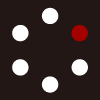
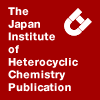
HETEROCYCLES
An International Journal for Reviews and Communications in Heterocyclic ChemistryWeb Edition ISSN: 1881-0942
Published online by The Japan Institute of Heterocyclic Chemistry
e-Journal
Full Text HTML
Received, 16th June, 2009, Accepted, 13th July, 2009, Published online, 14th July, 2009.
DOI: 10.3987/COM-09-S(S)36
■ Synthesis of (+)-Batzelladine K
Miyuki Sekine, Yumi Iijima, Osamu Iwamoto, and Kazuo Nagasawa*
Institute of Symbiotic Science and Technology, Tokyo University of Agriculture and Technology, 2-24-16 Nakamachi, Koganei, Tokyo 184-8588, Japan
Abstract
Total synthesis of batzelladine K (1), a marine guanidine alkaloid, was achieved based upon successive 1,3-dipolar cycloaddition reaction of cyclic nitrone. The relative and absolute stereochemistry of 1 was established by spectral comparison of the natural and synthetic products and the trans-isomer, which was also synthesized.Many natural products possessing a guanidine functional group have been isolated, and have attracted much attention because of their wide range of biological activities.1 Batzelladines, a unique family of polycyclic guanidine alkaloids, were reported to influence protein-protein interactions. In particular, batzelladines A-E block interaction between HIV envelope glycoprotein gp120 and the extracellular domains of human CD4 receptor protein,2 while batzelladines F and G induce dissociation of the complex between tyrosine kinase p56lck and CD4.3 Consequently, considerable synthetic efforts have been devoted to batzelladines,4 and total syntheses of batzelladines A, D, E, and F have been reported.5 Structure-activity relationship studies have also been reported, and some synthetic derivatives were found to regulate characteristic protein-protein interactions of Nef-p52, Nef-actin, and Nef-p56lck.6 Recently, new analogs of the batzelladine family, batzelladines K-N, were reported by Hamann et al.7 We are interested in the modulation of protein-protein interactions by batzelladines,8 and therefore planned to synthesize batzelladine K (1), which corresponds to the left-hand tricyclic guanidine structure of batzelladine F (2). Herein, we report a synthesis of batzelladine K (1) based upon a strategy involving successive 1,3-dipolar cycloaddition (1,3-DC). The chemical structure of 1, including relative and absolute stereochemistry, was confirmed by this synthesis.
As shown in the synthetic plan in Scheme 1, a successive 1,3-DC reaction strategy was applied for synthesis of batzelladine K (1). For this approach, optically active nitrone 3,9 propylene (4) and 1-heptene (6) were required. However, propylene, which is a gas at ambient temperature, is not suitable for 1,3-DC reaction under heating, so allyl alcohol (5) was used as a synthetic equivalent of propylene.
1,3-DC reaction of the optically active nitrone 3 in allyl alcohol at 90 °C gave isoxazolidine, whose hydroxyl group was protected as pivalate to give 7 in 69% yield (2 steps). The regioselective oxidation of 7 with m-CPBA gave nitrone 8, which was subjected to a second 1,3-DC reaction with 1-heptene (6) in toluene to give isoxazolidine 9 in 63% yield from 7. Next, inversion of the stereochemistry of the secondary alcohol and conversion of the methyl group at C2 were conducted. Thus, mesylation of the secondary alcohol with methanesulfonyl chloride and triethylamine followed by treatment with potassium carbonate in methanol gave 10, whose epoxy group was reduced regioselectively with LiAlH4 to give the alcohol 11. The resulting alcohol was protected with acetate to give 12 in 84% yield from 9. Deoxygenation of the secondary alcohol at C5 of 12, which was obtained by deprotection of the silyl ether in 12 with n-Bu4NF (85%), was conducted by the Barton-McCombie method10 to give 13 in 75% yield. Oxidation of 13 with m-CPBA in dichloromethane regioselectively regenerated nitrone 14, and stepwise reduction of 14 with PtO2 and Raney-Ni under hydrogen provided cis-substituted pyrrolidine 16.11 Guandination of the pyrrolidine 16 with bis-Cbz-methylthiopseudourea (17), mercury (II) chloride and triethylamine followed by cyclization under the Mitsunobu reaction conditions in the presence of DEAD and triphenylphosphine gave bicyclic guanidine 19 quantitatively. Tricyclic guanidine 1 was quantitatively synthesized by selective deprotection of the Cbz group in 19 with sodium hydride in THF-methanol followed by mesylation of the resulting alcohol. Finally, deprotection of the Cbz group with Pd-C under hydrogen furnished batzelladine K (1) in 32% yield from 18.
To confirm the reported structure for batzelladine K (1),12 the trans-isomer at C2 and C4 of batzelladine K (1), i.e., trans-batzelladine K (27), was also synthesized based upon a successive 1,3-DC protocol. Thus, the alcohol 20 obtained from the 1,3-DC reaction of nitrone 3 with allyl alcohol was converted into 21 by mesylation of the alcohol followed by reduction with LiAlH4 in 70% yield from 20. Oxidation of isoxazolidine 21 with m-CPBA generated nitrone and subsequent 1,3-DC reaction with 6 stereoselectively gave 22 in 59% yield. After protection of the hydroxyl group with acetate, the TIPS ether at C5 was removed by the Barton-McCombie method10 to give 23 in 80% yield from 22. Hydrogenolysis of 23 in the presence of zinc powder in HCl-aq gave trans-disubstituted-β-hydroxy pyrrolidine 24, which was subsequently treated with bis-Cbz-methylthiopseudourea (17), mercury (II) chloride and triethylamine to afford 25 in 77% yield from 23. Synthesis of trans-batzelladine K (27) from 25 was completed in the same manner as described for 18, providing 27 in 34% yield from 26.
