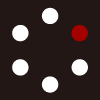
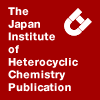
HETEROCYCLES
An International Journal for Reviews and Communications in Heterocyclic ChemistryWeb Edition ISSN: 1881-0942
Published online by The Japan Institute of Heterocyclic Chemistry
e-Journal
Full Text HTML
Received, 22nd July, 2009, Accepted, 31st August, 2009, Published online, 1st September, 2009.
DOI: 10.3987/COM-09-S(S)64
■ Preparation and Ferroelectric Properties of New Chiral Liquid Crystalline Organic Radical Compounds
Naohiko Ikuma, Katsuaki Suzuki, Yoshiaki Uchida, Rui Tamura,* Yoshio Aoki, and Hiroyuki Nohira
Graduate School of Human and Environmental Studies, Kyoto University, Kyoto 606-8501, Japan
Abstract
We have synthesized new chiral liquid crystalline (LC) organic radical compounds 2 and 3, which are the derivatives of the previously reported compounds 1 containing a chiral cyclic nitroxide unit and an ester group in the mesogen core, and their ferroelectric properties have been studied. The enantiomerically enriched (2S,5S)-2 and (2S,5S)-3 showed an SmC* phase for which their ferroelectric properties such as spontaneous polarization (Ps) and tilt angle (θ) were compared with those of (2S,5S)-1.INTRODUCTION
Recently, we have shown that a 2,2,5,5-tetrasubstituted pyrrolidin-1-yloxy (PROXYL) structure is stable enough for repeated heating and cooling cycles below 150°C in the air and thereby this radical unit can serve as the excellent source of the magnetic spin (S = 1/2) and spontaneous polarization (Ps) for elaborating paramagnetic as well as ferroelectric all-organic liquid crystals (LCs), which can combine the optical and electric properties of conventional ferroelectric LCs (FLCs) with the magnetic properties of paramagnetic compounds.1-9 For example, it is quite interesting to know whether magneto-electric effects can be observed in the FLC state of paramagnetic materials. The magneto-electric effects are well known to arise from the intra- and intermolecular interactions between magnetic and electric dipole moments and to be observed in ferroelectric rare earth metal manganite crystals such as YMnO3, TbMnO3, and HoMnO3 with magnetic order at low temperatures.10-12 Experimentally, the effects can be detected as the influence of a magnetic (electric) field on the polarization (magnetization) of a material and vice versa.13-15
In this context, we successfully obtained the prototypic all-organic chiral LC radical compounds 1 which have a PROXYL group with a large electric dipole moments (3 Debye) in the mesogen core as well as a polar ester group (1.8 Debye) used very often for the preparation of conventional FLCs.16 Indeed, (2S,5S)-1 (n=11–15) showing an SmC* phase exhibited ferroelectricity in a thin sandwich cell (4 µm thickness); the highest Ps value of 24 nC cm–2 was recorded for (2S,5S)-1 (n=13).2,3
In order to improve the Ps value which should become a crucial factor for observing magneto-electric effects in the paramagnetic FLCs, we have designed compounds (2S,5S)-2 and (2S,5S)-3 with an additional benzene ring on either 2 or 5 position of the PROXYL ring, compared to (2S,5S)-1 (Chart 1). This structural modification is expected to result in the strong intra-layer interactions in the SmC* phase and hence to increase the overall Ps value.17 Here we report the synthesis, LC characterization and ferroelectric properties of (2S,5S)-2 and (2S,5S)-3.
RESULTS AND DISCUSSION
Synthesis of (2S,5S)-2 and (2S,5S)-3. (2S,5S)-2 and (2S,5S)-3 were synthesized with high stereoselectivity by repeating the addition of an appropriate Grignard reagent to nitrones [4 (ca. 90% ee) and 5] and the subsequent oxidation twice, followed by benzoylation of the phenolic hydroxy group after desilylation, similarly to the synthesis of (2S,5S)-1 (Scheme 1).1-3,18-23
Characterization of LC phases. The phase transition behavior of (2S,5S)-2 and (2S,5S)-3 was characterized by DSC, POM, and XRD analyses, which were measured below 160°C because these compounds instantly decompose in the isotropic phase above 160°C (Table 1, Figure 1 and 2).
