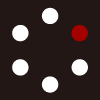
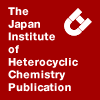
HETEROCYCLES
An International Journal for Reviews and Communications in Heterocyclic ChemistryWeb Edition ISSN: 1881-0942
Published online by The Japan Institute of Heterocyclic Chemistry
e-Journal
Full Text HTML
Received, 31st July, 2009, Accepted, 31st August, 2009, Published online, 3rd September, 2009.
DOI: 10.3987/COM-09-S(S)93
■ Synthesis of the ABC Ring Fragment of Brevisin, a New Dinoflagellate Polycyclic Ether
Naohito Ohtani, Ryosuke Tsutsumi, Takefumi Kuranaga, Tomohiro Shirai, Jeffrey L. C. Wright, Daniel G. Baden, Masayuki Satake,* and Kazuo Tachibana*
Department of Chemistry, Graduate School of Science, University of Tokyo, 7-3-1 Hongo, Bunkyo-ku, Tokyo 113-0033, Japan
Abstract
A polycyclic ether, brevisin was isolated from the red-tide dinoflagellate Karenia brevis. Its unique skeletal structure consists of two separate tricyclic ether assemblies connected by a methylene bridge. The ABC ring fragment of brevisin was synthesized via Suzuki–Miyaura cross coupling toward a total synthesis of brevisin.A polycyclic ether, brevisin (1)1 was isolated from the red tide dinoflagellate Karenia brevis which simultaneously produces a range of ladder-frame polyethers, including brevetoxins,2-4 brevenal and its acetal,5 and a cyclic ether amide, brevisamide.6 The structure of 1 is characterized by two tricyclic ether ring assemblies linked by a methylene group. One of the assemblies contains a conjugated aldehyde side chain which has been found in brevenal and brevisamide. The interrupted polycyclic ether skeleton of the molecule is quite unique. Common structural features for representative marine polycyclic ethers such as the brevetoxins, ciguatoxins7 and yessotoxins8 are contiguous fused ether ring assemblies containing middle size (from 7 to 9-membered) ether rings at the center of their polycyclic ether skeletons.9
Interestingly, even though the skeletal structure of 1 is divided into two ether ring assemblies by the methylene, 1 inhibited the binding of 42-dihydrobrevetoxin B (PbTx-3) to the VSSC.1 Stereochemical information of 1 is essential in order to elucidate the relationship between its unique structure and biological activity. However, the relative stereochemistry of the ABC and DEF ring assemblies could be only determined separately due to the shortage of natural brevisin which hampered determination of the stereochemical correlation between the ether ring assemblies and the absolute configuration by NMR methods. Therefore we have begun synthesis of 1 to determine the complete stereostructure of this unusual polyether. Including our group, numerous efforts have been directed toward the synthesis of marine polycyclic ethers using various approaches.10 Our synthetic strategy based on Suzuki–Miyaura cross coupling11 has led to convergent synthesis of ether ring skeletons of polycyclic ethers12 and the recent successful synthesis of the related metabolite brevisamide.13 Herein we report synthesis of the ABC-ring fragment 2 leading toward the total synthesis of 1 via Suzuki–Miyaura cross coupling.
Based on the structural similarity of the dienal side chain and the 6-membered ether ring in 1, brevenal, and brevisin, we synthesized the ABC-ring fragment with an 8S configuration. Our synthetic strategy is summarized in Scheme 1. The A-ring exo-olefin 3 was prepared from 3-benzyloxypropan-1-ol (5) and the C-ring ketene acetal phosphate 4 was prepared from 2-deoxy-D-ribose by reported procedures.15 The A-ring exo-olefin 3 and the C-ring ketene acetal phosphate 4 could be linked by a Suzuki–Miyaura cross coupling and subsequent B-ring construction by mixed thioacetalization/methylation to yield the ABC-ring fragment 2.