The 13C NMR spectra data of batzelladine K (1) (reported and synthetic) and its trans-isomer 27 are summarized in Table 1.13 The spectral data for synthetic 1 were in good agreement with reported values for the natural product. On the other hand, distinct differences were observed in the C4, 7, and 9 signals of the trans-isomer 27. Thus, we have succeeded in the synthesis of natural batzelladine K (1). Since the optical rotation of synthetic 1 was found to be +2.7 (c 0.4, MeOH), (lit.,7 +6.4 (c 0.1, MeOH)), the absolute stereochemistry of batzelladine K (1) was also defined.
In summary, we have achieved total synthesis of batzelladine K (1) and its trans-isomer 27 based upon successive 1,3-dipolar cycloaddition reaction of cyclic nitrone. The relative and absolute stereochemistry of 1 was thereby established.
EXPREMENTAL
General
Flash column chromatography was performed on Silica gel 60 (spherical, particle size 0.040 ~ 0.100 µm; Kanto). Optical rotations were measured on a JASCO P-2200 polarimeter, using the sodium lump (589 nm). 1H and 13C NMR spectra were recorded on JEOL JNM-ECA 500 or JNM-ECX 400. Mass spectra were recorded on JEOL JMS-T100X spectrometer with ESI-MS mode using methanol as solvent.
Isoxazolidine 7. A mixture of nitrone 3 (2.44 g, 9.50 mmol) and allyl alcohol (30 mL, 0.44 mol) was heated at 90 °C for 30 h. Reaction mixture was concentrated in vacuo, and the residue was purified by flash column chromatography on silica gel (hexane/EtOAc = 8:1 to 4:1) to give isoxazolidine 20 (2.32 g, 77 %). Spectral data for 20: [α]D18 -12 (c 1.5, CHCl3); 1H NMR (400 MHz, CDCl3) δ 4.15 (m, 2H), 3.65 (dd, J = 3.2, 11.9 Hz, 2H), 3.56 (dd, J = 5.5, 11.9 Hz, 1H), 3.30 (dd, J = 4.6, 8.7 Hz, 2H), 2.43 (ddd, J = 5.0, 9.2, 12.8 Hz, 1H), 2.18 (m, 1H), 2.10 (ddd, J = 4.1, 7.3, 12.4 Hz, 1H), 1.70 (m, 1H), 1.04 (brs, 21H); 13C NMR (100 MHz, CDCl3) δ 79.5, 77.8, 75.3, 64.7, 55.3, 36.3, 34.3, 17.9, 12.0 ppm; HRMS (ESI, M+Na+) calcd for C16H33NNaO3Si 338.2127, found 338.2122. To a solution of isoxazolidine 20 (535 mg, 1.70 mmol), pyridine (411 µL, 5.09 mmol) , and DMAP (20.7 mg, 0.169 mmol) in CH2Cl2 (20 mL) was added pivaloyl chloride (413 µL, 3.39 mmol) at 0 °C under N2 atmosphere, and stirred for 18 h at rt. To the reaction mixture was added sat. aq. NH4Cl, and the organic layer was extracted with CH2Cl2. The combined organic layer was dried over MgSO4, filtered and concentrated in vacuo. The residue was purified by flash column chromatography on silica gel (hexane/EtOAc = 30:1 to 20:1) to give isoxazolidine 7 (606 mg, 89%). Spectral data for 7: [α]D18 -15 (c 1.5, CHCl3); 1H NMR (400 MHz, CDCl3) δ 4.23 (ddd, J = 5.7, 6.4, 12.1 Hz, 1H), 4.16 (ddd, J = 2.7, 2.8, 5.5 Hz, 1H), 4.09, (s, 1H), 4.08 (d, J = 1.8 Hz, 1H), 3.62 (ddd, J = 2.7, 3.7, 8.7 Hz, 1H), 3.36 (ddd, J = 6.4, 9.7, 12.9 Hz, 1H), 3.25 (ddd, J = 3.9, 7.1, 12.9 Hz, 1H), 2.30 (ddd, J = 6.0, 8.7, 12.8 Hz, 1H), 2.13 (m, 2H), 1.72 (ddd, J = 3.4, 3.7, 6.4, 12.8 Hz, 1H), 1.20 (s, 9H), 1.04 (brs, 21H); 13C NMR (100 MHz, CDCl3) δ 178.4, 79.0, 75.0, 74.3, 64.8, 55.4, 38.7, 37.1, 34.3, 27.1, 17.9, 12.0 ppm; HRMS (ESI, M+Na+) calcd for C21H41NNaO4Si 422.2703, found 422.2716.
