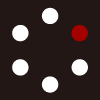
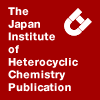
HETEROCYCLES
An International Journal for Reviews and Communications in Heterocyclic ChemistryWeb Edition ISSN: 1881-0942
Published online by The Japan Institute of Heterocyclic Chemistry
e-Journal
Full Text HTML
Received, 30th July, 2009, Accepted, 4th September, 2009, Published online, 9th September, 2009.
DOI: 10.3987/COM-09-S(S)88
■ Structure-Activity Relationships of Synthesized Procyanidin Oligomers: DPPH Radical Scavenging Activity and Maillard Reaction Inhibitory Activity
Akiko Saito and Noriyuki Nakajima*
Biotechnology Research Center, Toyama Prefectural University, 5180 Kurokawa, Kosugi, Toyama 939-0398, Japan
Abstract
Procyanidins are known as condensed tannins and/or oligomeric flavonoids, and are present in many edible plants and show interesting various biological activities. We previously developed and reported a simple and versatile method of synthesizing procyanidin oligomers consisting of (–)-epicatechin and (+)-catechin. Here, we report the structure–activity relationships (SAR) between the DPPH (1,1-diphenyl-2-picrylhydrazyl) radical scavenging activity and Maillard reaction inhibitory activities of various procyanidin oligomers synthesized, including dimers, trimers, tetramers, pentamers, 3-O-substituted oligomers, and other artificial oligomers.INTRODUCTION
Compounds with strong antioxidant and superior radical scavenging abilities are currently of great research interest. Furthermore, foods with ingredients that can degrade active oxygen and free radicals have recently received increased attention. The free radical scavenging activity was assessed by several methods such as, superoxide anions (O2•-) generated by hypoxanthine (HPX)/xanthine oxidase (XOD),1 1,1-diphenyl-2-picrylhydrazyl (DPPH•),2 •OH radicals, damaged DNA detection assay (3D assay).3 It is widely believed that the polyphenols present in fruits and vegetables, as well as various health foods have a beneficial impact on health. Polyphenolic compounds are also increasingly studied because of their strong biological activities. For example, epigallocatechin-3-O-gallate (EGCG), a major polyphenol in green tea, which belongs to the flavan-3-ol group, has notably been the focus of intense research interest for its protective effect against a variety of cancer types, such as lung, prostate, and breast.4
The nonenzymatic browning reaction by heating a mixture of sugar with protein or amino acid is known as the Maillard reaction, which occurs extensively in food and in vivo.5 The relationship of the Maillard reaction to diabetic complications has been noted6 in the field of sitology.7 The aminoguanidine (AG),4 aspirin,8 and vitamin B6,9 have been reported as important Maillard reaction inhibitors. Particularly, quercetin10 and components of tea extracts (catechin derivatives),11 grouped as polyphenols, are also strong inhibitors.
Procyanidins are known as condensed tannins and/or oligomeric flavonoids12,13 and present in various foods, such as, fruits, wine, tea, soybeans, etc. Separation of the individual structural analogs of procyanidins from the plants is very difficult because these compounds are presented as mixtures containing a number of structurally related compounds, and the isolation of compounds depend on the type of plant used. There are many studies on structure–activity relationships (SAR) of phenolic compounds,14 however, research on SAR of procyanidins is less well understood. Development of a systematic technology for synthesizing procyanidins in pure form is useful and important. There have been numerous studies concerning the isolation, synthesis, and bioactivity of procyanidin oligomers.15,16
We previously developed a simple, versatile, stereoselective and size-controlled method of synthesizing procyanidin oligomers consisting of (–)-epicatechin (1) and (+)-catechin (2) (Figure 1). Our synthetic method is applicable to various (4–8) linked procyanidin oligomers, such as dimers and trimers, galloyl derivatives of dimers and trimers, and longer oligomers in good yields.17 Here, we describe the SAR studies between DPPH radical scavenging activity and the Maillard reaction inhibitory activity18 of previously synthesized procyanidin oligomers and their synthetic intermediates having an unnatural structure.
