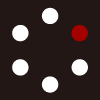
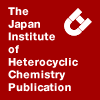
HETEROCYCLES
An International Journal for Reviews and Communications in Heterocyclic ChemistryWeb Edition ISSN: 1881-0942
Published online by The Japan Institute of Heterocyclic Chemistry
e-Journal
Full Text HTML
Received, 13th June, 2009, Accepted, 16th July, 2009, Published online, 21st July, 2009.
DOI: 10.3987/COM-09-S(S)35
■ Phenanthrene-4,5-diylbis(10-methylacridinium) with a Short C+ - - C+ Contact: Preparation, Molecular Structure, Redox Properties, and Electrochromic Interconversion with Dihydropyrene Derivative
Takanori Suzuki,* Yasuyo Yoshimoto, Kazuhisa Wada, Takashi Takeda, Hidetoshi Kawai, and Kenshu Fujiwara
Division of Chemistry, Graduate School of Science, Hokkaido University, Kita 10 Nishi 8, Kita-ku, Sapporo, Hokkaido 060-0810, Japan
Abstract
The title dication (12+) was generated by oxidative bond cleavage of a 4,5-dihydoropyrene derivative (2) with two spiro(10-methylacridan) units. Despite the skewed deformation of the arylene spacer, two cationic chromophores in 12+ are forced to face in a proximity with a very short interatomic separation of 2.98(1) Å between carbenium centers (X-ray), which makes the LUMO level of 1 much lower than that of biphenyl-2,2'-diyl-type dication (32+).4,5-Disubstituted phenanthrenes are sterically challenged molecules.1-3 The bay region substituents make severe steric repulsion even when the molecules deform the rigid phenanthrene core to a skewed geometry. At the same time, the substituents are forced to stay in proximity, thus attaining special properties, such as enhanced Lewis acidity in the modified proton sponge.1 In the course of our study on the redox behavior of isolable dications with a different degree of intramolecular interaction between two cationic centers,4 we have got interested in phenanthrene-4,5-diylbis(diarylcarbenium)s, where the two cationic chromophores are overlapped as in the case of helicenes.5 By considering the very large value of pKR+ for 10-methyl-9-phenylacridinium (11.0),6 we assumed that the title dication (12+) would be obtained as a stable species despite the increased Lewis acidity or electrostatic repulsion. Here we report its successful generation from a 4,5-dihydropyrene derivative (2), the less hindered neutral precursor (Scheme 1). Detailed structural features determined by the X-ray analyses as well as electrochromic behavior are also disclosed.
After the unfruitful trials to introduce two diarylmethyl units to the bay region via 4,5-dilithiophenanthrene, we then planned to obtain 12+ by oxidative bond cleavage of the hexaarylethane-type compound (2). This strategy was postulated based on our previous results on the redox pair of biphenyl-2,2'-diyl(diarylcarbenium) (32+) and 9,10-dihydrophenanthrene derivative (4) (Scheme 2).7 The preparation route for the precursor (2) is shown in Scheme 3.
First, 9,9'-(6,6'-diformylbiphenyl-2,2'-diyl)diacridine (5) was derived from 2,2,6,6-tetrabromobiphenyl8 over 3 steps in 52% yield as we reported previously.9 Treatment of 5 with MeOTf (3.5 eq) in CH2Cl2 at room temperature afforded a TfO− salt of bis(10-methylacridinium) (62+)10 in 89% yield. Upon reduction with excess Zn dust in MeCN at room temperature, 4,5-diformyl-9,10-dihydrophenanthrene derivative (7)10 was obtained. Without purification, it was further reduced under McMurry conditions (TiCl4-Zn in refluxing THF) to furnish dihydropyrene (2)10 in 25% yield over two steps. Stepwise reductive ring-closure from 62+ to 2 via 7 provides a promising route, since McMurry coupling of dialdehyde 5 failed to give fully aromatized phenanthrene core but diol (8).9
The route from 5 shown in Scheme 3 could be used to prepare the analogues of 2 having different substituents on nitrogens. In this way, another dihydropyrene 910 with two spiro(10-propargylacridan) units was also obtained, which gave a suitable single crystal for the X-ray analysis11 (Figure 1). From the ORTEP drawing was confirmed the successful formation of dihydropyrene skeleton in 9 (and in 2) through the double ring-closure processes of biphenyl derivative (5). The C4-C5 bond length is 1.632(4) Å, which is longer than the standard Csp3-Csp3 (1.54 Å) but characteristic to the hexaphenylethane-type compounds [1.61-1.65 Å].12 The condensed aromatic core is nearly planar with a small torsion angle of 3.7(4)° for C3a-C10b-C10c-5a, whereas the out-of-plane deviation of C4 and C5 from the aromatic core allows a large enough torsion angle of 33.5(3)° for C3a-C4-C5-C5a to reduce the "front strain"12,13 between the two spiroacridan units.