Alcohol 9. To a solution of isoxazolidine 7 (287 mg, 0.716 mmol) in CH2Cl2 (7.0 mL) was added m-CPBA (193 mg, 0.861 mmol) at 0 °C under N2 atmosphere. After stirring for 10 min at 0 °C, to the reaction mixture was added 10% aq. Na2S2O3 and sat. aq. NaHCO3. The resulting mixture was extracted with CH2Cl2 and CHCl3. The combined organic layer was dried over MgSO4, filtered and concentrated in vacuo to give nitrone 8. A mixture of the crude nitrone 8 and 1-heptene (1.5 mL, 10.8 mmol) in toluene (1.0 mL) was heated at 50 °C for 30 h, and the reaction mixture was concentrated in vacuo. The residue was purified by flash column chromatography on silica gel (hexane/EtOAc = 8:1 to 4:1) to give alcohol 9 (230 mg, 0.448 mmol, 63% in 2 steps). Spectral data for 9: [α]D16 -5.6 (c 2.0, CHCl3); 1H NMR (400 MHz, CDCl3) δ 4.21 (m, 2H), 4.09 (d, J = 5.5 Hz, 2H), 4.04 (q, J = 7.0 Hz, 1H), 3.80 (dq, J = 1.9, 7.7 Hz, 1H), 3.24 (dt, J = 3.7, 7.9 Hz, 1H), 2.34 (ddd, J = 6.4, 6.9, 13.3 Hz, 1H), 2.10 (ddd, J = 2.6, 5.3, 11.9 Hz, 1H), 1.94 (q, J = 9.4 Hz, 1H), 1.92 (m, 1H), 1.66 (ddd, J = 7.8, 8.7, 16.5 Hz, 1H), 1.65 (q, J = 7.8 Hz, 1H), 1.62 (dd, J = 2.7, 8.2 Hz, 1H), 1.29 (m, 8H), 1.20 (s, 9H), 1.04 (brs, 21H), 0.87 (t, J = 6.6 Hz, 3H); 13C NMR (100 MHz, CDCl3) δ 178.5, 75.7, 73.6, 70.5, 68.0, 67.3, 61.4, 41.7, 40.6, 38.8, 34.1, 32.3, 31.8, 27.2, 26.2, 22.5, 18.0, 14.0, 12.2 ppm; HRMS (ESI, M+Na+) calcd for C28H55NNaO5Si 536.3747, found 536.3783.
Isoxazolidine 12. To a solution of alcohol 9 (285 mg, 0.554 mmol) and Et3N (232 µL, 1.66 mmol) in CH2Cl2 (5.5 mL) was added MsCl (64 µL, 0.831 mmol) at 0 °C under N2 atmosphere, and the mixture was stirred for 10 min. To the reaction mixture was added sat. aq. NaHCO3, and the resulting mixture was extracted with CHCl3 and EtOAc. The combined organic layer was dried over MgSO4, filtered and concentrated in vacuo to give mesylate. To a solution of the crude mesylate in MeOH (5.5 mL) was added K2CO3 (153 mg, 1.11 mmol) at 0 °C, and the mixture was stirred for 30 h at rt. To the reaction mixture was added water at 0 °C and the organic layer was extracted with EtOAc. The combined organic layer was dried over MgSO4, filtered and concentrated in vacuo to give epoxide 10. To a solution of crude epoxide 10 (226 mg) in Et2O (5.5 mL) was added LiAlH4 (153 mg, 4.02 mmol) at 0 °C under N2 atmosphere, and the resulting mixture was stirred for 10 min. To the reaction mixture was added H2O (50 µL), 15 % NaOH aq. (50 µL) and H2O (150 µL), and the resulting mixture was filtered through a pad of Celite. The filtrates were concentrated in vacuo to give alcohol 11. To a solution of the crude alcohol 11 in pyridine (3.0 mL) was added acetic anhydride (2.0 mL) at rt, and the mixture was stirred for 22 h. The reaction mixture was concentrated in vacuo, and the residue was purified by flash column chromatography on silica gel (hexane/EtOAc = 12:1 to 9:1) to give isoxazolidine 12 (213 mg, 0.467 mmol, 84% in 4 steps). Spectral data for 12: [α]D16 -6.7 (c 2.8, CHCl3); 1H NMR (400 MHz, CDCl3) δ 5.17 (m, 1H), 4.08 (dq, J = 5.5, 9.6 Hz, 1H), 3.87 (q, J = 6.7 Hz, 1H), 3.72 (dq, J = 2.2, 8.0 Hz, 1H), 2.90 (dt, J = 5.0, 7.3 Hz, 1H), 2.23 (ddd, J = 6.4, 7.3, 12.4 Hz, 1H), 2.02 (ddd, J = 2.8, 5.5, 11.9 Hz, 1H), 1.99 (brs, 3H), 1.86 (dt, J = 9.6, 11.9 Hz, 1H), 1.78 (ddd, J = 2.3, 5.0, 5.5 Hz, 1H), 1.60 (dt, J = 7.3, 12.8 Hz, 2H), 1.52-1.33 (m, 1H), 1.27 (m, 7H), 1.25 (d, J = 6.4 Hz, 3H), 1.04 (brs, 21H), 0.86 (t, J = 6.9 Hz, 3H); 13C NMR (100 MHz, CDCl3) δ 170.3, 75.1, 74.8, 70.3, 69.7, 61.9, 42.1, 40.4, 39.7, 32.5, 31.8, 26.2, 22.5, 21.5, 19.6, 18.0, 13.9, 12.1 ppm; HRMS (ESI, M+Na+) calcd for C25H49NNaO4Si 478.3329, found 478.3326.