As expected, (2S,5S)-2 and (2S,5S)-3 showed stable LC phases over wide temperature ranges and at higher temperatures than (2S,5S)-1 due most likely to the stronger inter-layer interactions.17 Similarly to the case of (2S,5S)-1, (2S,5S)-2 with C12 to C15 chains and (2S,5S)-3 with C13 and C14 chains exhibited SmC* and N* phases, with respective broken fan-shaped and oily-streak textures observed on the cooling run (Table 1, Figure 1 and 2). Variable temperature XRD analysis verified the existence of the SmC* and N* phases (Figure 3).
Figure 2. Polarized optical micrographs showing (a) oily-streaks texture (N*) under random conditions at 158°C and (b) broken fan-shaped texture (SmC*) in a thin sandwich cell (4 mm) under homogeneous planar boundary conditions at 130°C for (2S,5S)-2b, and (c) oily-streaks texture (N*) under random conditions at 160°C and (d) broken fan-shaped texture (SmC*) in a thin sandwich cell (4 µm) under homogeneous planar boundary conditions at 150°C for (2S,5S)-3b.
Ferroelectric properties. The magnitude of Ps and the optical and calculated tilt angles [θ and cos–1(d/l)] of (2S,5S)-2 and (2S,5S)-3 are summarized in Figures 4 and 5 and Table 2. On the cooling run from the N*-to-SmC* transition temperature, the Ps values rapidly increased (Figure 4). On the whole, the Ps values of (2S,5S)-2 were larger than those of the corresponding (2S,5S)-1 and (2S,5S)-3; (2S,5S)-2d showed the highest Ps(–10°) value of 32 nC cm–2 (Figure 4a and Table 2). Such high Ps values observed for (2S,5S)-2 can be interpreted in terms of the increased intra-layer interactions.17 The tilt angles of (2S,5S)-2 and (2S,5S)-3 were too large to measure the optical response time (Figure 5 and Table 2); only a bright-dark-bright or dark-bright-dark texture change was observed upon switching the polarity of the applied electric field.
Moreover, in contrast to the case of (2S,5S)-1, we observed the relaxation of the ferroelectric state of (2S,5S)-2a and (2S,5S)-2b in the same type of FLC cell, i.e., POM texture change by turning off the electric fields (Figure 6). At the same time, the textures in the two field-free states of (2S,5S)-2a or (2S,5S)-2b considerably differed from each other, suggesting the short-pitch SmC* nature and the incomplete formation of a helical superstructure in the FLC cell used (Figures 6b and d).
In summary, we could obtain new chiral organic radical compounds with strong inter-layer interactions which showed FLC properties at high temperatures and over wide temperature ranges. Above all, (2S,5S)-2d showed the highest Ps(–10°) value of 32 nC cm–2, which was larger than that (24 nC cm–2) of (2S,5S)-1 (n=13) previously reported.2,3
EXPERIMENTAL
General. Transition temperatures refer to the peak top of each transition curve by DSC analysis, which was performed at a scanning rate of 5 °C min–1. Enantiomeric excess (ee) were determined by HPLC analysis using a chiral stationary phase column (Daicel Chiralpak AD, 0.46 x 25 cm), a mixture of hexane and 2-PrOH (9:1) as the mobile phase at a flow rate of 1.0 mL min–1, and a UV-vis spectrometer (254 nm) as the detector. Ps was measured by the triangular wave method at a frequency of 20 Hz in a thin sandwich cell (EHC Co., Japan; thickness of 4 µm) coated with ITO electrodes and covered with antiparallel-rubbed polyimide films in an electric field of 10 V peak to peak. Tilt angles were measured by polarized optical microscopy (POM). Their g values and hyperfine coupling constants (aN) were determined by electron paramagnetic resonance (EPR) spectroscopy in THF at 25 ºC. The variable temperature X-ray diffraction (XRD) patterns were recorded at a continuous scanning rate of 2° 2θ min–1 at a heating and cooling rate of 4 °C min–1 using CuKα radiation (40 kV, 20 mA), with the intensity of the diffracted X-rays being collected at intervals of 0.02° 2θ.