The dihydropyrene (2) is stable and colorless crystalline material with strong electron-donating properties as in dihydrophenanthrene (4) (Eox +0.18 V). According to the voltammetric analysis in MeCN (E / V vs SCE, 0.1 M Et4NClO4, Pt electrode, scan rate 100 mV s−1), its oxidation potential (+0.28 V) is close to that of tetrathiafulvalene (Eox +0.31 V) measured under the similar conditions (Figure 2). The oxidation wave is irreversible with the return peak being shifted to the cathode, which was later assigned and confirmed as the reduction peak of the desired dication (12+). The observed separation of redox peaks is the characteristic feature to the "dynamic redox" pairs4 that undergo reversible C-C bond formation/breaking upon electron transfer. Thus, the voltammetric study suggested that 12+ would be formed upon two-electron oxidation of dihydropyrene (2) by cleavage of C4-C5 bond.
Upon treatment of 2 with (4-BrC6H4)3N+•SbCl6− (2 eq) in CH2Cl2, a deep orange powder was obtained in 90% yield. In the NMR spectrum (MeCN-d3), the aromatic resonances assigned to acridine parts are downfield shifted than those for 2. N-Methyl protons appear at 4.27 ppm, which is again downfield shifted from 2.72 ppm for that in 2 (CDCl3). By combining other spectral data, the orange powder thus obtained was proven to be SbCl6− salt of the desired dication (12+),10 which regenerated the starting material quantitatively upon reduction with Zn dust in MeCN. By using (4-BrC6H4)3N+•BF4− (2 eq) as an oxidant, 12+(BF4−)2 was similarly obtained in 90% yield.
The structure was finally verified by the low-temperature X-ray analysis11 of the BF4− salt, from which the detailed geometrical features have been also revealed as follows (Figure 3). The two acridinium chromophores are overlapped nearly in parallel, as expected, with the C9+ -- C9'+ interatomic separation of 2.98(1) Å. Such a contact is one of the shortest ever reported for the carbenium centers,14 and there are only few precedents15 with the non-bonded contact less than 3 Å (sum of vdW radii: 3.40 Å). At the same time, these bulky chromophores at the bay region causes skewing deformation of the phenanthrene core with a bay-region torsion angle of 27.3(7)° for C4-C4a-C4b-C5 to adopt a helical geometry. The chiral space group (hexagonal P65) of 12+(BF4−)2 shows that spontaneous resolution occurs upon crystallization, and each piece of single crystals contains the dication molecule with the same helicity. However, higher symmetry (C2v) of 1H NMR spectrum at room temperature indicates that 12+ undergoes facile helicity inversion in solution as in the case of 4,5-dimethylphenanthrene (ΔG‡ = 16.1 kcal mol−1 at 25 °C).2 Based on the VT-NMR analysis of 12+ in MeCN-d3, the inversion barrier was determined to be only 15.3 kcal mol−1 (at Tc = 10 °C).
