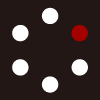
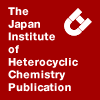
HETEROCYCLES
An International Journal for Reviews and Communications in Heterocyclic ChemistryWeb Edition ISSN: 1881-0942
Published online by The Japan Institute of Heterocyclic Chemistry
e-Journal
Full Text HTML
Received, 27th July, 2009, Accepted, 25th August, 2009, Published online, 26th August, 2009.
DOI: 10.3987/COM-09-S(S)77
■ Novel and Facil Synthesis and Evaluation of Antitumor Activities of 6,7-Bisaryl-1-(β-D-ribofuranosyl)pteridine-2,4(1H,3H)-diones
Rafiya Khan Kandahary, Abugafar M. L. Hossion, Noriyuki Ashida, and Tomohisa Nagamatsu*
Graduate School of Medicine, Dentistry and Pharmaceutical Sciences, Okayama University, 1-1-1 Tsushima-naka, Okayama 700-8530, Japan
Abstract
Novel 6,7-bisaryl-1-(β-D-ribofuranosyl)pteridine-2,4(1H,3H)-dione derivatives were synthesized by condensation of 5,6-diamino-2’,3’-O-iso-propylideneuridine, which was derived from uridine, with an appropriate α,β-diketone, followed by acidic hydrolysis allowing removal of the isopropylidene group of the sugar moiety as a protecting group. Moreover, several N-3 alkyl derivatives of the 6,7-bisaryl-1-(β-D-ribofuranosyl)pteridine-2,4(1H,3H)-diones were obtained by alkylation of the 6,7-bisaryl-1-(2’,3’-O-isopropylidene-β-D-ribofuranosyl)pteridine-2,4(1H,3H)-diones with alkyl halides and by their acidic hydrolysis for deprotection. The antitumor activities of all synthesized compounds against CCRF-HSB-2 and KB cell lines were also investigated in vitro and some of the compounds showed prospective antitumor activities.INTRODUCTION
The development of practical and efficient route for synthesis of the drug like small molecules is of considerable interest to medicinal chemists and chemical biologists.1,2 Nucleoside analogs have been well established as one of the richest sources of antiviral agents despite their toxicity3,4 and the drug resistance.5 Others are active compounds exhibiting antineoplastic,6–9 antibiotic and antifungal properties.10–12 Recently, some nucleoside analogs (NAs) have been developed as antitumor agents, and their activities generally have been shown to result from their incorporation into DNA and/or inhibition of DNA polymerases of cells.13,14 Some nucleoside derivatives as antimetabolite, e.g. 1-(β-D- arabinofuranosyl)cytosine (araC), 2-chloro-2’-deoxyadenosine (CldA), 9-β-D-arabinofuranosyl-2-fluoro- adenine 5’-monophosphate (fludarabine, FaraAMP), 2’-deoxy-2’,2’-difluorocytidine (gemcitabine, dFdC), have been widely used not only as antileukemic agents, but also as antitumor agents against solid tumors.15 Nevertheless, the survival rate of patients still remains low and one of the reasons is the poor sensitivity of tumors to drugs. Therefore, the development of new agents capable of suppressing tumor growth would contribute greatly to a better prognosis and current therapy.16 Then, several approaches of large nucleoside analogue libraries have emerged as an important synthetic goal to chase the high efficiency and velocity of the current biological screenings.
As a part of our ongoing project of novel antitumor agents,17–25 our recent interest is the fused pyrimidines containing pteridine ring systems in which carbohydrate moiety is attached to pyrimidine ring. Several examples of similar nucleosides have been prepared by direct glycosylation into pteridine ring via the silylation method,26 which did not serve the facile path due to containing by-products, nor did good yield.27–31 Herein we describe the first, new and convenient synthetic route of pteridine nucleosides, 6,7-diaryl-1-(β-D-ribofuranosyl)pteridine-2,4(1H,3H)-diones (4b–g), which were derived in several steps from uridine. Various 3-N-alkylated pteridine nucleosides (6a–l) were also prepared by alkylation of the pteridine nucleosides (3c–f) with appropriate alkyl halides in alkali solution, followed by acidic hydrolysis. The purpose of this work is to evaluate their antitumor activities in vitro against CCRF-HSB-2 and KB cell lines and to survey the change of biological activity of various 6,7-bisarylpteridine nucleosides as well as the nucleosides having a different alkyl group at the N-3-position in order to establish structure-activity relationship. Even though two nucleosides (4a and 4b) of the pteridinedione have been previously reported by a different synthetic method,27 we wanted to include them in our study to evaluate any differences in biological activity.
