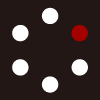
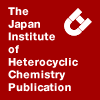
HETEROCYCLES
An International Journal for Reviews and Communications in Heterocyclic ChemistryWeb Edition ISSN: 1881-0942
Published online by The Japan Institute of Heterocyclic Chemistry
e-Journal
Full Text HTML
Received, 31st July, 2009, Accepted, 31st August, 2009, Published online, 3rd September, 2009.
DOI: 10.3987/COM-09-S(S)92
■ Preparation and Photochemical Properties of [2]Rotaxanes Containing an Aniline Moiety Encapsulated by Crown Ethers
Yuji Tokunaga,* Satoshi Nakashima, Takuya Iwamoto, Kei Gambayashi, Kenji Hisada, and Tomonori Hoshi
Department of Materials Science and Engineering, Faculty of Engineering, Fukui University, Bunkyo, Fukui 910-8507, Japan
Abstract
This paper describes preparation of rotaxanes having an aniline moiety in the axle and crown ethers as wheels through an imine formation reaction and hydrogen-bond-guided self-assembly. UV-vis and emission measurements of the rotaxanes show that both absorption and fluorescence bands shift to longer wavelengths as compared to those of the unencapsulated axle component.Polyanilines are remarkable conjugated polymers with application in electrochromic devices, sensors, electromechanical actuators, and rechargeable batteries, and as a hole-transporting material in organic LEDs.1 Recently, it has been reported that the stability and other chemical properties of redox-centers can be improved by rotaxane encapsulation.2 A noticeable stabilization (improvement of oxidation-proof property) of the phenylenediamine subunits was observed in the inclusion complex of the former and cyclobis(paraquat-p-phenylene).3 A radical cation of oligo- and polyaniline axle encapsulated by a cucurbituril is strongly stabilized4 and the chemical oxidation of polyaniline encapsulated in cyclodextrin is effectively inhibited, e.g., doping of polyaniline by iodine.5
Crown ethers bind secondary ammonium ions allowing the construction of the pseudorotaxanes through hydrogen bonding between the two components,6 and several crown ethers with different ring sizes and functionalities have been shown to form pseudorotaxanes.7 Because these modifications effectively change the internal environment of the rings, the way aromatic functionalities in the crown ethers may influence the chemical properties of the axle part of the rotaxane have to be considered. In this study, we investigated the synthesis of rotaxanes having aniline moieties in the axle and a variety of crown ethers as wheels. Furthermore, we studied how changing the aromatic functionality of the crown ether alters the photochemical properties of the aniline moieties in the axle of the rotaxanes.
The crown ethers chosen for this investigation included 24C8 3a, DB24C8 3b, possessing two benzene rings, and DN24C8 3c, bearing two naphthalene rings (Scheme 1). We synthesized the rotaxanes 4 in a series of steps, with imine bond formation as the key step; this is an effective method for selectively synthesizing thermodynamically stable rotaxanes.8 The ring encapsulation was facilitated by hydrogen bonding between secondary ammonium groups and crown ethers.6 Condensation of 3,5-diphenylbenzaldehyde9 with benzylamine 1 followed by reduction of the imine produced the corresponding secondary amine. Deprotection and salt formation of the aminoacetal provided the ammonium salt 2, which had the crown-recognition part and bulky stopper at one end. The solubility of 2 in dichloromethane was poor; the addition of DB24C8 improved its solubility, suggesting the formation of the pseudorotaxane. The key imine bond formation by reaction of 2 with 3,5-diphenylaniline10 was performed in the presence of the crown ether in dichloroethane. After formation of the complex between 2 and 3b and reduction of the imine formed by reaction with 3,5-diphenylaniline, the 1H NMR spectrum of the intermediate crude product indicated the presence of translational isomers. Treatment of the crude product with hexafluorophosphoric acid and ammonium hexafluorophosphate produced rotaxane 4b, having a dialkylammonium group encapsulated in DB24C8. The 1H NMR spectrum of 4b clearly showed the hydrogen bonding between the ammonium ion and the crown ether; the signals of CH2N protons of the dumbbell-shaped units appear at 4.53 and 4.71 ppm for the rotaxane 4b, and the signals of the aliphatic protons of the DB24C8 component appear at 3.31, 3.53–3.70, and 3.93–4.07 ppm. These chemical shift assignments are consistent with those reported previously for structurally similar systems.6,7