RESULTS AND DISCUSSION
DPPH radical scavenging activity and the Maillard reaction inhibitory activity
The SC50 values (concentration of 50% scavenging activity) of DPPH radical and the IC50 values (concentration of 50% inhibiting activity) of monomeric compounds exhibiting Maillard reaction inhibitory activity are shown in Table1. The SC50 values of the DPPH radicals of (−)-epicatechin 1, (+)-catechin 2, and (−)-epigallocatechin 3 in natural products, were 2.2, 2.6 and 2.3 µM, respectively. Meanwhile, IC50 values of the inhibitory activity of the Maillard reaction of 1, 2, and 3 were 24, 5.0 and 12 µM, respectively. It appeared that the stereochemistry of the 3-hydroxy group affected Maillard reaction inhibitory activity, although radical scavenging activity was unaffected. Esters 4 and 7 showed SC50 values for the radical scavenging activity to be 4.0 and 4.3 µM, respectively. Compounds 5 and 6 in which the phenol groups are protected did not show DPPH radical scavenging activity at all. On the other hand, regarding the Maillard reaction inhibitory activity of compound 5, in which the phenol groups are protected with acetyl groups, it had strong inhibitory activity equivalent to that of 1. The results for monomeric compounds indicate that free phenol groups are necessary and that the stereochemistry of the 3-position does not affect DPPH radical scavenging activity. On the other hand, Maillard reaction inhibitory activities of catechin and its derivatives with 3β-hydroxy stereochemistry are higher than those of epicatechin compounds. In addition, acetylation compounds also have strong inhibitory activities against the Maillard reaction.
Table 2 shows the SC50 values of the DPPH radical scavenging activity and the IC50 values of the Maillard reaction inhibitory activity of synthetic various (+)-catechin dimers. (+)-Catechin-(4α-8)-(+)-catechin dimer 8, known as procyanidin B3, and (+)-catechin-(4β-8)-(+)-catechin dimer 13 were isolated from various plants9,10 and compounds 9-11 and 14 are their acetyl derivatives. Compound 12 is the diester derived from the synthetic intermediate of procyanidin B3 by intramolecular coupling (Scheme 2). The SC50 values of the radical scavenging activity of dimeric compounds 8, 10, 11, 12, and 13 were 1.3, 0.8, 1.8, 1.4 and 0.7 µM, respectively, and they were lower compared to that of catechin 2, a monomeric compound. Interestingly, 3,4-cis isomer (13) of procyanidin B3 showed strong radical scavenging activity as compared with procyanidin B3 (8). This indicates that the radical scavenging activity of procyanidins is influenced not only by the number of phenolic groups but also by structure.19 In the Maillard reaction inhibitory activity, the IC50 values of 8–14 were 3.0, 7.0, 1.0, 5.0, 7.3, 3.0 and 4.0 mM, respectively. Compounds 8, 10, 13, and 14 inhibited more strongly than catechin 2, but 9, 11 and 12, in which the 3- and 3''-positions are protected by ester groups, were not strong inhibitors in spite of double the number of phenol groups. 3-O-Acetate 10 was the strongest inhibitor of the Maillard reaction in the procyanidin B analogs.
It is well known that galloyl derivatives such as EGCG show strong biological activities. We have synthesized many gallate derivatives of procyanidins to enhance their activities. Table 3 summarizes the results for the DPPH radical scavenging activity and the Maillard reaction inhibitory activities. The SC50 values of radical scavenging activity of gallate dimers 15-26 were 0.8, 0.7, 0.6, 0.8, 0.6, 0.6, 3.2, 1.1, 1.1, 0.9, 0.8 and 0.9 µM, respectively. Radical scavenging activity of the epicatechin–containing dimers, activity, the IC50 of 15–32 were in the level of 0.2 to 10 µM, respectively. It appears that the 3''-O-gallate dimers (16, 19, 22, and 25), in which the bottom unit is substituted with the galloyl group, had relatively
weak inhibitory activity. The Maillard reaction inhibitory activity of the epicatechin–containing oligomers, 15–20 and 24–26, increased in the following order, 3,3''-O-digallate > 3-O-gallate > 3''-O-gallate. On the other hand, the oligomers consisting of catechin showed different trends of 3-O-gallate > 3,3''-O-digallate >3''-O-gallate. In addition, the Maillard reaction inhibitory activity of these gallate-substituted oligomers was not correlated with the DPPH radical scavenging activity.