The synthesis of the A-ring exo-olefin 3 began with 3-benzyloxypropan-1-ol (5), which was oxidized with TEMPO and PhI(OAc)2 to generate aldehyde 6.16 Optically active homoallylic alcohol 7 was streoselectively prepared from 6 by the following procedures. Reagent-controlled enantioselective crotylation of aldehyde using (Z)-2-butene and (+)-β-methoxydiisopinocampheylborane17 gave the desired alcohol 7 in 56% yield (90% ee). Determination of the absolute configuration of alcohol 7 was confirmed by the modified Mosher method.18 The alcohol was esterified with acryloyl chloride and i-Pr2NEt (DIPEA) to furnish acrylate 8 in 92% yield. Treatment of 8 with the second-generation Grubbs’ catalyst (Grubbs’ II)19 resulted in successful closure of α,β-unsaturated six-membered lactone 9 in 94% yield, and was subsequently oxidized to diol 10 with OsO4 and NMO in 88% yield. After protection of the diol with TESOTf to give lactone 11, the stereostructure of this key intermediate was assigned by NOE correlations and 3JH,H coupling constants. NOE correlations between the C-9 methyl and H-11, and the C-9 methyl and H-10 suggested an axial orientation of the C-9 methyl and H-11, and an equatorial orientation of H-10. An NOE correlation and small coupling constant (J =3 Hz) between H-9 and H-8 confirmed an axial direction of H-8. The A-ring exo-olefin 3 was generated by a Petasis–Tebbe reaction with Cp2TiMe2 at 65 °C in 92% yield (Scheme 2).20
The known lactone 12 was prepared from 2-deoxy-D-ribose in seven steps.15 Enolization of this lactone with KHMDS in the presence of (PhO)2P(O)Cl generated the C-ring ketene acetal phosphate 4 in 96% yield. Stereoselective hydroboration of the A-ring exo-olefin 3 with 9-BBN-H produced an alkylborane, which was reacted in situ with the C-ring ketene acetal phosphate 4 in the presence of aqueous Cs2CO3 and a catalytic amount of PdCl2(dppf), to give rise to the cross coupling product 13 in 66% yield from 4. A diastereomer could not be detected because of its trace amount. Stereoselective hydroboration-oxidation of the coupling product 13 furnished alcohol 14 in 77% yield. An NOE correlation between H-14 and the C-19 methyl unambiguously indicated the stereochemistry of 14 as shown in Scheme 3. Oxidation of the resultant hydroxy group with TPAP/NMO led to ketone 15 in 93% yield. Treatment of 15 with EtSH and Zn(OTf)2 led to removal of the TES group and formation of mixed thioacetal, and following acetylation yielded mixed thioacetals 16 (29%) and 17 (48%) for the two steps. Introduction of the C-15 methyl group in the triacetylated thioacetal 16 was achieved in a one pot manner with mCPBA and then AlMe3 giving rise to triether ring assembly 2 in 98% yield (Scheme 3).21,22 The stereochemistry of 2 was confirmed by NOE correlations H-11/the C-15 methyl and H-12/H-8 and H-14.
Although the 1H NMR chemical shifts from H-8 to H-18 of the ABC-ring fragment 2 are slightly different from those of the corresponding domain of tetraacetylated brevisin probably due to the absence of a dienal structure and the DEF ring assembly, the coupling constants of 2 agreed well with those of 1.
In summary, the ABC-ring fragment 2 of brevisin was synthesized in a stereocontrolled manner from commercially available materials, 3-benzyloxypropan-1-ol and 2-deoxy-D-ribose. Olefin metathesis was highly effective for construction of the A-ring. The α,β-unsaturated lactone 9 was constructed in only two steps from homoallylic alcohol 7. Assembly of the A-ring exo-olefin 3 and the C-ring ketene acetal phosphate 4 was accomplished by an effective Suzuki–Miyaura coupling reaction. Further study toward the total synthesis of brevisin is now in progress.
ACKNOWLEDGEMENTS
This work was financially supported by KAKENHI (No. 2061102) and the Global COE Program for Chemistry Innovation, the University of Tokyo. T.K. is grateful for a SUNBOR Scholarship. The natural brevisin was isolated from the cultures maintained under grants P01 ES 10594 (NIEHS, DHHS) and MARBIONC, Marine Biotechnology in North Carolina. J.L.C.W. acknowledges funding from NOAA-ECOHAB (MML-106390A) and NCDHHS (01515-04).
References
1. M. Satake, A. Campbell, R. M. Van Wagoner, A. J. Bourdelais, H. Jacocks, D. G. Baden, and J. L. C. Wright, J. Org. Chem., 2009, 74, 989. CrossRef
2. Y.-Y. Lin, M. Risk, S. M. Ray, D. Van Engen, J. Clardy, J. Golik, J. C. James, and K. Nakanishi, J. Am. Chem. Soc., 1981, 103, 6773. CrossRef