RESULTS AND DISCUSSION
The desired starting material, 6-amino-5-nitroso-2’,3’-O-isopropylideneuridine (1), was synthesized from uridine according to the previously outlined procedure.19 Treatment of the compound (1) with Na2S2O4 in 0.5N aqueous acetic acid solution at room temperature led to the formation of requisite key intermediate 5,6-diamino-2’,3’-O-isopropylideneuridine (2) in 60% yield.32 Subsequent condensation reaction between 5,6-diamino-2’,3’-O-isopropylideneuridine (2) and appropriate α,β-diketones in ethanol at refluxing temperature afforded the corresponding, 1-(2’,3’-O-isopropylidene-β-D-ribofuranosyl)pteridine-2,4(1H, 3H)-dione (3a) and its diphenyl (3b) and 6,7-bisaryl derivatives (3c–g) in 58%, 70%, and 60–65% yields, respectively, as shown in Scheme 1 and Table 1. Then, the 1-(2’,3’-O-isopropylidene-β-D-
ribofuranosyl)pteridine-2,4(1H,3H)-diones (3a–g) thus obtained were treated with 0.5N hydrochloric acid in methanol solution at 50 °C to afford the corresponding deprotected 1-(β-D-ribofuranosyl)pteridine- 2,4(1H,3H)-dione and its 6,7-bisaryl derivatives (4a–g) in 50–76% yields. All new compounds (3c–g and 4c–g) exhibited satisfactory elemental combustion analyses except for 3c and UV, IR, and 1H-NMR spectral data as shown in Tables 1–3, consistent with the structures indicated in Scheme 1. The compounds (3a,b and 4a,b) were identified with the previously reported compounds in all spectral data.27,33 This simple and convenient preparation of the pteridine nucleosides suggested that the previous methods26–31 by direct glycosylation via silylation of the pteridines were not expeditious. On the other hand, synthesis of the N-3 substituted alkyl derivatives (5a–l) of 6,7-bisaryl-1-(2’,3’-O-isopropylidene- β-D-ribofuranosyl)pteridine-2,4(1H,3H)-diones (3c–f) were accomplished by alkylation of the pteridine nucleosides (3c–f) protected by an isopropylidene group on the sugar moiety with appropriate alkyl halides (i.e., BrCH2Ph, BrCH2CO2C2H5, and Br(CH2)3CO2C2H5) in the presence of anhydrous K2CO3 and dry N,N-dimethylformamide (DMF) at 0 °C in 60–70% yields as shown in Tables 4–6. Similarly, the deprotection of 5a–l were attained by acidic hydrolysis in methanolic HCl solution to get the corresponding N-3 substituted alkyl derivatives (6a–l) of 6,7-bisaryl-1-(β-D-ribofuranosyl)pteridine- 2,4(1H,3H)-diones (4c–f) in 60–71% yields (Tables 7–9). Specific rotation, UV, IR, and 1H-NMR spectra and elemental analyses for 5a–l and 6a–l were also used for the structure determination of the newly assigned compounds. The amorphous intermediates (3c and 5a,c) were characterized by UV, IR, and 1H-NMR spectra except for the micro analyses and were used for next reaction without any purification. In the 1H-NMR spectra for 3a–g and 5a–l in CDCl3, the presence of an isopropylidine group at the 2’ and 3’-positions of the sugar moieties indicated two singlet signals for protons of two geminal methyl groups at δ 1.38–1.40 ppm and 1.63–1.67 ppm, respectively. In contrast, the OH proton signals at the 2’ and 3’-positions of 4a–g and 6a–l in DMSO-d6 appeared as doublet at δ 5.05–5.19 ppm and 4.88–4.97 ppm, respectively. Therefore, the appearance of OH protons supported strongly the deprotected structures on the sugar moieties. Each geminal proton of the methylene group of sugar moiety at the 5’-position appeared as triple doublet signals in the region of ca. 3.8–3.9 ppm for 3a–g and 5a–l and ca. 3.4–3.6 ppm for 4a–g and 6a–l with the coupling constant of 12.0–12.3 Hz between two geminal protons. Considering the 1H-NMR spectra of the N-3 substituted alkyl derivatives (6a–l), the alkylations were characterized by disappearance of the singlet signal of NH protons, which were assigned for the compounds (3c–f) at δ 9.0–9.4 ppm. In case of the benzyl substituted compounds of 5a, 5d, 5g, and 5j were particularly showed each singlet signal of the geminal protons for benzylic methlene at ca. δ 5.29 and 5.30 ppm, respectively. The coupling of geminal protons bearing a coupling constant of 16.8 or 17.1 Hz was further observed in the methylene group for the derivatives (6b, 6e, 6h, and 6k) substituted by an ethoxycarbonylmethyl group at the 3-position. On the other hand, most of the pteridine nucleosides in ethanol exhibited three maxima absorption bands at ca. 230–240, 270–290 and 360–380 nm in UV-vis spectra.