Table 4 summarizes the results for the DPPH radical scavenging and the Maillard reaction inhibitory activities of peracetyl 3-O-galloyl dimers. Peracetates 27-32 shows no DPPH radical scavenging activity at 100 µM. On the other hand, peracetates effectively inhibit the Maillard reaction, the IC50 values of Maillard reaction inhibitory activity were 1.2, 3.5, 3.5, 0.9, 4.2 and 0.6 µM, respectively. The Maillard reaction inhibitory activity of peracetate 27–32 was same level as free phenol dimers 15–26.
The activities of longer oligomers and their peracetates were shown in Table 5 and Table 6. SC50 values of radical scavenging activities of 33–43 were 0.7, 0.7, 1.2, 1.1, 0.9, 0.9, 0.8, 0.4, 0.6, 0.4, and 0.4 µM, respectively. Nearly all these compounds have strong radical scavenging activities. The galloyl trimers 40
and 41, tetramer 42, and pentamer 43 was highly active.21 These compounds also showed strong inhibitory activities against the Maillard reaction (IC50 values were 0.5, 0.4, and 0.7 µM, respectively), but the activity of the pentamer 58 was low (IC50 value was 3.9 µM). Unfortunately, it is not clear whether the number of hydroxyl groups and/or structure affect activity.
Table 6 summarizes the results for the DPPH radical scavenging activity and the Maillard reaction inhibitory activities of peracetates. Peracetates 44-48 shows the Maillard reaction inhibitory activity at 1.0, 1.5, 2.0, 1.4, and 1.9 µM, respectively but no DPPH radical scavenging activity at 100 µM. The Maillard reaction inhibitory activity of peracetate 44–48 was same level as phenol trimers 33–37.
Collectively, among the 48 compounds we studied, 40 (SC50: 0.4 µM, galloyl derivative of 33), 42 (0.4 µM, tetramer), 43 (0.4 µM, pentamer), 41 (0.6 µM, galloyl-dimer, galloyl derivative of 34), 17 (0.6 µM, galloyl-dimer), 19 (0.6 µM, galloyl-dimer), 20 (0.6 µM, galloyl-dimer), 13 (0.7 µM, dimer), 16 (0.7 µM, galloyl-dimer), 33 (0.7 µM, trimer), 34 (0.7 µM, trimer), 10 (0.8 µM, dimer), 15 (0.8 µM, galloyl-dimer),
18 (0.8 µM, galloyl-dimer), 25 (0.8 µM, galloyl-dimer), and 39 (0.8 µM, trimer) were effective as radical scavengers. Galloyl derivatives and tetramer and pentamer showed strong radical scavenging activity. On the other hand, it is interesting to note that no galloyl compound, such as catechin dimer analogs 10 and 13, had relatively high activity, implying that radical scavenging activity is not proportional to the number of hydroxyl groups. Furthermore, reading the Maillard reaction inhibitory activity, 21 (IC50: 0.2 µM, galloyl-dimer), 20 (0.3 µM), 17 (0.4 µM), 41 (0.4 µM), 40 (0.5 µM), 32 (0.6 µM, galloyl-dimer,
peracetate of 20), 42 (0.7 µM), 33 (0.8 µM, trimer), 37 (0.8 µM, trimer), 18 (0.9 µM), 30 (0.9 µM, galloyl-dimer, peracetate of 18), 10 (1.0 µM), 23 (1.0 µM, galloyl-dimer), 26 (1.0 µM, galloyl-dimer) and 44 (1.0 µM, trimer, peracetate of 33) were effective as inhibitors. Similar to radical scavenging activity, galloyl derivatives and longer oligomers have strong Maillard reaction inhibitory activity. Interestingly, 21, which is a weak radical scavenger, shows strongest Maillard reaction inhibitory activity.
CONCLUSION
We studied the structure-activity relationships of synthesized procyanidins. In assays, galloyl derivatives and longer oligomers showed strong activities to the DPPH radical scavenging and the Maillard reaction inhibition. However, the SAR of procyanidins yielded complicated results in spite of simple in vitro assays such as assessing radical scavenging and Maillard reaction inhibitory activities. It is expected that the behavior of procyanidins in vivo is more complex. Further study is required to elucidate the SAR in vitro and in vivo. We are presently attempting to synthesize more various procyanidin analogs in order to examine their biological activities.
EXPERIMENTAL SECTION
DPPH radical scavenging activity22-24
All the samples were assayed after HPLC purification. DPPH radicals were measured as follows. A solution of DPPH radicals in EtOH (30 µM, 10 mL) was added to 1 mL of the test sample in DMSO and incubated at 30°C for 30 min. The scavenging activity was estimated by measuring the absorption of the reaction mixture at 517 nm using a microplate reader (Model 3550, BIO-RAD).