Similarly, rotaxanes (4a and c) and the axle molecule of rotaxanes 4d were isolated in moderate yields. Selective acylation of the dialkylamino group in rotaxanes 4 was used to selectively encapsulate the aniline nitrogen with crown ethers. Rotaxanes 4 possess dialkylammonium and alkyl aryl amino groups, where the dialkylammonium moiety is protected by the crown ethers. Consequently, selective acylation of the dialkylammonium moiety was found to be difficult. However, it is interesting to note that when rotaxanes 4 were treated with excess triethylamine and di-t-butyl dicarbonate (Boc2O), they were selectively converted to the nonionic rotaxanes 5.11 Furthermore, the acylation of the hexafluorophosphonium salt 4b afforded 5b in poor yield, probably because the benzylic position on the aniline part was cleaved by the hexafluorophosphonate ion or the corresponding acid.12 An exchange of the counter anion improved the yield of the acylation reaction for 4b and d, and the direct acylation of carboxylate salts (4a and c) gave corresponding rotaxanes.
The absorption spectra of rotaxanes (5a and b) and their axle component 5d were recorded in acetonitrile at room temperature. For the two rotaxanes and axle component, the absorption spectra show an intense band with a maximum around 260 nm, attributed to the absorption of the 3,5-diphenylphenyl units and the wheel component, and a weak and broad band with a maximum around 350 nm, which is ascribed to the 3,5-diphenylaniline moiety (n-π*).13 However, these rotaxanes bands are extremely small because of the protonation of the aniline moiety. The latter forms an ammonium salt by reaction with CO2 in the air, resulting in a selective binding of the crown ether to the protonated nitrogen. Addition of triethylamine increases the intensity of the bands; absorption maxima (λmax) of rotaxanes (5a, 5b, and 5c) and the free axle 5d were observed at 348, 346, 346, and 334 nm, respectively (Figure 1a). The fluorescence emission maxima of rotaxanes also shifted to longer wavelengths, comparable to that of the axle component (431 (5a), 432 (5b), 430 (5c), and 399 nm (5d)) (Figure 1b). The wavelength shifts showed the shielding effects of rotaxanes; noncovalent interaction between the axle and wheels seems to cause a decrease the energy gaps between HOMO–LUMO orbitals.
The X-ray structure analysis of pseudorotaxane from N-benzylanilinium-DB24C8 showed functionality-specific π-stacking interaction between the benzene rings of DB24C8 and the axles.14,15 In their study, the benzene ring of the aniline connected to an electron-withdrawing group (4-trifluoromethyl) positioned in the center of both benzene rings of DB24C8, and these distances between aniline-benzene and crown-benzene rings are in the upper range for π-stacking interactions. In contrast, there are no face-to-face π-stacking interactions between these benzene rings when the aniline is substituted with an electron-donating group (4-methoxy).
In the present study, the π-stacking interaction in rotaxane 5b is weak, because the aniline moiety has two electron-donating and bulky groups (phenyl) at the 3 and 5 positions. Unexpectedly, introduction of two naphthalene rings in the wheel has no effect on the photochemical properties of the aniline unit.
In summary, we have synthesized [2]rotaxanes 5 comprising N-alkylaniline moieties with crown ethers using a process involving reversible imine formation and a specific N-acylation reaction. Their photochemical properties have been investigated; crown ether-wrapping resulted in long-wave shifting of aniline moiety in UV-vis and emission spectra.
ACKNOWLEDGEMENTS
We are grateful to University of Fukui for financial support.
References
1. A. G. MacDiarmid, 'Conjugated Polymers and Related Materials,' ed. by W. R. Salaneck, I. Lundström, and B. Rånby, Oxford University Press, Oxford, 1993, pp. 73–98.