Acetate 13. To a solution of isoxazolidine 12 (347 mg, 0.761 mmol) in THF (8.0 mL) was added TBAF (398 mg, 1.52 mmol) at 0 °C, and the mixture was stirred for 30 min. To the reaction mixture was added sat. aq. NH4Cl, and the resulting mixture was extracted with EtOAc. The extracts were dried over MgSO4, filtered and concentrated in vacuo. The residue was purified by flash column chromatography on silica gel (hexane/EtOAc = 4:1 to 1:1, then EtOAc/MeOH = 1:0 to 10:1) to give alcohol (194 mg, 0.650 mmol, 85 %). To a solution of the alcohol (165 mg, 0.551 mmol) in THF (5.5 mL) was added thiocarbonyldiimidazole (437 mg, 2.20 mmol), and the mixture was stirred at 60 °C for 14 h. Reaction mixture was concentrated in vacuo, and the residue was filtered through a short pad of silica gel column with an eluent of hexane/EtOAc = 10:1 and 5:1 to give thiocarbamate (224 mg, 0.548 mmol, 99%). To a solution of thiocarbamate (176 mg, 0.430 mmol) in toluene (4.5 mL) was added n-Bu3SnH (1.2 mL, 4.3 mmol) and AIBN (14.1 mg, 0.0860 mmol) at rt, and the mixture was heated at 100 °C for 30 min. The reaction mixture was concentrated in vacuo, and the residue was purified by silica gel column chromatography (hexane/EtOAc = 1:0 to 4:1 to 1:1) to give acetate 13 (91.0 mg, 0.321 mmol, 75%) and alcohol which was generated by simple elimination of thiocarbamate group (23.6 mg, 0.0789 mmol, 18%). Spectral data for 13: [α]D17 -66 (c 1.5, CHCl3); 1H NMR (400 MHz, CDCl3) δ 5.02 (m, 1H), 3.95 (dt, J = 6.4, 7.8 Hz, 1H), 3.74 (dt, J = 6.0, 7.3 Hz, 1H), 2.99 (m, 1H), 2.06-1.92 (m, 4H), 2.00 (s, 3H), 1.91 (s, 1H), 1.89 (dd, J = 2.8, 6.9 Hz, 1H), 1.60 (m, 1H), 1.54 (ddd, J = 5.0, 8.2, 11.0 Hz, 1H), 1.46 (m, 1H), 1.36 (m, 1H), 1.27 (m, 6H), 1.23 (d, J = 6.4 Hz, 3H), 0.86 (t, J = 6.9 Hz, 3H); 13C NMR (100 MHz, CDCl3) δ 170.6, 75.3, 69.3, 63.7, 63.6, 42.1, 41.2, 32.9, 31.8, 30.7, 29.6, 26.0, 22.5, 21.4, 20.4, 14.0 ppm; HRMS (ESI, M+Na+) calcd for C16H29NNaO3 306.2045, found 306.2045.
Hydroxylamine 15. To a solution of acetate 13 (69.8 mg, 0.247 mmol) in CH2Cl2 (2.5 mL) was added m-CPBA (66.3 mg, 0.296 mmol) at 0 °C under N2 atmosphere, and the mixture was stirred for 10 min. To a reaction mixture was added 10% aq. Na2SO3 and sat. aq. NaHCO3, and the resulting mixture was extracted with CHCl3. The extracts were dried over MgSO4, filtered and concentrated in vacuo. The residue was filtered through a short pad of silica gel column chromatography (hexane/EtOAc = 4:1 to 1:1 and EtOAc/MeOH = 1:0 to 10:1) to give nitrone 14 (48.3 mg, 66%). To a solution of nitrone 14 (84.4 mg, 0.282 mmol) in MeOH (3.0 mL) was added PtO2 (catalytic amount), and the resulting mixture was stirred under H2 atmosphere (balloon) for 3 h. The reaction mixture was filtered through a pad of Celite and concentrated in vacuo. The residue was purified by flash column chromatography on silica gel (hexane/EtOAc = 10:1 to 3:1 to 1:1) to give hydroxylamine 15 (79.8 mg, 0.265 mmol, 94%). Spectral data for 15: [α]D16 +17 (c 0.7, CHCl3); 1H NMR (400 MHz, CDCl3) δ 4.98 (ddq, J = 5.5, 6.4, 13.3 Hz, 1H), 3.95 (m, 1H), 3.12 (m, 1H), 2.79 (m, 1H), 2.05-1.81 (m, 4H), 2.01 (s, 3H), 1.67 (m, 2H), 1.57-1.33 (m, 4H), 1.29 (m, 6H), 1.23 (d, J = 1.3 Hz, 3H), 0.88 (t, J = 6.9 Hz, 3H); 13C NMR (100 MHz, CDCl3) δ 170.6, 69.3, 69.0, 65.8, 65.0, 40.2, 37.5, 36.2, 31.9, 26.4, 25.3, 23.6, 22.6, 21.4, 20.6, 14.0 ppm; HRMS (ESI, M+Na+) calcd for C16H31NNaO4 324.2151, found 324.2152.
Bicyclic guanidine 19. To a solution of hydroxylamine 15 (58.7 mg, 0.195 mmol) in MeOH (2.0 mL) was added Raney-Ni (catalytic amount), and the resulting mixture was stirred under H2 atmosphere (balloon) for 17 h. The reaction mixture was filtered through a pad of Celite, and the filtrates were concentrated in vacuo to give pyrrolidine 16. To a solution of the crude pyrrolidine 16 in DMF (2.0 mL) was added Et3N (86 µL, 0.62 mmol), thiopseudourea 17 (110 mg, 0.308 mmol) and HgCl2 (83.5 mg, 0.308 mmol) at 0 °C under N2 atmosphere, and the mixture was stirred for 1 h. The reaction mixture was diluted with EtOAc, and filtered through a pad of Celite. The filtrates were washed with H2O and the organic layer was dried over MgSO4, filtered and concentrated in vacuo. The residue was purified by flash column chromatography on silica gel (hexane/EtOAc = 6:1 to 3:1) to give guanidine 18 (46.0 mg, 0.0773 mmol, 38% in 2 steps). To a solution of guanidine 18 (44.3 mg, 0.0744 mmol) and triphenylphosphine (58.5 mg, 0.223 mmol) in toluene (1.0 mL) was slowly added DEAD (88 µL, 0.22 mmol, 40% in toluene) at 0 °C under N2 atmosphere. After stirring for 30 min, the reaction was quenched with a drop of H2O and the mixture was concentrated in vacuo. The residue was purified by flash column chromatography on silica gel (hexane/EtOAc = 6:1 to 4:1) to give bicyclic guanidine 19 (53.5 mg, 99%). Spectral data for 19: [α]D17 -128 (c 1.1, CHCl3); 1H NMR (400 MHz, CDCl3) δ 7.35-7.22 (m, 10H), 5.14 (d, J = 12.4 Hz, 1H), 4.98 (d, J = 12.4 Hz, 1H), 4.93 (d, J = 12.6 Hz, 1H), 4.85 (q, J = 6.4 Hz, 1H), 4.80 (d, J = 11.9 Hz, 1H), 4.24 (m, 2H), 3.47 (m, 1H), 2.58 (ddd, J = 3.7, 9.6, 13.3 Hz, 1H), 2.17 (m, 1H), 2.05-1.86 (m, 3H), 2.00 (s, 3H), 1.67 (m, 3H), 1.57 (ddd, J = 6.4, 10.1, 13.3 Hz, 1H), 1.46-1.25 (m, 7H), 1.28 (d, J = 6.4 Hz, 3H), 0.86 (t, J = 6.9 Hz, 3H); 13C NMR (100 MHz, CDCl3) δ 170.5, 160.3, 153.5, 151.2, 137.0, 135.6, 128.5, 128.43, 128.39, 128.2, 127.6, 69.4, 68.2, 66.8, 55.9, 55.3, 55.0, 40.0, 38.7, 36.8, 31.4, 30.0, 28.9, 25.1, 22.6, 21.4, 19.9, 14.0 ppm; HRMS (ESI, M+Na+) calcd for C33H43N3NaO6 600.3050, found 600.3039.