It should be the interchromophore interaction due to the helical molecular structure that endows 12+ with strong electron-accepting properties. Its reduction potential (Ered +0.12 V) is much more positive than that for biphenyl-type dication (32+) (Ered −0.27 V),7 showing the low-lying LUMO of 12+ due to electronic interaction of two acridinium parts. In the UV-Vis spectrum, the absorption end of 12+ (λend 550 nm) is red-shifted by 50 nm than that of 32+,7 which can be also rationalized by the low-lying LUMO in 12+. Although the electron-transfer processes should be regarded as irreversible, dication 12+ and neutral precursor 2 can be reversibly interconverted ("dynamic redox" pair). Thus, the continuous changes of UV-Vis spectra were observed upon electrolysis, which was accompanied by vivid color change from colorless (2) to deep orange (12+). Presence of isosbestic points (234, 282, 331 nm) in the spectroelectrogram indicates clean interconversion as well as negligible steady-state concentration of the intermediary cation radical species (Figure 4). This is the successful demonstration of electrochromic behavior16 of 12+ and 2.
In summary, we have generated and characterized helically shaped 9,9'-[phenanthrene-4,5-diyl- bis(10-methylacridinium) (12+), whose properties are determined by electronic interaction between two cationic parts with a very short interatomic contact. The reversible electrochromic interconversion with dihydropyrene (2) is an attracting function of 12+, and we are currently studying its analogues having the different diarylcarbenium moieties and/or a different arylene core.
ACKNOWLEDGEMENTS
This work is supported by MEXT KAKENHI (No. 21350022). The financial support from the Global COE Program by MEXT 'Catalysis as the Basis for Innovation in Materials Science', Hokkaido University is gratefully acknowledged. We thank Prof. Tamotsu Inabe (Department of Chemistry, Faculty of Science, Hokkaido University) for the use of facilities to analyze the X-ray structures. Elemental analyses were done at the Center for Instrumental Analysis of Hokkaido University. Mass spectra were measured by Mr. Kenji Watanabe and Dr. Eri Fukushi at the GC-MS & NMR Laboratory (Faculty of Agriculture, Hokkaido University).
References
1. H. A. Staab and T. Saupe, Angew. Chem., Int. Ed. Engl., 1988, 27, 865; CrossRef S. T. Howard, J. Am. Chem. Soc., 2000, 122, 8238. CrossRef
2. R. N. Armstrong, H. L. Ammon, and J. N. Darnow, J. Am. Chem. Soc., 1987, 109, 2077. CrossRef
