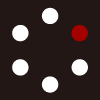
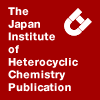
HETEROCYCLES
An International Journal for Reviews and Communications in Heterocyclic ChemistryWeb Edition ISSN: 1881-0942
Published online by The Japan Institute of Heterocyclic Chemistry
e-Journal
Full Text HTML
Received, 2nd July, 2009, Accepted, 10th August, 2009, Published online, 11th August, 2009.
DOI: 10.3987/COM-09-S(S)46
■ New Monomeric and Dimeric Hydrolyzable Tannins from Tamarix nilotica
Mohamed A. A. Orabi, Shoko Taniguchi, Morio Yoshimura, Takashi Yoshida, and Tsutomu Hatano*
Graduate School of Medicine, Dentistry and Pharmaceutical Sciences, Okayama University, 1-1-1 Tsushima-naka, Okayama 700-8530, Japan
Abstract
Two new ellagitannin monomers, nilotinins M2 (4) and M3 (5), two new dimers, nilotinins D4 (6), D5 (7), and a known dimer (8), were isolated from an aqueous acetone extract of dried leaves of Tamarix nilotica (Ehrenb.) Bunge. Structures of 4–7 were determined based on spectral data and chemical correlations with known tannins. The known dimer 8 was isolated from a plant extract for the first time; its 1H and 13C assignments are also reported here.INTRODUCTION
Early studies on tannins from tamaricaceous plants revealed the occurrence of a unique class of hydrolyzable tannins with vast structural diversity, including those with hellinoyl, dehydrodigalloyl (DHDG), isodehydrodigalloyl (isoDHDG), and macrocyclic structures.2–7 We have recently investigated tannin constituents of T. nilotica grown in Egypt (Tamaricaceae) and reported the isolation of a new ellagitannin monomer, nilotinin M1, three new dimers, nilotinins D1 (1), D2, and D3, and six known ellagitannins, hirtellin A, remurin A (2), remurin B, gemin D, hippomanin A, and 1,3-di-O-galloyl-4,6-O-(S)-hexahydroxydiphenoyl-β-D-glucose (3) (Figure 1).1 Continued investigation of tannins from this plant species led to the isolation of two additional unique ellagitannin monomers (4, 5), and three dimers (6–8) belonging to three different types of tannins. This paper deals with these five tannins.
RESULTS AND DISCUSSION
The eluate with 40% aq. MeOH from a Dia-ion HP-20 column of a concentrated extract of the dried leaves of T. nilotica was subjected to a combination of column chromatography on Toyopearl HW-40, Sephadex LH-20, and MCI-gel CHP-20P, followed by preparative reverse-phase HPLC, to afford ellagitannins (4–8) (Figures 2 and 3).
Structure of nilotinin M2
Nilotinin M2 (4) was isolated as an off-white amorphous powder. Its 1H NMR spectrum displayed a pair of m-coupled signals [δ 7.24, 6.62 (each 1H, d, J = 2.0 Hz)], and a 1H singlet (δ 7.05), which is the characteristic pattern for a DHDG unit.1,3 The spectrum also displayed a 2H singlet (δ 6.92), and two 1H singlets (δ 6.60 and 6.48), which indicate the presence of a galloyl and a hexahydroxydiphenoyl (HHDP) groups, respectively. 1-7 A system of seven aliphatic proton signals (δ 5.41–3.83) with large coupling constants (J1,2 = 8 Hz, J2,3 = J3,4 = J4,5 = 10 Hz) indicated a β-glucose core with a C1 conformation (Table 1).
Additionally, the aliphatic region of the spectrum also displayed a 3H singlet signal (δ 3.07), ascribable to a methoxy group. The high resolution (HR) ESIMS of 4 exhibited an [M + Na]+ ion peak, corresponding to a molecular formula of C42H32O27, consistent with the structural moieties. The presence of these constructing units of 4 was further confirmed by corresponding carbon peaks (Table 2 and experimental section) in the 13C NMR spectrum. A large difference in chemical shifts of the H-6 gem-protons (δ 5.23 and 3.83) indicated the location of the HHDP group at O-4/O-6 of the glucose core. An upfield shift (δ 4.00) of the resonance of the glucose anomeric proton relative to that of a related monomer, remurin A (2) (δ 5.53),1,3 suggested methylation, rather than galloylation, of the glucose O-1. A methoxy group at C-1 of
the glucose core was demonstrated by a heteronuclear multiple-bond coherence (HMBC) correlation of its proton signal (δ 3.07) with the glucose anomeric carbon peak (δ 102.9) and correlations of the glucose anomeric proton signal with both the glucose anomeric carbon and the methoxy carbon (δ 56.9). In the HMBC spectrum, correlations of the DHDG singlet signal [δ 7.05 (H-6’)] with the glucose H-2 signal (δ 5.15) via a common carbonyl carbon peak [δ 164.5 (C-7’)] substantiated the location of the DHDG unit at O-2 of the glucose core. On the other hand, correlations of the remaining proton signals [δ 7.24 (H-6) and 6.62 (H-2)] of the DHDG unit with a carboxyl carbon peak [δ 168.1 (C-7)], combined with the absence of correlation with any of the glucose proton signals to this carbon confirmed an identical orientation of the DHDG unit in 4 to that in 2. Similarly, the location of the galloyl group at O-3 of the glucose core was also evidenced by two HMBC correlations of the galloyl proton signal (δ 6.92) and the glucose H-3 signal (δ 5.41) with the common carbonyl carbon peak (δ 166.9). Correlations among the HHDP proton signals (δ 6.60 and 6.48) and the glucose H-4 (δ 5.00) and H-6 (δ 5.23) signals through the common ester carbonyl carbon peaks (δ 167.8) and (δ 168.4), respectively, were also observed (Figure 2). The HMBC spectrum also substantiated the assignments of the carbon signals in Table 2 and in the experimental section. The CD spectrum of 4 showed a positive Cotton effect in the short wavelength region, indicating the S configuration of the HHDP group.8 Based on these data, nilotinin M2 was assigned structure 4. The occurrence of methyl glucoside, the sugar core in nilotinin M2, as a natural compound in higher plants has been reported, 9,10 while 1-O-methyl derivative of a C-glycosidic tannin has been suggested to be an artifact. 11 Although nilotinin M2 may be an artifact, this is the first report of methylated ellagitannins in tamaricaceous plants.