Maillard reaction inhibitory activity18
All the samples were assayed after HPLC purification. The evaluation of in vitro glycation, the Maillard reaction was achieved by using a method described previously. In brief, 500 µL of reaction mixture was prepared containing 400 µg BSA (fraction V, Sigma Chemical Co. Ltd.), 200 mM glucose with or without 10 µL of the synthesized compound in 50 mM phosphate buffer pH 7.4, and the reaction mixture was heated to 60°C on a heat block for indicated hours. A negative control sample was kept at 4°C until measurement. After cooling, aliquots of 100 µL were transferred to new 1.5 mL plastic tubes and 10 µL of 100% w/v TCA was added to each tube. The supernatant containing glucose and the compound was removed after agitation and centrifugation (15,000 rpm, 4°C, 4 min.), and the precipitate of AGEs-BSA was dissolved with 400 µL of alkaline PBS to serve for screening. The changes in fluorescence intensity (ex. 370 nm, em. 440 nm) based on AGEs were monitored by using the spectrofluorometer RF-1500 (Shimadzu, Japan). Real inhibition activity was estimated by subtracting the quenching effect from the apparent inhibitory activity, which was calculated by the above method. The quenching effect was measured by using the same sample dissolved with alkaline PBS after TCA treatment of the mixed solution of 2 µL of inhibitor solution and 100 µL of the control solution incubated for 30 hours.
ACKNOWLEDGMENTS
Partial financial support of this research under the NOVARTIS Foundation (Japan) for the Promotion of Science is gratefully acknowledged. We also thank the Japan Society for the Promotion of Science (JSPS) for the Young Science Research Fellowship (to A. S.).