3. H. Bock, M. Sievert, and Z. Havlas, Chem. Eur. J., 1998, 4, 677; CrossRef Y. Tanaka, A. Sekita, H. Suzuki, M. Yamashita, T. Oshikawa, T. Yonemitsu, and A. Torii, J. Chem. Soc., Perkin Trans. I, 1998, 2471; CrossRef S. Grimme, I. Pischel, M. Nieger, and F. Vögtle, J. Chem. Soc., Perkin Trans. 2, 1996, 2771; CrossRef K. Müllen, W. Heins, F. -G. Klämer, W. R. Roth, I. Kindermann, O. Adamczak, M. Wette, and J. Lex, Chem. Ber., 1990, 123, 2349; CrossRef A. Mannschreck, E. Gmahl, T. Burgemeister, F. Kastner, and V. Sinnwell, Angew. Chem. Int. Ed., 1998, 27, 270; CrossRef R. Cosmo, T. W. Hambley, and S. Sternhell, J. Org. Chem., 1987, 52, 3119; CrossRef H. Scherübl, U. Fritzsche, and A. Mannschreck, Chem. Ber., 1984, 117, 336; CrossRef D. L. Fields and T. H. Regan, J. Org. Chem., 1971, 36, 2991. CrossRef
4. Review: T. Suzuki, E. Ohta, H. Kawai, K. Fujiwara, and T. Fukushima, Synlett, 2007, 851. CrossRef
5. Reviews: R. Amemiya and M. Yamaguchi, Chem. Rec., 2008, 8, 116; CrossRef A. Rajca, S. Rajca, M. Pink, and M. Miyasaka, Synlett, 2007, 1799; CrossRef I. Starý, I. G. Stará, Z. Alexandrová, P. Sehnal, F. Teplý, D. Šaman, and L. Rulíšek, Pure Appl. Chem., 2006, 78, 495. CrossRef
6. J. W. Bunting and W. G. Meathrel, Can. J. Chem., 1973, 51, 1965. CrossRef
7. T. Suzuki, A. Migita, H. Higuchi, H. Kawai, K. Fujiwara, and T. Tsuji, Tetrahedron Lett., 2003, 44, 6837. CrossRef
8. A. Rajca, A. Safronov, S. Rajca, C. R. Ross, II, and J. J. Stezowski, J. Am. Chem. Soc., 1996, 118, 7272; CrossRef F. R. Leroux, R. Simon, and N. Nicod, Lett. Org. Chem., 2006, 3, 948. CrossRef
9. T. Suzuki, Y. Yoshimoto, T. Takeda, H. Kawai, and K. Fujiwara, Chem. Eur. J., 2009, 15, 2210. CrossRef
10. Selected spectral data are as follows. 12+(SbCl6−)2: mp 254-258 °C; 1H NMR (CD3CN) δ = 8.43 (d, J =7.5 Hz, 2H), 8.30 (s, 2H), 8.35-7.87 (br m, 8H), 7.75 (dd, J =7.5, 7.5 Hz, 2H), 7.32 (dd, J = 8.0, 8.0 Hz, 4H), 7.01 (d, J = 7.5 Hz, 2H), 6.81(br, 4H), 4.27 ppm (s, 6H); IR (KBr): 3016, 3053, 1621, 1607, 1575, 1548, 1529, 1446, 1403, 1371, 1270, 1192, 1172, 1033, 862, 835, 765, 730, 672, 657, 601 cm-1; UV-Vis (MeCN): λmax (ε) = 218 (62200), 250 (86000, sh), 263 (111600), 314 (8350), 369 (15700), 451 nm (8690 м-1 cm-1); 2: mp 289-290 °C; 1H NMR (CDCl3) δ = 7.88 (s, 2H), 7.81 (dd, J = 7.7, 0.7 Hz, 2H), 7.41 (dd, J = 7.7, 7.7 Hz, 2H), 7.24 (dd, J = 7.7, 0.7 Hz, 2H), 6.94 (ddd, J = 8.3, 6.5, 2.3 Hz, 4H), 6.50 (d, J = 8.3 Hz, 4H), 6.21-6.13 (m, 8H), 2.72 ppm (s, 6H); IR (KBr): 3047, 2964, 2925, 2869, 2818, 2806, 1608, 1591, 1475, 1446, 1362, 1326, 1293, 1272, 1166, 1133, 1056, 859, 829, 748, 737, 728, 697, 655 cm-1; UV-Vis (MeCN): λmax (ε) = 211 (71200), 271 (36500), 292 nm (28500 м-1 cm-1); 62+(TfO−)2: mp 150-152 °C; 1H NMR (300 MHz, CD3CN): δ = 10.22 (s, 2H), 8.47 (d, J = 9.2 Hz, 2H), 8.27 (ddd, J = 9.2, 