3. Y. Shimizu, H. N. Chou, H. Bando, G. Van Duyne, and J. Clardy, J. Am. Chem. Soc., 1986, 108, 514. CrossRef
4. D. G. Baden, A. J. Bourdelais, H. Jacocks, S. Michelliza, and J. Naar, Environ. Health Perspect., 2005, 113, 621.
5. A. J. Bourdelais, H. M. Jacocks, J. L. C. Wright, P. M. Jr. Bigwarfe, and D. G. Baden, J. Nat. Prod., 2005, 68, 2. CrossRef
6. M. Satake, A. J. Bourdelais, R. M. Van Wagoner, D. G. Baden, and J. L. C. Wright, Org. Lett., 2008, 10, 3465. CrossRef
7. M. Murata, A. M. Legrand, Y. Ishibashi, M. Fukui, and T. Yasumoto, J. Am. Chem. Soc., 1990, 112, 4380. CrossRef
8. M. Murata, M. Kumagai, J. S. Lee, and T. Yasumoto, Tetrahedron Lett., 1979, 28, 5869. CrossRef
9. T. Yasumoto and M. Murata, Chem. Rev., 1993, 93, 1897. CrossRef
10. Recent reviews for synthesis of polycyclic ethers. M. Inoue, Chem. Rev., 2005, 105, 4379; CrossRef T. Nakata, Chem. Rev., 2005, 105, 4314; CrossRef H. Fuwa and M. Sasaki, Curr. Opin. Drug Discovery Dev., 2007, 10, 784; K. C. Nicolaou, M. O. Frederick, and R. J. Aversa, Angew. Chem. Int. Ed., 2008, 47, 7182. CrossRef
11. A. Suzuki and N. Miyaura, Chem. Rev., 1995, 95, 2457; CrossRef S. R. Chemler, D. Trauner, and S. J. Danishefsky, Angew. Chem. Int. Ed., 2001, 40, 4544; CrossRef M. Sasaki and H. Fuwa, Nat. Prod. Rep., 2008, 25, 401. CrossRef
12. H. Fuwa, N. Kainuma, K. Tachibana, and M. Sasaki, J. Am. Chem. Soc., 2002, 124, 14983; CrossRef H. Fuwa, S. Fujikawa, K. Tachibana, H. Takakura, and M. Sasaki, Tetrahedron Lett., 2004, 45, 4795; CrossRef M. Sasaki and K. Tachibana, Tetrahedron Lett., 2007, 48, 3181. CrossRef
13. T. Kuranaga, T. Shirai, D. G. Baden, J. L. C. Wright, M. Satake, and K. Tachibana, Org. Lett., 2009, 11, 217. CrossRef
14. The carbon numbering of all compounds in this paper corresponds to that of brevisin.
15. I. Kadota, C. Kadowaki, C.-H. Park, H. Takamura, K. Sato, P. W. H. Chan, S. Thorand, and Y. Yamamoto, Tetrahedron, 2002, 58, 1799. CrossRef
16. A. D. Mico, R. Margarita, L. Parlanti, A. Vescovi, and G. Piancatelli, J. Org. Chem., 1997, 62, 6974. CrossRef
17. H. C. Brown and K. S. Bhat, J. Am. Chem. Soc., 1986, 108, 5919; CrossRef U. S. Racherla and H. C. Brown, J. Org. Chem., 1991, 56, 401. CrossRef
18. I. Otani, T. Kusumi, Y. Kashman, and H. Kakisawa, J. Am. Chem. Soc., 1991, 113, 4092. CrossRef
19. M. Scholl, S. Ding, C. W. Lee, and R. H. Grubbs, Org. Lett., 1999, 1, 953. CrossRef
20. N. A. Petasis and E. I. Bzowej, J. Am. Chem. Soc., 1990, 112, 6392. CrossRef
21. K. C. Nicolaou, M. F. Duggan, and C.-K. Hwang, J. Am. Chem. Soc., 1986, 108, 2468; CrossRef K. C. Nicolaou, C. V. C. Prasad, C.-K. Hwang, M. E. Duggan, and C. A. Veale, J. Am. Chem. Soc., 1989, 111, 5321. CrossRef
22. Physicochemical data of 2: [α]D29 –15 (c 0.13, CHCl3); 1H-NMR (500 MHz, CD3OD) δ 7.36 (5H, m, Ph), 5.16 (1H, br dd, J=2.1, 5.4 Hz), 4.92 (1H, m), 4.53 (1H, d, J=12.2 Hz), 4.47 (1H, d, J=12.2 Hz), 3.95 (1H, m), 3.94 (1H, d, J=11.4 Hz), 3.89 (1H, d, J=11.4 Hz), 3.65 (1H, dd, J=5.1, 12.2 Hz), 3.57 (1H, m), 3.55 (1H, m), 3.46 (1H, dd, J=2.9, 11.5 Hz), 3.46 (1H, ddd, J=3.8, 13.5, 13.5 Hz), 2.16 (3H, s), 2.08 (3H, s), 2.07 (3H, s), 1.89 (1H, m), 1.87 (1H, m), 1.85 (2H, m), 1.73 (1H, ddd, J=4.2, 9.2, 9.2 Hz), 1.69 (1H, dd, J=4.6, 10.9 Hz), 1.58 (1H, m), 1.51 (1H, m), 1.49 (1H, ddd, J=13, 13, 13 Hz), 1.22 (3H, s), 1.19 (3H, s), 1.01 (3H, d, J=7.6 Hz); 13C-NMR (100 MHz, CD3OD) δ 172.2, 172.1, 171.6, 139.9, 129.3, 129.0, 128.7, 80.3, 79.0, 75.9, 74.9, 73.9, 73.3, 73.1, 72.9, 69.5, 68.7, 67.7, 39.3, 36.4, 35.0, 33.2, 30.8, 24.4, 21.2, 20.8, 18.5, 15.7, 11.3, HRMS (FAB), calcd for C31H44O10Na 599.2827 (M+Na+), found 599.2823.