BIOLOGICAL ACTIVITY
The modified34 3-(3,4-dimethylthiazol-2-yl)-2,5-diphenyltetrazolium bromide (MTT) assay for cellular growth and survival application method developed by Mosmann35 were used to determine the growth inhibitory effects (antitumor activity) of the synthesized compounds against two human cultured tumor cell lines, CCRF-HSB-2 (human T-cell acute lymphoblastoid leukemia) and KB (human oral epidermoid carcinoma) cells in vitro. The antitumor agent, arabinoside cytosine (Ara-C), was used as a positive control in this study. The results, i.e., 50% inhibitory concentration [IC50 (µg/mL)] of each compound against both cells are summarized in Table 10. As can be seen from the table of evaluated antitumor activities, many pteridine nucleosides (3c,d,f and 5b–e,h,i) protected by a lipophilic isopropylidene group at the 2’ and 3’-position exhibited potential antitumor activities with IC50 value of ca. 6.0–10.0 µg/mL against CCF-HSB-2 cells. In contrast, the deprotected pteridine nucleoside (4a–g) showed moderate activities of ca. 30–100 µg/mL. However, the deprotected pteridine nucleoside (6a–g,j–l) having an alkyl group such as benzyl, ethoxycarbonylmethyl, and ethoxycarbonylpropyl at the 3-position exhibited more potential antitumor activity of 9.0–35.0 µg/mL than the compounds (4a–g). On the other hand, the activities of the protected pteridine nucleosides (3c,f and 5b,c,h,i) against KB cell showed potential antitumor activity with IC50 value of ca. 8.0–10.0 µg/mL, while the deprotected pteridine nucleosides (6a,d,i–l) exhibited potential antitumor activity with IC50 value of ca. 5.0–10.0 µg/mL. Among them, compound (6l) exhibited the best antitumor activity with IC50 value of 4.8 µg/mL against KB cell. It seemed worthwhile to investigate that the 6,7-bis(para-substituted phenyl)pteridine nucleosides tend to increase the activity compare to the unsubstituted and diphenyl pteridine nucleosides (3a,b and 4a,b) as shown in Table 10.
CONCLUSION
In this study, we demonstrated a novel, facile, and general synthesis of 6,7-bisaryl-1-(β-D- ribofuranosyl)pteridine-2,4(1H,3H)-diones (4b–g) in good yields by condensation of 5,6-diamino-2’,3’ -O-isopropylideneuridine (2) and appropriate α,β-diketones, followed by acidic hydrolysis. The N-3 substituted alkyl derivatives (6a–l) were also synthesized by alkylation of 6,7-bisaryl-1-(2’,3’-O- isopropylidene−β-D-ribofuranosyl)pteridine-2,4(1H,3H)-diones (3c–f) with different alkyl halides, followed by acidic hydrolysis to remove the isopropylidene group. All synthesized compounds were screened in vitro against two human cultured tumor cell lines, CCRF-HSB-2 and KB cell lines, and some of the N-6 and N-7 substituted pteridine nucleoside derivatives displayed prospective antitumor activities, which can be new lead compounds as antitumor agents. Further optimization of these new compounds can possibly lead to more active molecules against tumor cells.