6.6, 1.5 Hz, 2H), 8.25 (dd, J = 7.7, 1.5 Hz, 2 H), 8.09 (d, J = 9.2 Hz, 2 H), 8.02 (ddd, J = 9.2, 6.6, 1.5 Hz, 2 H), 7.89 (dd, J = 8.8, 1.5 Hz, 2 H), 7.74 (dd, J = 7.7, 7.7 Hz, 2 H), 7.65 (ddd, J = 8.8, 6.6, 1.5 Hz, 2 H), 7.25 (dd, J = 7.7, 1.5 Hz, 2 H), 7.12 (ddd, J = 8.8, 6.6, 1.5 Hz, 2 H), 6.19 (d, J = 8.8 Hz, 2 H), 4.58 ppm (s, 6H); IR (KBr): 3107, 3063, 2849, 2745, 1697, 1625, 1610, 1579, 1550, 1461, 1375, 1261, 1224, 1158, 1030, 864, 806, 768, 739, 713, 701, 637, 601, 573, 518 cm-1; 7: 1H NMR (CDCl3) δ = 9.93 (s, 2H), 8.03 (m, 2H), 7.42 (m, 4H), 7.06-6.97 (m, 4H), 6.70 (dd, J = 7.9, 1.3 Hz, 2H), 6.62 (dd, J = 8.4, 1.3 Hz, 2H), 6.48 - 6.46 (m, 4H), 6.23 (ddd, J = 7.9, 7.3, 1.3 Hz, 2H), 5.67 (dd, J = 7.9, 1.4 Hz, 2H), 2.74 ppm (s, 6H); 9: mp 289-290 °C; 1H NMR (CDCl3) δ = 7.90 (s, 2H), 7.83 (d, J = 7.7 Hz, 2H), 7.43 (t, J = 7.7 Hz, 2H), 7.26 (d, J = 7.7 Hz, 2H), 7.00 (td, J = 7.9, 1.4 Hz, 4H), 6.76 (d, J = 7.9 Hz, 4H), 6.31 (t, J = 7.9 Hz, 4H), 6.23 (dd, J = 7.9, 1.4 Hz, 4H), 3.74 (d, J = 2.2 Hz, 4H), 2.26 ppm (t, J = 2.2 Hz, 2H); IR (KBr): 3281, 3052, 2954, 2924, 1606, 1591, 1479, 1461, 1380, 1319, 1292, 1269, 1225, 1168, 1067, 852, 830, 751, 737, 728, 701, 656 cm-1.
11. Crystal data are as follows. 12+(BF4−)2 (CCDC 734864): C42H30B2F8N2, M 736.32, hexagonal, P65, a = 13.440(2), c = 32.401(5) Å, V = 5068.6(1) Å3, Z = 6, Dc 1.447 g cm−3, independent reflection 3844 (all), R = 7.3 %. 9-xylene solvate (CCDC 734865): C54H40N2, M 716.92, orthorhombic, Pbcn, a = 12.165(3), b = 24.397(6), c = 12.566(3) Å, V = 3729(3) Å3, Z = 4, Dc 1.277 g cm−3, independent reflection 4236 (all), R = 8.5%.
12. T. Suzuki, T. Takeda, H. Kawai, and K. Fujiwara, Pure Appl. Chem., 2008, 80, 547. CrossRef
13. W. D. Hounshell, D. A. Dougherty, J. P. Hummel, and K. Mislow, J. Am. Chem. Soc., 1977, 99, 1916. CrossRef
14. H. Kawai, T. Takeda, K. Fujiwara, M. Wakeshima, Y. Hinatsu, and T. Suzuki, Chem. Eur. J., 2008, 14, 5780; CrossRef H. Kawai, T. Takeda, K. Fujiwara, and T. Suzuki, Tetrahedron Lett., 2004, 45, 8289; CrossRef T. Suzuki, S. Tanaka, H. Higuchi, H. Kawai, and K. Fujiwara, Tetrahedron Lett., 2004, 45, 8563; CrossRef H. Wang and F. P. Gabbaï, Angew. Chem. Int. Ed., 2004, 43, 184; CrossRef H. Wang, C. E. Webster, L. M. Pérez, M. B. Hall, and F. P. Gabbaï, J. Am. Chem. Soc., 2004, 126, 8189. CrossRef
15. H. -J. Hasselbach, C. Krieger, M. Decker, and H. A. Staab, Liebigs Ann. Chem., 1986, 765; CrossRef H. Kawai, T. Takeda, K. Fujiwara, and T. Suzuki, Tetrahedron Lett., 2004, 45, 8289. CrossRef
16. P. M. S. Monk, R. J. Mortimer, and D. R. Rosseinsky, 'Electrochromism and Electrochromic Devices', Cambridge Univ. Press, Cambridge, 2007.