymers (Table 2, run 1). The stereochemistry of 7ja was unambiguously determined by NOE experiments after assignment of the related protons using 1H NMR, 13C NMR, and HMBC spectra (Figure 2). The reactions of 1,3-diynes 6k-6n under the same conditions also produced isoquinolines 7ka-7na as single isomers (Table 2, runs 2-5), and those stereochemistries were determined in a manner similar to that in the case of 7ja by NOE experiments.
It is noteworthy that all products in these cases were always obtained as a single isomer, with unreacted alkyne parts of the starting 1,3-diynes 6j-6n located at C6- and C7-positions and protected hydroxymethyl moieties located at C5- and C8-positions in the isoquinoline rings of the products 7ja-7na.
We next turned our attention to the scope of 3,4-pyridynes in the [2+2+2] cycloaddition. The results of reactions of 6b with various 3,4-pyridyne precursors 2 are summarized in Table 3.
The reaction of 6b and 2b, which has a pivaloyloxymethyl group at the C2 position of the pyridine ring, under the above-described optimal conditions gave the corresponding isoquinoline 7bb in 80% yield (Table 3, run 1). Electron-donating groups such as a methoxy group on the pyridyne precursor are tolerated in the [2+2+2] cycloaddition, and the reaction of 2c or 2d with 6b produced the corresponding isoquinoline 7bc or 7bd in 81% or 80% yield, respectively. On the other hand, in the case of 2e having an electron-withdrawing group, the yield of the product 7be was lowered to 10%.
The scope of 3,4-pyridynes in the [2+2+2] cycloaddition of 1,3-diynes was also investigated, and the results of the reaction with 6k and 6m are summarized in Table 4. The reaction of 1,3-diyne 6k and the precursor 2b, 2c, or 2d under the optimized conditions produced the corresponding isoquinoline 7kb, 7kc, or 7kd as a single isomer in 72%, 70%, or 74% yield, respectively (runs 1-3). In the case of the reaction of 2b, 2c, and 2d with 6m under the same conditions, the isoquinolines 7mb, 7mc, and 7md were again produced as a single isomer in good yields, respectively (runs 4-6).
The stereochemistries of 7kb, 7kd, 7mb, and 7md were determined in a similar manner by NOE experiments (Figure 3). On the other hand, 7kc and 7mc were successfully converted to 7ka and 7ma via conversion of the C1-methoxy group to a chloride5 (Scheme 3), the spectral data of which were identical to those obtained by the experiments described in Table 2. Notably, in these cases, the same tendency as that in the above-mentioned reactions in Table 2 was observed for the stereochemistry concerned the alkyne moieties and the protected hydroxymethyl moieties in the products.
A possible mechanism of the [2+2+2] cycloaddition of 3,4-pyridyne and two molecules of alkynes is shown in Scheme 4. On the basis of the results described above, it is obvious that the existence of a propargylic oxygen functionality in alkynes 6 is crucial to enhance the reactivity and to control the selectivity of the products in this nickel(0)-catalyzed [2+2+2] cycloaddition with 3,4-pyridiynes.6 Thus, the oxygen at a propargylic position of 6 would initially coordinate to a nickel complex and form the nickel complex I. Then oxidative addition would proceed to produce nickelacyclopentadiene intermediate II in a stereoselective manner. Insertion of 3,4-pyridyne 2’, generated in situ from the precursor 2 and CsF, into the nickel-carbon bond of II would afford seven-membered nickelacycle intermediate IIIa or IIIb, from which reductive elimination would proceed to stereoselectively produce substituted isoquinoline 7.
In summary, we succeeded in developing a novel methodology for the synthesis of substituted isoquinolines via a nickel(0)-catalyzed [2+2+2] cycloaddition of 3,4-pyridynes with two molecules of alkyne. Through screening of various alkynes, 2-butyn-1,4-diol derivatives and 1,3-diynes were found to be suitable as substrates for this [2+2+2] cycloaddition. It was also found that a propargylic oxygen functionality in these alkynes is essential for the reactivity and the selectivity of the products in the reaction. Further studies along this line are in progress.
ACKNOWLEDGEMENTS
Part of this work was supported by Grant-in-Aid for Scientific Research (B) (No. 19390001) from JSPS, Grant-in-Aid for Science Research on Priority Areas (No. 19028001 and 20037002, “Chemistry of Concerto Catalysis”) from MEXT, Japan and by the Takeda Science Foundation, the Uehara Memorial Foundation, and the Akiyama Foundation, which are gratefully acknowledged.
References
1. For recent reviews, see: (a) J. A. Varela and C. Saá, Chem. Rev., 2003, 103, 3787; CrossRef (b) S. Kotha, E. Brahmachary, and K. Lahiri, Eur. J. Org. Chem., 2005, 4741; CrossRef (c) Y. Yamamoto, Curr. Org. Chem., 2005, 9, 503; CrossRef (d) P. R. Chopade and J. Louie, Adv. Synth. Catal., 2006, 348, 2307; CrossRef (e) V. Gandon, C. Aubert, and M. Malacria, Chem. Commun., 2006, 2209; CrossRef (f) K. Tanaka, Synlett, 2007, 1977; CrossRef (g) D. Peña, D. Pérez, and E. Guitián, Chem. Rec., 2007, 7, 326; CrossRef (h) T. Shibata and K. Tsuchikawa, Org. Biomol. Chem., 2008, 1317; CrossRef (i) R. B. Galan and T. Rovis, Angew. Chem. Int. Ed., 2009, 48, 2830. CrossRef
2. T. Iwayama and Y. Sato, Chem. Commun., 2009, 5245. CrossRef
3. For recent examples of nickel(0)-catalyzed [2+2+2] cycloadditions involving arynes as a substrate, see: (a) J.-C. Hsieh, D. K. Rayabarapu, and C.-H. Cheng, Chem. Commun., 2004, 532; CrossRef (b) J.-C. Hsieh and C.-H. Cheng, Chem. Commun., 2005, 2459; CrossRef (c) N. Saito, K. Shiotani, A. Kinbara, and Y. Sato, Chem. Commun., 2009, 4284; CrossRef (d) Z. Qiu and Z. Xie, Angew. Chem. Int. Ed., 2009, 48, 5729. CrossRef
4. For a nickel(0)-catalyzed [2+2+2] cycloaddition of 1,3-diynes and diynes, see: (a) Y. Sato, K. Ohashi, and M. Mori, Tetrahedron Lett., 1999, 40, 5231; CrossRef (b) A. Jeevanandam, R. P. Korivi, I-w. Huang, and C.-H. Cheng, Org. Lett., 2002, 4, 807; CrossRef For a [2+2+2] cycloaddition of 1,3-diynes with a nickel-benzyne complex, see: (c) K. R. Deaton and M. S. Gin, Org. Lett., 2003, 5, 2477; CrossRef (d) K. R. Deaton, C. S. Strouse, and M. S. Gin, Synthesis, 2004, 3084. CrossRef
5. T. R. Kasturi, H. R. Y. Jois, and L. Mathew, Synthesis, 1984, 743. CrossRef
6. The effect of a propargylic oxygen functionality in enhancing the reactivity in the nickel-mediated [2+2+2] cycloaddition has already been reported by Smith, see: P. Bhatarah and E. H. Smith, J. Chem. Soc., Perkin Trans. 1, 1990, 2603. CrossRef