EXPERIMENTAL
Mps were determined using a Yanaco micro-melting point apparatus and were uncorrected. IR spectra were recorded using a JASCO FT/ IR-200 spectrophotometer as Nujol mulls. NMR spectra were obtained with the Varian VXR 300 MHz spectrometer. The 1H chemical shifts are expressed in parts per million (ppm) based on internal TMS (0.00 pm) in CDCl3 or DMSO-d6. The coupling constant, J are given in Hz. UV spectra were recorded in EtOH with a BECKMAN DU-600 spectrophotometer. Specific rotations were recorded in dioxane with DIP-1000 digital polarimeter. Elemental analyses were measured by a Yanako CHN Corder MT-5 apparatus. All reagents were of commercial quality from freshly opened containers and were used without further purification. Reaction progress was monitored by analytical thin layer chromatography (TLC) on pre-coated glass plates (silica gel 60 F254-plate-Merck) and products were visualized by UV light. Column chromatography was accomplished on Daisogel IR-60 (63/ 210 μm, Daiso Co.). The reaction temperatures are indicated as the temperature of the oil bath. Anhyd DMF was stored over activated 4Å molecular sieves and other all solvents were dried and freshly distilled prior to use.
5,6-Diamino-2’,3’-O-isopropylideneuridine (2)
A solution of 6-amino-2’,3’-O-isopropylidene-5-nitrosouridine (1, 0.5 g, 1.52 mmol) in 0.5N acetic acid was stirred vigorously at rt and to it Na2S2O4 (0.5 g, 2.87 mmol) was added gradually until becomes colorless. After the reaction was complete, the solution was neutralized with 28% aqueous ammonia. Then, one third of the solution was evaporated and cooled in ice. The solid deposited collected by filtration and then the filtrated solution was again subjected to evaporation under reduced pressure to afford the second crop of 5,6-diamino-2’,3’-O-isopropylideneuridine (2) as colorless powder.
Yield: 0.3 g (60 %); mp 170 °C (from EtOH) (Lit.32); IR (Nujol) νmax or δmax/ cm-1: 3400 (ν, OH), 3300 (νas, NH2), 3200 (νs, NH2), 3100 (ν, NH), 1710 (ν, C=O), 1620 (δ, NH2), 1080 (ν, OH); 1H-NMR (CDCl3) δ: 1.34 and 1.58 (each 3H, each s, 2×CH3), 2.51 (2H, br s, 5-NH2, exchangeable with D2O), 3.80–3.92 (2H, m, 5’-H), 4.12 (1H, m, 4’-H), 4.88 (1H, br, 5’-OH, exchangeable with D2O), 4.98 (1H, dd, J2’,3’ = 6.9 Hz, J3’,4’ = 4.2 Hz, 3’-H), 5.14 (1H, dd, J1’,2’ = 3.9 Hz, J2’,3’ = 6.9 Hz, 2’-H), 6.13 (2H, s, 6-NH2, exchangeable with D2O), 6.51 (1H, d, J1’,2’= 3.9 Hz, 1’-H), 9.37 (1H, br, NH, exchangeable with D2O).