Batzelladine K (1). To a solution of bicyclic guanidine 19 (53.5 mg, 0.0744 mmol) in MeOH-THF = 1:1 (1.0 mL) was added NaH (29.8 mg, 0.744 mmol, 60% dispersion in paraffin liquid) at 0 °C. After stirring for 3 h at rt, the reaction was quenched with a drop of H2O at 0 °C. The resulting mixture was extracted with EtOAc, and the extracts were dried over MgSO4, filtered and concentrated in vacuo. The residue was purified by flash column chromatography on silica gel (hexane/EtOAc = 1:0 to 6:1 to 4:1) to give bicyclic guanidine (34.7 mg, 99%). To a solution of bicyclic guanidine (95.2 mg, 0.151 mmol) and Et3N (105 µL, 0.755 mmol) in CH2Cl2 (2.0 mL) was added methanesulfonyl chloride (59 µL, 0.755 mmol) at 0 °C under N2 atmosphere, and the mixture was stirred for 30 min. To the reaction mixture was added sat. aq. NH4Cl, and the organic layer was extracted with hexane. The extracts were washed with sat. aq. NH4Cl, and the aqueous layer was alkalified to pH 8 with sat. aq. NaHCO3. The resulting mixture was extracted with CHCl3, and the extracts were dried over MgSO4, filtered and concentrated in vacuo. The residue was purified by flash column chromatography on silica gel (CHCl3/MeOH = 200:1 to 50:1 to 10:1) to give tricyclic guanidine (13.1 mg, 99%). To a solution of tricyclic guanidine (27.8 mg) in MeOH (1.0 mL) was added Pd/C (catalytic amount), and the reaction mixture was stirred under H2 atmosphere (balloon) for 25 h. The reaction mixture was filtered through a pad of Celite and concentrated in vacuo. The residue was purified by flash column chromatography on silica gel (CHCl3/MeOH = 1:0 to 100:1) to give batzelladine K (7) (4.0 mg, 0.016 mmol, 32 % in 4 steps).
Isoxazolidine 21. To a solution of isoxazolidine 20 (407 mg, 1.29 mmol) and Et3N (540 µL, 3.87 mmol) in CH2Cl2 (15 mL) was added methansulfonyl chloride (150 µL, 1.94 mmol) at 0 °C, and the mixture was stirred for 10 min. To the reaction mixture was added sat. aq. NaHCO3, and the resulting mixture was poured into brine and extracted with CHCl3. The extracts were dried over MgSO4, filtered and concentrated in vacuo to give mesylate. To a solution of the crude mesylate in Et2O (15 mL) was added LiAlH4 (98.0 mg, 2.58 mmol) at 0 °C, and the resulting mixture was stirred for 30 min. To the reaction mixture was added H2O (0.1 mL), 15% NaOH aq. (0.1 mL) and H2O (0.3 mL), and the resulting mixture was filtered through a pad of Celite and the filtrates were concentrated in vacuo. The residue was purified by flash column chromatography on silica gel (hexane/EtOAc = 15:1 to 9:1) to give isoxazolidine 21 (272 mg, 0.909 mmol, 70% in 2 steps). Spectral data for 21: [α]D13 +4.8 (c 0.5, CHCl3); 1H NMR (400 MHz, CDCl3) δ 4.12 (ddd, J = 2.7, 3.2, 6.0 Hz, 1H), 4.09 (dt, J = 6.0, 6.8 Hz, 1H), 3.63 (dt, J = 2.7, 6.0 Hz, 1H), 3.39 (ddd, J = 6.0, 8.7, 12.4 Hz, 1H), 3.18 (ddd, J = 5.0, 6.4, 11.9 Hz, 1H), 2.09 (m, 2H), 2.05 (m, 1H), 1.72 (m, 1H), 1.24 (d, J = 6.0 Hz, 3H), 1.05 (brs, 21H); 13C NMR (100 MHz, CDCl3) δ 79.0, 74.4, 72.8, 55.7, 42.1, 34.6, 19.2, 18.0, 12.1 ppm; HRMS (ESI, M+Na+) calcd for C16H33NNaO2Si 322.2178, found 322.2192.