References
1. E. K. Hodgson and I. Fridovich, Biochim. Biophys. Acta, 1976, 430, 182. CrossRef
2. W. Brand-Williams, M. E. Cuvelier, and C. Berset, Lebensm. Wiss. -Technol., 1995, 28, 25.
3. B. Salles, C. Provot, P. Calsou, and I. A. Hennebelle, Anal. Biochem., 1995, 231.
4. C. S. Yang, P. Maliakai, and X. Meng, Annu. Rev. Pharmacol. Toxicol., 2002, 42, 25. CrossRef
5. A. Kanane and T. P. Labuza, Prog. Clin. Biol. Res., 1989, 304, 301.
6. V. M. Monnier, R. R. Kohn, and A. Cerami, Proc. Natl. Acad. Sci. U. S. A., 1984, 81, 583. CrossRef
7. M. Brownlee, H. Vlassara, A. Kooney, P. Ulrich, and A. Cerami, Science, 1986, 232, 1629. CrossRef
8. R. Huby and J. J. Harding, Exp. Eye Res., 1988, 47, 53. CrossRef
9. A. A. Booth, R. G. Khalifah, and B. G. Hudson, Biochem. Biophys. Res. Commun., 1996, 220, 113. CrossRef
10. Y. Morimitsu, K. Yoshida, S. Esaki, and A. Hirota, Biochem. Biotechnol. Biochem., 1995, 59, 2018.
11. T. Nakagawa, T. Yokozawa, K. Terasawa, S. Shu, and L. R. Juneja, J. Agric. Food Chem., 2002, 50, 2418. CrossRef
12. J. B. Harborne, The Flavonoids: Advances in research from 1986. Chapman and Hall: London, 1993.
13. J. B. Harborne and H. Baxter, The Handbook of Natural Flavonoids, John Wiley & Sons, NY, (1999).
14. G. Cao, E. Sofic, and R. L. Prior, Free Rad. Biol. Med., 1997, 22, 749; CrossRef W. Bors and C. Michel, Free Rad. Biol. Med., 1999, 27, 1413; CrossRef M. Y. Moridani, G. Galati, and P. J. O'Brien, Chemico-Biological Interactions, 2002, 139, 251. CrossRef
15. K. Ohmori, N. Ushimaru, and K. Suzuki, Proc. Natl. Acad. Sci. U. S. A., 2004, 101, 12002; CrossRef I. Tarascou, K. Barathieu, Y. Andre, I. Pianet, E. Dufourc, and E. Fouquet, Eur. J. Org. Chem., 2006, 5367; CrossRef P. K. Sharma, A. Kolchinski, H. A. Shea, J. J. Nair, Y. Gou, L. J., Jr. Romanczyk, and H. H. Schmitz, Org. Process Res. Dev., 2007, 11, 422; CrossRef Y. Mohri, M. Sagehashi, T. Yamada, Y. Hattori, K. Morimura, T. Kamo, M. Hirota, and H. Makabe, Tetrahedron Lett., 2007, 48, 5891; CrossRef K. Oyama, M. Kuwano, M. Ito, K. Yoshida, and T. Kondo, Tetrahedron Lett., 2008, 49, 3176; CrossRef A. C. Matthew, S. L. Bonnet, and J. H. van der Westhuizen, Org. Lett., 2008, 10, 3865; CrossRef Y. Mohri, M. Sagehashi, T. Yamada, Y. Hattori, K. Morimura, T. Kamo, M. Hirota, and H. Makabe, Heterocycles, 2009, 79, 549. CrossRef
16. N. E. Es-Safi, S. Ghidouche, and P. H. Ducrot, Molecules, 2007, 12, 2228. CrossRef
17. A. Saito, N. Nakajima, A. Tanaka, and M. Ubukata, Biosci. Biotechnol. Biochem., 2002, 66, 1764; CrossRef A. Saito, N. Nakajima, A. Tanaka, and M. Ubukata, Tetrahedron, 2002, 58, 7829; CrossRef A. Saito, N. Nakajima, A. Tanaka, and M. Ubukata, Tetrahedron Lett., 2003, 44, 5449; CrossRef A. Saito, N. Nakajima, A. Tanaka, and M. Ubukata, Heterocycles, 2003, 61, 287; CrossRef A. Saito, N. Nakajima, N. Matsuura, A. Tanaka, and M. Ubukata, Heterocycles, 2004, 62, 479; CrossRef A. Saito, A. Tanaka, M. Ubukata, and N. Nakajima, Synlett, 2004, 1069; CrossRef A. Saito, A. Tanaka, M. Ubukata, and N. Nakajima, Synlett, 2004, 2040; CrossRef A. Saito, Y. Doi, N. Matsuura, A. Tanaka, M. Ubukata, and N. Nakajima, Bioorganic & Medicinal Chemistry, 2004, 12, 4783; CrossRef A. Saito, M. Emoto, A. Tanaka, Y. Doi, K. Shoji, Y. Mizushina, H. Ikawa, H. Yoshida, N. Matsuura, and N. Nakajima, Tetrahedron, 2004, 60, 12043; CrossRef A. Saito, Y. Mizushina, H. Ikawa, H. Yoshida, Y. Doi, A. Tanaka, and N. Nakajima, Bioorganic & Medicinal Chemistry, 2005, 13, 2759; CrossRef H. Sakuda, A. Saito, Y. Mizushina, H. Ikawa, H. Yoshida, A. Tanaka, and N. Nakajima, Heterocycles, 2006, 67, 175. CrossRef
18. N. Matsuura, T. Aradate, C. Sasaki, H. Kojima, J. Hasegawa, and M. Ubukata, J. Health Sci., 2002, 48, 520. CrossRef
19. C. A. Rice-Evans, N. J. Miller, and G. Paganga, Free Rad. Biol. Med., 1996, 20, 933. CrossRef
20. Previous studies obtained same results. N. Saint-Cricq de Gaulejac, C. Provost, and N. Vivas, J. Agric. Food Chem., 1999, 47, 425; CrossRef M. Dueñas, T. Hernández, I. Estrella, and R. Rabanal, Food Chemistry, 2003, 82, 373. CrossRef
21. These data was also reported to the following paper. A. Saito, Y. Mizushina, A. Tanaka, and N. Nakajima, Tetrahedron, 2009, 65, 7455.
22. M. S. Blois, Nature, 1958, 181, 1199. CrossRef
23. F. Nanjo, K. Goto, R. Seto, M. Suzuki, M. Sakai, and Y. Hara, Free Radical Biol. Med., 1996, 21, 895. CrossRef
24. T. Hatano, H. Miyatake, M. Natsume, N. Osakabe, T. Takazawa, H. Ito, and T. Yoshida, Phytochemistry, 2002, 59, 749. CrossRef