General procedure for the preparation of 1-(2’,3’-O-isopropylidene-β-D-ribofuranosyl)pteridine- 2,4(1H,3H)-dione and its 6,7-bisaryl derivatives (3a–g)
A mixture of 5,6-diamino-2’,3’-O-isopropylideneuridine (2, 0.5 g, 1.59 mmol) and an appropriate α,β-diketone (1.60 mmol) in EtOH was heated under reflux for 18 h. After the reaction was complete, the solvent was evaporated in vacuo to yield yellow solid. The resulting solid was subjected to column chromatography on silica gel using a mixture of EtOAc and n-hexane as eluting solvent to afford the corresponding pure compounds (3a–g) (Table 1–3). The products of 3a and 3b were obtained in 58% and 70% yield, respectively, and were identified with the previously reported compounds in all spectral data.33
General procedure for the preparation of 1-(β-D-ribofuranosyl)pteridine-2,4(1H,3H)-dione and its 6,7-bisaryl derivatives (4a–g)
A solution one of 6,7-bisaryl-1-(2’,3’-O-isopropylidene-β-D-ribofuranosyl)pteridine-2,4(1H,3H)-dione (3a–g, 0.5 g, 0.77–1.49 mmol) in a mixture of MeOH (25 mL) and 0.5N HCl (10 mL) was heated at 50–60 °C for 3–5 h. After cooling to rt, the solution was neutralized with triethylamine and evaporated in vacuo. Finally, co-evaporation of the solution with EtOH to dryness was achieved. The dry residue was treated with absolute ethanol and the insoluble material was filtered off. The filtrate was evaporated in vacuo and the residue was purified by column chromatography on silica gel using a mixture of EtOAc and n-hexane as eluting solvent to give the corresponding products (4a–g) (Table 1–3). The products of 4a and 4b were obtained in 51% and 75% yield, respectively, and were identified with the previously reported compounds in all spectral data.27
General procedure for the preparation of N-3-substituted 6,7-bisaryl-1-(2’,3’-O-isopropylidene- β-D-ribofuranosyl)pteridine-2,4(1H,3H)-diones (5a–l)
A mixture of compounds (3c–f, 0.5 g, 0.77–0.96 mmol) with anhydrous K2CO3 (1.50 mmol) in dried DMF (4–5 mL) was cooled to 0 °C. After addition of an appropriate alkyl halide (2.56–2.90 mmol), the mixture was stirred vigorously at 0 °C for 2–3 h. The reaction mixture was poured into water (10 mL), and extracted with ethyl acetate (2 × 8 mL). The organic layer was dried over anhydrous MgSO4 and evaporated to dryness under reduced pressure. The resulting solid was subjected to column chromatography on silica gel using a mixture of EtOAc and n-hexane as eluting solvent to afford the corresponding pure compounds (5a–l) (Table 4–6).
General procedure for the preparation of N-3-substituted 6,7-bisaryl-1-(β-D-ribofuranosyl)- pteridine-2,4(1H,3H)-diones (6a–l)
A solution of 5a–l (0.5 g, 0.65–0.82 mmol) in a mixture of MeOH (25 mL) and 0.5N HCl (10 mL) was heated at 50–60 °C for 3–5 h. After cooling to rt, the solution was neutralized with triethylamine and evaporated in vacuo. Finally, co-evaporation of the solution with EtOH to dryness was achieved. The dry residue was treated with absolute ethanol and the insoluble material was filtered off. The filtrate was evaporated in vacuo and the residue was purified by column chromatography on silica gel using a mixture of EtOAc and n-hexane as eluting solvent to give the corresponding deprotected N-3 alkyl derivatives (6a–l) (Table 7–9).
Growth inhibitory activities of compounds 3–6 against human tumor cell lines
The synthesized compounds were evaluated in vitro for the growth inhibitory effects against CCRF-HSB-2 (human T-cell acute lymphoblastoid leukemia) and KB (human oral epidermoid carcinoma) cells by the modified [3-(3,4-dimethylthiazol-2-yl)-2,5-diphenyltetrazolium bromide] assay34 for cellular growth and survival application method developed by Mosmann.35 The results, i.e., IC50 (µg/mL) of each compound against both cells are summarized in Table 10.
ACKNOWLEDGEMENTS
The authors are indebted to the SC-NMR Laboratory of Okayama University for the NMR spectral experiments.
References
1. M. D. Bruke and S. L. Schreiber, Angew. Chem. Int. Ed., 2004, 43, 46. CrossRef
2. S. L. Schreiber, Science, 2000, 287, 1964. CrossRef
3. E. A. Petersen, C. H. Ramirez-Ronda, W. D. Hardy, R. Schwartz, H. S. Sacks, S. Follansbee, D. M. Peterson, A. Cross, R. E. Anderson, and L. M. Dunkle, J. Infect. Dis., 1995, 171 (suppl. 2), S131.