Alcohol 22. To a solution of isoxazolidine 21 (379 mg, 1.27 mmol) in CH2Cl2 (15 mL) was added m-CPBA (341 mg, 1.52 mmol) at 0 °C, and the mixture was stirred for 10 min. To the reaction mixture was added 10% aq. Na2S2O3 and sat. aq. NaHCO3, and the resulting mixture was extracted with CHCl3. The extracts were washed with brine, and organic layer was dried over MgSO4, filtered and concentrated in vacuo to give nitrone. To a solution of the crude nitrone and 1-heptene (2.8 mL, 19.0 mmol) in toluene (3.0 mL) was heated at 60 °C for 47 h. The reaction mixture was concentrated in vacuo, and the residue was purified by flash column chromatography on silica gel (hexane/EtOAc = 9:1 to 5:1) to give the alcohol 22 (308 mg, 59% in 2 steps). Spectral data for 22: [α]D12 -9.9 (c 1.1, CHCl3); 1H NMR (400 MHz, CDCl3) δ 4.15 (m, 2H), 4.04(q, J = 7.3 Hz, 1H), 3.74 (dq, J = 2.3, 8.1 Hz, 1H), 3.16 (dt, J = 4.1, 7.4 Hz, 1H), 2.32 (quint, J = 6.5 Hz, 1H), 2.07 (ddd, J = 2.3, 5.0, 11.9 Hz, 1H), 1.92 (dq, J = 9.6, 11.9 Hz, 1H), 1.81 (ddd, J = 4.1, 10.1, 14.2 Hz, 1H), 1.63 (m, 3H), 1.52 (m, 1H), 1.40 (m, 1H), 1.29 (m, 5H), 1.20 (d, J = 6.4 Hz, 3H), 1.04 (brs, 21H), 0.87 (t, J = 6.9 Hz, 3H); 13C NMR (100 MHz, CDCl3) δ 75.2, 72.9, 70.6, 65.1, 61.1, 41.9, 40.7, 38.7, 32.3, 31.8, 26.2, 23.1, 22.5, 18.0, 14.0, 12.2 ppm; HRMS (ESI, M+Na+) calcd for C23H47NNaO3Si 436.3223, found 436.3203.
Isoxazolidine 23. To a solution of alcohol 22 (90.8 mg, 0.220 mmol) in pyridine (2.0 mL) was added acetic anhydride (1.0 mL) at rt, and the mixture was stirred for 5 h. The reaction mixture was concentrated in vacuo, and the residue was purified by flash column chromatography on silica gel (hexane/EtOAc = 15:1 to 12:1 to 9:1) to give acetate (99.3 mg, 0.218 mmol, 99%). To a solution of acetate (326 mg, 0.715 mmol) in THF (7.0 mL) was added TBAF (374 mg, 1.43 mmol) at 0 °C. After stirring for 1 h, the reaction was quenched with sat. aq. NH4Cl. The resulting mixture was extracted with EtOAc, and combined organic layer was dried over MgSO4, filtered and concentrated in vacuo. The residue was purified by flash column chromatography on silica gel (hexane/EtOAc = 4:1 to 2:1 and EtOAc/MeOH; 1:0 to 8:1) to give alcohol (256 mg, 99%). To a solution of alcohol (256 mg, 0.715 mmol) in THF (7.0 mL) was added thiocarbonyldiimidazole (566 mg, 2.86 mmol), and the mixture was heated at 60 °C for 12 h. The reaction mixture was concentrated in vacuo, and the residue was purified by silica gel column chromatography (hexane/EtOAc; 10:1 to 6:1 to 4:1) to give thiocarbamate (294 mg). To a solution of thiocarbamate (294 mg, 0.72 mmol) in toluene (7.0 mL) was added n-Bu3SnH (2.0 mL, 7.15 mmol) and AIBN (23.5 mg, 0.143 mmol) at rt under N2 atmosphere, and the resulting mixture was heated at 100 °C for 20 min. The reaction mixture was concentrated in vacuo, and the residue was purified by silica gel column chromatography (hexane/EtOAc = 1:0 to 9:1 to 5:1) to give isoxazolidine 23 (164 mg, 0.579 mmol, 81% in 3 steps) and alcohol which was generated by simply elimination of thiocarbamate group (29.2 mg, 0.0976 mmol, 18% in 3 steps). Spectral data for 23: [α]D13 -61 (c 1.0, CHCl3); 1H NMR (400 MHz, CDCl3) δ 5.06 (m, 1H), 3.94 (m, 1H), 3.73 (m, 1H), 2.99 (dq, J = 6.5, 10.5 Hz, 1H), 2.04 (m, 1H), 2.00 (s, 3H), 1.88 (m, 3H), 1.80 (ddd, J = 4.6, 6.4, 13.7 Hz, 1H), 1.72 (ddd, J = 6.4, 8.7, 14.2 Hz, 1H), 1.57 (m, 1H), 1.45 (m, 2H), 1.37 (m, 2H), 1.27 (m, 5H), 1.23 (d, J = 6.0 Hz, 3H), 0.86 (t, J = 6.6 Hz, 3H); 13C NMR (100 MHz, CDCl3) δ 170.4, 75.2, 69.8, 63.7, 63.6, 42.1, 41.7, 32.9, 31.9, 30.8, 30.0, 26.1, 22.5, 21.4, 20.6, 14.0 ppm; HRMS (ESI, M+Na+) calcd for C16H29NNaO3 306.2045, found 306.2002.