Structure of nilotinin M3
Nilotinin M3 (5) was isolated as an off-white amorphous powder and exhibited an [M + Na]+ ion peak in HRESIMS, indicating its molecular formula to be C41H30O27. Its 1H NMR spectrum exhibited a 2H (7.16), and a 1H (δ 6.90) singlets, which are characteristic of an isoDHDG unit.1,4,5 The spectrum also showed the proton signals at δ 7.03 (2H, s; galloyl), and δ 6.60 and 6.47 (each 1H, s; HHDP) in the aromatic region.5 The presence of seven aliphatic signals in the range δ 5.87–3.82, with coupling constants identical to those of 4, indicated a β-glucose core with a C1 conformation (Table 1). The presence of these acyl units as well as the glucose core was also confirmed by their carbon signals shown in Table 2 and the experimental section. The appearance of the H-2 signal of the glucose core in the higher field (δ 4.00) relative to that of 4 (δ 5.15) indicates an unacylated OH-2 of the glucose core of 5. The 1H-1H COSY data for 5 revealed resonances of H-6 gem-protons at δ 5.26 and 3.81 indicating the location of the HHDP group at O-4/O-6 of the glucose core. In the 13C NMR spectrum of 5, a carbon peak at δ 150.7 was assigned to isoDHDG C-3 (C-5), because C-3 (C-5) of an isoDHDG unit resonates at lower field shift than the C-3 (C-5) of the usual galloyl (at around δ 145.7), which is attributed to the formation of the ether linkage at the p-hydroxyl group in the isoDHDG moiety.1 Consequently, a 2H singlet signal (δ 7.16) was assigned to the isoDHDG moiety (H-2, H-6) based on the two-bond HMBC correlation of the proton signal with the carbon peak (δ 150.7) of C-3 (C-5) of the same moiety (see the formula 5 in Figure 2).1 The correlations of this aromatic proton signal with the glucose H-1 signal (δ 5.87) via the common ester carbonyl carbon [δ 165.3 (C-7)] substantiated the location of the isoDHDG unit at O-1 of the glucose core. A carbonyl carbon peak (δ 170.7) was assigned to the isoDHDG unit (C-7’) based on the HMBC connectivity of its 1H singlet [δ 6.90 (H-6’) with this carbon signal. The absence of correlation between any of the glucose proton signals and this carbon confirmed the orientation of the isoDHDG unit, as shown in 5 (Figure 2). Placements of the galloyl and HHDP groups on the glucose core were further confirmed by the HMBC correlations, as represented by the arrows in Figure 2. A positive Cotton effect, [θ]237 +9.3×104 in the CD spectrum of 5, indicated the S configuration of the HHDP group. Based on these data, structure 5 was proposed for nilotinin M3. This is the first reported monomeric ellagitannin with an isoDHDG unit at the anomeric center of its glucose core.