4. R. Yarchoan, J. M. Pluda, R. V. Thomas, H. Mitsuya, P. Brouwers, K. M. Wyvill, N. Hartman, D. G. Johns, and S. Broder, Lancet, 1990, 336, 526. CrossRef
5. B. A. Larder, J. Acquir. Immune Defic. Syndr. Hum. Retrovirol, 1995, 10 (Suppl. 1), S28. CrossRef
6. I. M. Lagoja, Chem. Biodivers, 2005, 2, 1. CrossRef
7. K. Kimura and T. D. H. Bugg, Nat. Prod. Rep, 2003, 20, 252. CrossRef
8. S. Rachakonda and L. Cartee, Curr. Med. Chem., 2004, 11, 775. CrossRef
9. S. Knapp, Chem. Rev., 1995, 95, 1859. CrossRef
10. S. Miura and S. Izuta, Curr. Drug Targets, 2004, 5, 191. CrossRef
11. W. B. Parker, J. A. Secrist, and W. R. Waud, Curr. Opin. Investig. Drugs, 2004, 5, 592.
12. S. I. Szafraniec, K. J. Stachnik, and J. S. Skierski, Acta Pol. Pharm., 2004, 61, 223.
13. M. Hejna, G. V. Kornek, M. Raderer, H. Ulrich-Pur, W. C. C. Fiebiger, L. Marosi, B. Schneeweiss, R. Greul, and W. Scheithauer, Cancer, 2000, 89, 516. CrossRef
14. V. Gandhi, E. Estey, M. J. Keating, A. Chucrallah, and W. Plunkett, Blood, 1996, 87, 256.
15. J. A. Montgomery, S. Ananthan, W. B. Parker, J. A. Secrist, and C. G. Temple, ’Cancer Chemotherapy Agents, Series: Antimetabolites,’ ed. by W. O. Foye, ACS professional reference book, Washington DC: Americal Chemical Society, 1995, pp. 47–110.
16. E. Tsoukala, G. Agelis, J. Dolinsek, T. Botic, A. Cencic, and D. Komiotis, Bioorg. Med. Chem., 2007, 15, 3241. CrossRef
17. R. Islam and T. Nagamatsu, Heterocylces, 2006, 68, 2387. CrossRef
18. H. I. Ali, K. Tomita, E. Akaho, H. Kambara, S. Miura, H. Hayakawa, N. Ashida, Y. Kawashima, T. Yamagishi, H. Ikeya, F. Yoneda, and T. Nagamatsu, Bioorg. Med. Chem., 2007, 15, 242. CrossRef
19. T. Nagamatsu, R. Islam, and N. Ashida, Heterocylces, 2007, 72, 573. CrossRef
20. H. I. Ali, N. Ashida, and T. Nagamatsu, Bioorg. Med. Chem., 2007, 15, 6336. CrossRef
21. H. I. Ali, N. Ashida, and T. Nagamatsu, Bioorg. Med. Chem., 2008, 16, 922. CrossRef
22. R. Islam, N. Ashida, and T. Nagamatsu, Heterocylces, 2008, 76, 605. CrossRef
23. R. Islam, N. Ashida, and T. Nagamatsu, Tetrahedron, 2008, 64, 9885. CrossRef
24. A. R. Shrestha, T. Shindo, N. Ashida, and T. Nagamatsu, Bioorg. Med. Chem., 2008, 16, 8685. CrossRef
25. A. R. Shrestha, H. I. Ali, N. Ashida, and T. Nagamatsu, Bioorg. Med. Chem., 2008, 16, 9161. CrossRef
26. L. Birkofer and A. Ritter, Angew. Chem., 1965, 77, 414. CrossRef
27. G. Ritzmann and W. Pfleiderer, Chem. Ber., 1973, 106, 1401. CrossRef
28. W. Hutzenlaub, K. Kobayashi, and W. Pfleiderer, Chem. Ber., 1976, 109, 3217. CrossRef
29. G. Ritzmann, K. Ienaga, and W. Pfleiderer, Chem. Ber., 1977, 1217.
30. M. Hattori and W. Pfleiderer, Liebigs Ann. Chem, 1978, 1780.
31. A. Nagarajan, B. R. Meltsner, and T. J. Delia, J. Heterocycl. Chem., 1997, 34, 1581. CrossRef
32. P. Wang, L. J. Stuyver, K. A. Watanabe, A. Hassan, B.-K. Chun, and L. Hollecker, PCT Int. Appl, 2004, WO 2004013300.
33. K. Kobayashi and W. Pfleiderer, Chem. Ber., 1976, 109, 3159. CrossRef
34. S. Miura, Y. Yoshimura, M. Endo, H. Machida, A. Matsuda, M. Tanaka, and T. Sasaki, Cancer Lett., 1998, 129, 103. CrossRef
35. T. Mosmann, J. Immunol. Methods, 1983, 65, 55. CrossRef