Bicyclic guanidine 26. To a solution of isoxazolidine 23 (49.0 mg, 0.173 mmol) in 3N HCl/THF = 1:3 (2.0 mL) was added freshly activated Zn powder (113 mg, 0.865 mmol) at 0 °C. After stirring for 47 h at rt, the reaction was quenched and neutralized with solid of NaHCO3 at 0 °C. The resulting mixture was extracted with EtOAc/MeOH = 5:1, and the combined organic layer was dried over MgSO4, filtered and concentrated in vacuo to give pyrrolidine 24. To a solution of the crude pyrrolidine 24 in DMF (2.0 mL) was added Et3N (70 µL, 0.5 mmol), thiopseudourea 17 (89.5 mg, 0.250 mmol) and HgCl2 (67.8 mg, 0.250 mmol) at 0 °C under N2 atmosphere. After stirring for 30 min, the reaction mixture was diluted with EtOAc, and filtered through a pad of Celite. The filtrates were washed with H2O twice and the organic layer was dried over MgSO4, filtered and concentrated in vacuo. The residue was purified by flash column chromatography on silica gel (hexane/EtOAc = 6:1 to 3:1) to give guanidine 25 (79.6 mg, 0.134 mmol, 77% in 2 steps). To a solution of guanidine 25 (78.6 mg, 0.132 mmol) and triphenylphosphine (104 mg, 0.396 mmol) in toluene (1.5 mL) was slowly added DEAD (157 µL, 0.396 mmol, 40% in toluene) at 0 °C under N2 atmosphere. After stirring for 20 min, the reaction mixture was quenched with a drop of H2O, and the resulting mixture was concentrated in vacuo. The residue was purified by flash column chromatography on silica gel (hexane/EtOAc = 9:1 to 6:1) to give bicyclic guanidine 26 (70.9 mg, 0.123 mmol, 93%).
trans-Batzelladine K (27). To a solution of bicyclic guanidine 26 (55.6 mg, 0.0963 mmol) in MeOH/THF = 1:1 (1.0 mL) was added NaH (38.5 mg, 0.963 mmol, 60% dispersion in paraffin liquid) at 0 °C. After stirring for 2 h at rt, the reaction mixture was quenched with a drop of H2O at 0 °C. The resulting mixture was extracted with EtOAc, and the extracts were dried over MgSO4, filtered and concentrated in vacuo. The residue was purified by flash column chromatography on silica gel (hexane/EtOAc = 1:0 to 4:1 to 2:1) to give bicyclic guanidine (26.9 mg, 0.0670 mmol, 70%). To a solution of bicyclic guanidine (11.3 mg, 0.0282 mmol) and Et3N (20 µL, 0.14 mmol) in CH2Cl2 (1.0 mL) was added methansulfonyl chloride (11 µL, 0.14 mmol) at 0 °C under N2 atmosphere, and the resulting mixture was stirred for 30 min. To the reaction mixture was added sat. aq. NH4Cl, and organic layer was extracted with hexane. The aqueous layer was ajested to pH 8 with sat. aq. NaHCO3, and the resulting mixture was extracted with an eluent of CH3Cl/MeOH = 5:1. The extracts were dried over MgSO4, filtered and concentrated in vacuo. The residue was purified by flash column chromatography on silica gel (CHCl3/MeOH = 80:1 to 30:1 to 10:1) to give tricyclic guanidine (11.1 mg, 99%). To a solution of tricyclic guanidine (16.3 mg, 0.0291 mmol) in MeOH (1.0 mL) was added Pd/C (catalytic amount), and the reaction mixture was stirred under H2 atmosphere (balloon) for 2 h. The reaction mixture was filtered through a pad of Celite and concentrated in vacuo. The residue was purified by flash column chromatography on silica gel (CHCl3/MeOH; 1:0 to 200:1) to give trans-batzelladine K (27) (3.6 mg, 0.0142 mmol, 49% in 2 steps).
ACKNOWLEDGEMENTS
The work described in this paper was supported by Grants-in-Aid for Scientific Research from the ministry of Education, Science, Sports and Culture, Japan, and the funds from the Mochida Memorial Foundation for Medicinal and Pharmaceutical Research, and the TERUMO Life Science Foundation.
References
1. R. G. S. Berlinck, A. C. B. Burtoloso, and M. H. Kossuga, Nat. Prod. Rep., 2008, 25, 919. CrossRef
2. A. D. Patil, N. V. Kumar, W. C. Kokke, M. F. Bean, A. J. Freyer, C. De Brosse, S. Mai, A. Truneh, D. J. Faulkner, B. Carté, A. L. Breen, R. P. Hertzberg, R. K. Johnson, J. W. Westley, and B. C. M. Potts, J. Org. Chem., 1995, 60, 1182. CrossRef
3. A. D. Patil, A. J. Freyer, P. B. Taylor, B. Carté, G. Zuber, R. K. Johnson, and D. J. Faulkner, J. Org. Chem., 1997, 62, 1814. CrossRef