General synthetic procedure for (2S,5S)-2 and (2S,5S)-3. Enantiomerically enriched nitrone (R)-4 (ca. 90% ee) was prepared according to the published procedure.18,19 The nitrone (R)-4 (0.452 g, 4.0 mmol) was reacted with the first Grignard reagent (8.0 mmol) in THF (5 mL) at –78 ºC. The reaction temperature was slowly raised to 25 ºC. After being stirred overnight, the reaction mixture was poured into saturated aqueous NH4Cl solution (50 mL), followed by extraction with CH2Cl2 (2 x 50 mL) and concentration in vacuo. The residue was dissolved in MeOH (20 mL) and oxidized by treatment with Cu(OAc)2⋅H2O(0.13 g), conc. NH3 solution (1.1 mL), and O2 bubbling until dark blue color developed in solution. The solution was evapolated in vacuo, and the residue extracted by CHCl3 (50 mL). The organic phase was washed with saturated aqueous NaHCO3 solution (50 mL), dried over MgSO4, and concentrated in vacuo to give the crude nitrone (S)-5. The crude (S)-5 was reacted with the second Grignard reagent (8.0 mmol) and the resulting crude addition product was oxidized by the same procedure. The crude (2S,5S)-6 was roughly purified by column chromatography on silica gel (hexane:CH2Cl2:Et2O 6/4/0~6/3/1) to remove mainly the less polar impurities. Then to this crude (2S,5S)-6 dissolved in THF (20 mL) was added a THF solution of Bu4NF (1 M solution, 1.0 mL) at 0 ºC. After being stirred at 0 °C for 20 min, the reaction mixture was poured into saturated aqueous NH4Cl solution (30 mL), followed by extraction with CH2Cl2 (2 x 50 mL), drying over MgSO4, and concentration in vacuo. The crude product was purified by column chromatography on silica gel (hexane:CH2Cl2:Et2O 6/3/1~5/3/2) to give the pure desilylated product of (2S,5S)-6 as yellow solid in ca. 10% yield from (R)-4.
To a mixture of the desilylated product (0.3 mmol) and Et3N (0.9 mL) in THF (10 mL) was added an appropriate p-alkoxybenzoyl chloride (0.9 mmol) in THF (3 mL) at 0°C. The reaction mixture was slowly warmed to 25 °C and stirred for 40 h. Then the mixture was poured into a saturated aqueous NaHCO3 solution (20 mL) and the aqueous mixture was extracted with Et2O (2 x 50 mL). The combined organic phase was dried over MgSO4 and evaporated in vacuo. Column chromatography on silica gel (hexane:CH2Cl2:Et2O 8/2/0~7/2/1) of the residue gave pure (2S,5S)-2 or (2S,5S)-3 as yellow solid in 70-80% yield from (2S,5S)-6.
(2S,5S)-2a: [α]D24 –71.49 (89.3% ee, c 1.001, THF). EPR (THF): g = 2.0057, aN = 1.33 mT. IR (KBr): 2923, 2850, 1734, 1607, 1507, 1395, 1254, 1164, 1071, 813, 764. Anal. Calcd for C55H77NO5: C, 79.47; H, 9.22; N, 1.69. Found: C, 79.39; H, 9.44; N, 1.70.
(2S,5S)-2b: [α]D25 –72.91 (91.0% ee, c 0.982, THF). EPR (THF): g = 2.0060, aN = 1.34 mT. IR (KBr): 2849, 1735, 1604, 1507, 1395, 1250, 1164, 1078, 820, 765. Anal. Calcd for C57H81NO5: C, 79.68; H, 9.38; N, 1.63. Found: C, 79.69; H, 9.41; N, 1.60.
(2S,5S)-2c: [α]D25 –70.40 (90.9% ee, c 0.991, THF). EPR (THF): g = 2.0058, aN = 1.33 mT. IR (KBr): 2915, 1731, 1607, 1498, 1371, 1254, 1201, 1165, 1077, 819, 764. Anal. Calcd for C59H85NO5: C, 79.86; H, 9.54; N, 1.58. Found: C, 79.90; H, 9.60; N, 1.55.
(2S,5S)-2d: [α]D25 –67.13 (90.8% ee, c 0.984, THF). EPR (THF): g = 2.0059, aN = 1.33 mT. IR (KBr): 2922, 2851, 1730, 1606, 1511, 1371, 1254, 1201, 1164, 1977, 823, 764. Anal. Calcd for C61H89NO5: C, 80.04; H, 9.69; N, 1.53. Found: C, 80.23; H, 9.52; N, 1.57.
(2S,5S)-3a: [α]D25 –71.27 (91.2% ee, c 1.006, THF). EPR (THF): g = 2.0055, aN = 1.33 mT. IR (KBr): 2917, 2853, 1731, 1605, 1509, 1372, 1256, 1206, 1169, 1079, 850, 767. Anal. Calcd for C57H81NO5: C, 79.68; H, 9.38; N, 1.63. Found: C, 79.44; H, 9.39; N, 1.65.