2. a) A. E. Kaifer and M. Gómez-Kaifer, 'Supramolecular Electrochemistry,' Wiley-VCH, Weinheim, 2000; b) C. M. Cardona, S. Mendoza, and A. E. Kaifer, Chem. Soc. Rev., 2000, 29, 37; CrossRef c) V. Balzani, A. Credi, F. M. Raymo, and J. F. Stoddart, Angew. Chem. Int. Ed., 2000, 39, 3348; CrossRef d) J. W. Lee, S. Samal, N. Selvapalam, H.-J. Kim, and K. Kim, Acc. Chem. Res., 2003, 36, 621; CrossRef e) M. J. Frampton and H. L. Anderson, Angew. Chem. Int. Ed., 2007, 46, 1028; CrossRef f) Y. H. Ko, E. Kim, I. Hwang, and K. Kim, Chem. Commun., 2007, 1305. CrossRef
3. a) E. Cordova, R. A. Bissell, N. Spencer, P. R. Ashton, J. F. Stoddart, and A. E. Kaifer, J. Org. Chem., 1993, 58, 6550; CrossRef b) E. Cordova, R. A. Bissell, and A. E. Kaifer, J. Org. Chem., 1995, 60, 1033. CrossRef
4. a) R. Eelkema, K. Maeda, B. Odell, and H. L. Anderson, J. Am. Chem. Soc., 2007, 129, 12384; CrossRef b) Y. Liu, J. Shi, Y. Chen, and C.-F. Ke, Angew. Chem. Int. Ed., 2008, 47, 7293. CrossRef
5. K. Yoshida, T. Shimomura, K. Ito, and R. Hayakawa, Langmuir, 1999, 15, 910. CrossRef
6. P. R. Ashton, P. J. Campbell, P. T. Glink, D. Philp, N. Spencer, J. F. Stoddart, E. J. T. Chrystal, S. Menzer, D. J. Williams, and P. A. Tasker, Angew. Chem., Int. Ed. Engl., 1995, 34, 1865. CrossRef
7. a) Y. Tokunaga, M. Yoshioka, T. Nakamura, T. Goda, R. Nakata, S. Kakuchi, and Y. Shimomura, Bull. Chem. Soc. Jp., 2007, 80, 1377; CrossRef b) Y. Tokunaga, T. Goda, N. Wakamatsu, R. Nakata, and Y. Shimomura, Heterocycles, 2006, 68, 5. CrossRef
8. For examples, see: a) S. J. Cantrill, S. J. Rowan, and J. F. Stoddart, Org. Lett., 1999, 9, 1363; CrossRef b) M. Horn, J. Ihringer, P. T. Glink, and J. F. Stoddart, Chem. Eur. J., 2003, 9, 4046; CrossRef c) H. Kawai, T. Umehara, K. Fujiwara, T. Tsuji, and T. Suzuki, Angew. Chem. Int. Ed., 2006, 45, 4281. CrossRef
9. C. A. Barker, X. Zeng, S. Bettington, A. S. Batsanov, M. R. Bryce, and A. Beeby, Chem. Eur. J., 2007, 13, 6710. CrossRef
10. G. E. Greco and R. R. Schrock, Inorg. Chem., 2001, 40, 3850. CrossRef
11. Specific N-acylations of both amino groups will be described elsewhere.
12. M. de Kort, A. W. Tuin, S. Kuiper, H. S. Overkleeft, G. A. van der Marelb, and R. C. Buijsmana, Tetrahedron Lett., 2004, 45, 2171. CrossRef
13. S. Qi, K. Shi, H. Gao, Q. Liu, and H. Wang, Molecules, 2007, 12, 988. CrossRef
14. S. J. Loeb, J. Tiburcio, and S. J. Vella, Org. Lett., 2005, 7, 4923. CrossRef
15. A rotaxane possessing an anilinium station was also reported: a) D. A. Leigh and A. R. Thomson, Org. Lett., 2006, 8, 5377; CrossRef b) F. Coutrot and E. Busseron, Chem. Eur. J., 2008, 14, 4784. CrossRef