4. Synthetic studies on batzelladines; a) A. V. R. Rao, M. K. Gurjar, and J. Vasudevan, J. Chem. Soc., Chem. Commun., 1995, 1369; CrossRef b) S. Louwrier, M. Ostendorf, A. Tuynman, and H. Hiemstra, Tetrahedron Lett., 1996, 37, 905; CrossRef c) G. P. Black, P. J. Murphy, N. D. A. Walshe, D. E. Hibbs, M. B. Hursthouse, and K. M. A. Malik, Tetrahedron Lett., 1996, 37, 6943; CrossRef d) B. B. Snider, J. Chen, A. D. Patil, and A. J. Freyer, Tetrahedron Lett., 1996, 37, 6977; CrossRef e) G. P. Black, P. J. Murphy, and N. D. A. Walshe, Tetrahedron, 1998, 54, 9481; CrossRef f) G. P. Black, P. J. Murphy, A. J. Thornhill, N. D. A. Walshe, and C. Zanetti, Tetrahedron, 1999, 55, 6547; CrossRef g) B. B. Snider and M. V. J. Busuyek, J. Nat. Prod., 1999, 62, 1707; CrossRef h) A. S. Franklin, S. K. Ly, G. H. Mackin, L. E. Overman, and A. J. Shaka, J. Org. Chem., 1999, 64, 1512; CrossRef i) S. G. Duron and D. Y. Gin, Org. Lett., 2001, 3, 1551; CrossRef j) P. A. Evans and T. Manangan, Tetrahedron Lett., 2001, 42, 6637; CrossRef k) M. C. Elliott and M. S. Long, Tetrahedron Lett., 2002, 43, 9191; CrossRef l) M. C. Elliott and M. S. Long, Org. Biomol. Chem., 2004, 2, 2003; CrossRef m) C. D. Davies, M. C. Elliott, J. Hill-Cousins, M. A. Khan, T. Maqbool, and J. L. Wood, Synlett, 2008, 2028. CrossRef
5. Total synthesis of batzelladines; a) B. B. Snider and J. Chen, Tetrahedron Lett., 1998, 39, 5697; CrossRef b) F. Cohen, L. E. Overman, and S. K. L. Sakata, Org. Lett., 1999, 1, 2169; CrossRef c) F. Cohen and L. E. Overman, J. Am. Chem. Soc., 2001, 123, 10782; CrossRef d) S. K. Collins, A. I. McDonald, L. E. Overman, and Y. H. Rhee, Org. Lett., 2004, 6, 1253; CrossRef e) F. Cohen and L. E. Overman, J. Am. Chem. Soc., 2006, 128, 2604; CrossRef f) M. A. Arnold, S. G. Durón, and D. Y. Gin, J. Am. Chem. Soc., 2005, 127, 6924; CrossRef g) M. A. Arnold, K. A. Day, S. G. Durón, and D. Y. Gin, J. Am. Chem. Soc., 2006, 128, 13255; CrossRef h) P. A. Evans, J. Qin, J. E. Robinson, and B. Bazin, Angew. Chem. Int. Ed., 2007, 46, 7417. CrossRef
6. a) C. A. Bewley, S. Ray, F. Cohen, S. K. Collins, and L. E. Overman, J. Nat. Prod., 2004, 67, 1319; CrossRef b) A. Olszewski, K. Sato, Z. D. Aron, F. Cohen, A. Harris, B. R. McDougall, W. E. Robinson, Jr., L. E. Overman, and G. A. Weiss, Proc. Nat. Acad. Sci., 2004, 101, 14079; CrossRef c) A. Olszewski and G. A. Weiss, J. Am. Chem. Soc., 2005, 127, 12178; CrossRef d) J. Shimokawa, Y. Iijima, Y. Hashimoto, H. Chiba, H. Tanaka, and K. Nagasawa, Heterocycles, 2007, 72, 145. CrossRef
7. H. -M. Hua, J. Peng, D. C. Dunbar, R. F. Schinazi, A. G. de Castro Andrews, C. Cuevas, J. F. Garcia-Fernandez, M. Kelly, and M. T. Hamann, Tetrahedron, 2007, 63, 11179. CrossRef
8. Synthetic studies on batzelladines; a) K. Nagasawa, H. Koshino, and T. Nakata, Tetrahedron Lett., 2001, 42, 4155; CrossRef b) K. Nagasawa, T. Ishiwata, Y. Hashimoto, and T. Nakata, Tetrahedron Lett., 2002, 43, 6383; CrossRef Total synthesis of batzelladines A and D; c) T. Ishiwata, T. Hino, H. Koshino, Y. Hashimoto, T. Nakata, and K. Nagasawa, Org. Lett., 2002, 4, 2921; CrossRef d) J. Shimokawa, K. Shirai, A. Tanatani, Y. Hashimoto, and K. Nagasawa, Angew. Chem., Int. Ed., 2004, 43, 1559; CrossRef e) J. Shimokawa, T. Ishiwata, K. Shirai, H. Koshino, A. Tanatani, T. Nakata, Y. Hashimoto, and K. Nagasawa, Chem. Eur. J., 2005, 11, 6878. CrossRef
9. A. Goti, M. Cacciarini, F. Cardona, and A. Brandi, Tetrahedron Lett., 1999, 40, 2853. CrossRef
10. D. H. R. Barton and S. W. McCombie, J. Chem. Soc., Perkin Trans. 1, 1975, 1574. CrossRef
11. The cis-stereochemistry of 16 was confirmed by nOe experiments with the hydroxyl amine 15.
12. Structural revisions have been made for some batzelladines.4d,f,g, 5a,c, 8a.
13. Spectral data for synthetic 1 and 27. Synthetic 1 : [α]D16 +2.7 (c 0.4, MeOH); 1H NMR (400 MHz, MeOH) δ 3.74 (m, 2H), 3.54 (m, 1H), 3.42 (m, 1H), 2.24 (m, 2H), 2.21 (m, 2H), 1.68 (m, 2H), 1.57 (m, 1H), 1.55 (m, 1H), 1.35 (m, 6H), 1.28 (m, 2H), 1.26 (d, J = 6.4 Hz, 3H), 0.92 (t, J = 7.1 Hz, 3H); HRMS (ESI, M+H+) calcd for C15H28N3 250.2278, found 250.2234. Synthetic 27 : [α]D18 -44 (c 0.4, MeOH); 1H NMR (500 MHz, MeOH) δ 3.62 (m, 2H), 3.60 (m, 1H), 3.50 (m, 1H), 2.30 (m, 2H), 2.20 (t, J = 6.0 Hz, 2H), 1.61 (m, 2H), 1.37 (m, 2H), 1.34 (m, 8H), 1.27 (d, J = 6.3 Hz, 3H), 0.92 (t, J = 6.9 Hz, 3H); HRMS (ESI, M+H+) calcd for C15H28N3 250.2278, found 250.2250.