(2S,5S)-3b: [α]D25 –67.84 (89.0% ee, c 1.003, THF). EPR (THF): g = 2.0056, aN = 1.32 mT. IR (KBr): 2921, 2851, 1730, 1605, 1510, 1372, 1255, 1206, 1169, 1079, 876, 767. Anal. Calcd for C59H85NO5: C, 79.86; H, 9.54; N, 1.58. Found: C, 79.76; H, 9.53; N, 1.63.
References
1. N. Ikuma, R. Tamura, S. Shimono, N. Kawame, O. Tamada, N. Sakai, J. Yamauchi, and Y. Yamamoto, Angew. Chem. Int. Ed., 2004, 43, 3677. CrossRef
2. N. Ikuma, R. Tamura, S. Shimono, Y. Uchida, K. Masaki, J. Yamauchi, Y. Aoki, and H. Nohira, Adv. Mater., 2006, 18, 477. CrossRef
3. N. Ikuma, R. Tamura, S. Shimono, K. Masaki, Y. Uchida, J. Yamauchi, Y. Aoki, and H. Nohira, Ferroelectrics, 2006, 343, 119. CrossRef
4. Y. Uchida, R. Tamura, N. Ikuma, J. Yamauchi, Y. Aoki, and H. Nohira, Mol. Cryst. Liq. Cryst., 2007, 479, 213. CrossRef
5. Y. Uchida, R. Tamura, N. Ikuma, J. Yamauchi, Y. Aoki, and H. Nohira, Ferroelectrics, 2008, 365, 158. CrossRef
6. Y. Uchida, N. Ikuma, R. Tamura, S. Shimono, Y. Noda, J. Yamauchi, Y. Aoki, and H. Nohira, J. Mater. Chem., 2008, 18, 2950. CrossRef
7. R. Tamura, N. Ikuma, and Y. Uchida, J. Mater. Chem., 2008, 18, 2872. CrossRef
8. G. I. Likhtenshtein, J. Yamauchi, S. Nakatsuji, A. I. Smirnov, and R. Tamura, ‘Nitroxides: Applications in Chemistry, Biomedicine, and Materials Science,’ Wiley-VCH, Weinheim, 2008.
9. R. Tamura, N. Ikuma, and S. Shimono, In ‘Soft Nanomaterials, Vol. 1,’ ed. by H. S. Nalwa, American Scientific Publishers, California, 2009, pp. 257-277.
10. M. Fiebig, T. Lottermoser, D. Fröhlich, A.V. Goltsev, and R. V. Pisarev, Nature, 2002, 419, 818. CrossRef
11. T. Kimura, T. Got, G. Shintani, K. Ishizuka, T. Arima, and Y. Tokura, Nature, 2003, 426, 55. CrossRef
12. T. Lottermoser, T. Lonkai, U. Amann, D. Hohlwein, J. Ihringer, and M. Fiebig, Nature, 2004, 430, 541. CrossRef
13. C. Falser, G. H. Fecher, and B. Balke, Angew. Chem. Int. Ed., 2007, 46, 668. CrossRef
14. C. N. Rao and C. R. Serrano, J. Mater. Chem., 2007, 17, 4931. CrossRef
15. W. Erenstein, N. D. Mathur, and J. F. Scott, Nature, 2006, 442, 759. CrossRef
16. D. M. Walba, Top. Stereochem., 2003, 24, 457. CrossRef
17. V. Bezborodov, V. Lapanik, G. Sasnouski, and W. Haase, Ferroelectrics, 2006, 343, 49. CrossRef
18. J. Einhorn, C. Einhorn, F. Ratajczak, I. Gautier-Lunear, and J.-L. Pierre, J. Org. Chem., 1997, 62, 9385. CrossRef
19. R. P. Short, R. M. Kennedy, and S. Masamune, J. Org. Chem., 1989, 54, 1755. CrossRef
20. N. Benfaremo, M. Steenbock, M. Klapper, K. Müller, V. Enkelmann, and K. Cabrera, Liebigs Ann. Chem., 1996, 1413.
21. N. Ikuma, R. Tamura, S. Shimono, N. Kawame, O. Tamada, N. Sakai, J. Yamauchi, and Y. Yamamoto, Mendeleev Commun., 2003, 109. CrossRef
22. N. Ikuma, R. Tamura, S. Shimono, N. Kawame, O. Tamada, N. Sakai, Y. Yamamoto, and J. Yamauchi, Mol. Cryst. Liq. Cryst., 2005, 440, 23. CrossRef
23. N. Ikuma, H. Tsue, N. Tsue, S. Shimono, Y. Uchida, K. Masaki, N. Matsuoka, and R. Tamura, Org. Lett., 2005, 7, 1797. CrossRef