Structure of nilotinin D4
Nilotinin D4 (6) was isolated as an off-white amorphous powder. Its molecular formula was determined to be C75H54O48 by HRESIMS. The 1H NMR spectrum of 6 exhibited proton signals δ 7.07, 7.04, 7.02, 6.97 (each 2H, s), and δ 6.77 (1H, s) attributable to three galloyl groups and an isoDHDG unit. Additionally, proton signals at δ 6.61, 6.60, 6.48, and 6.47 were assignable to two HHDP groups. In the aliphatic region of the spectrum, two seven-spin systems with large coupling constants (Table 1) were characteristic of two β-glucose cores with C1 conformations. The 1H-1H COSY data indicated that one (glucose-2) of these glucose cores was fully acylated, whereas the hydroxyl group at C-2 of the other (glucose-1) was unacylated, as indicated by the H-2 resonance at higher field (δ 4.03) relative to the corresponding signal of glucose-2. Two HHDP moieties in the molecule were assigned to be at O-4/O-6 of the glucose cores, in a way analogous to those in 4 and 5. The identical chemical shifts of the glucose-1 proton signals to those of nilotinin M3 (5; Table 1) suggested the presence of 5 in the dimeric structure of 6. Based on these data, structure 6 was assumed to be a structure isomeric to 1, through replacement of the DHDG linking unit in 1 with the isoDHDG in 6. Because modification of the less stable isoDHDG unit into the more stable DHDG one through the Smiles rearrangement under weak alkaline conditions has been reported for some hydrolyzable tannins,1,4,5 this reaction was applied to 6. Conversion of 6 into 1, as shown in the experimental section, satisfied structure 6 for nilotinin D4, including the S configuration of the HHDP units. Nilotinin D4 was presumed to be biologically derived from the co-existing monomers 3 (Figure 1) and 5 (Figure 2) through an esterification of the free carboxyl group of the isoDHDG unit of 5 with the glucose OH-2 of 3.
Structure of nilotinin D5
Nilotinin D5 (7) was isolated as an off-white amorphous powder. Its dimeric molecular formula was established as C68H50O44 by HRESIMS, along with the spectral data shown below. The aromatic region of the 1H NMR spectrum of 7 exhibited eight signals due to two galloyl groups [δ 7.13 and 7.03 (each 2H, s)], an HHDP group [δ 6.61 and 6.49 (each 1H, s)], and two isoDHDG units [δ 6.770 and 6.769 (each 2H, s), and 6.51 and 6.493 (each 1H, s)]. In the aliphatic region of the spectrum, signals of two glucose cores (Table 1) having β-configuration and adopting C1 conformations were seen. The 13C NMR spectrum exhibited aromatic, carbonyl, and aliphatic carbon peaks consistent with these units (see Table 2 and the experimental section). An HHDP unit in 7 locates O-4/O-6 of glucose-1, as indicated by the large chemical shift difference between H-6 methylene protons (δ 5.29 and 3.83), whereas OH-4 and OH-6 of glucose-2 were assigned to be unacylated based on the upfield shifts (δ 3.75–3.91) of H-4 and H-6. Thus, the remaining acyl groups should be at O-1–O-3 of each glucose core. The HMBC spectrum of 7 showed correlations of galloyl proton signals (δ 7.13 and 7.03) with signals of H-3 of glucose-2 (δ 5.32) and H-3 of glucose-1 (δ 5.73) through the ester carbonyl carbons (δ 167.3 and 167.0), respectively. Consequently, 7 was assigned to have a macrocyclic structure in which each of the two isoDHDG units links between O-1 of one glucose core and O-2 of the other glucose core. The mode of the attachments of the isoDHDG units in 7 was confirmed by the HMBC connectivities of two 2H singlet signals (δ 6.769 and 6.770) of the isoDHDG units (H-2, H-6) with H-1 of glucose-1 (δ 6.05) and H-1 of glucose-2 (δ 5.94) through the common ester carbonyl carbons (δ 164.8 and 165.2) (C-7), respectively. Similarly, connectivities of the two 1H singlet signals (δ 6.51 and 6.493) of the isoDHDG units (H-6’) with H-2 of glucose-2 (δ 5.32) and H-2 of glucose-1 (δ 5.531) through the respective ester carbonyl carbons (δ 166.7 and 166.8) (C-7’) were consistent with this attachment mode. The other HMBC correlations explained by the arrows in Figure 3 were also consistent with the proposed structure. The atropisomerism of the chiral HHDP group in 7 was determined to be S based on the strong, positive Cotton effect at 236 nm in the CD spectrum. Nilotinin D5 is therefore represented by structure 7, which corresponds to a des-HHDP derivative of tamarixinin B (9) 5 (Figure 4), where O-4/O-6 of one of the glucose cores are unacylated. The Mr of 7, demonstrated by an [M + Na]+ ion peak at m/z 1593, was 302 mass units (HHDP) less than 9. This is consistent with the structural correlation.
The isoDHDG 1H singlets (H-6’) in 7 resonated at δ 6.51 and 6.493, which is shifted upfield (ca. 0.4 ppm) relative to the corresponding proton signals of the isoDHDG units in the linear tannins 5, 6, and tamarixinin C (10) (Figure 4) (δ 6.84).5 Further, the corresponding protons of the isoDHDG units in 9 also showed analogous upfield shifts (δ 6.54).5 The upfield shifts of the isoDHDG 1H singlets in 7 and 9 could be attributed to the shielding effect of the aromatic ring, bearing two protons, of the isoDHDG units in these macrocyclic structures.
Structure of nilotinin D6
Nilotinin D6 (8) was isolated as an off-white amorphous powder, for which the molecular formula, C68H52O44, was established by HRESIMS, along with the spectral data below. The 1H NMR spectrum of 8 showed the presence of four galloyl groups [δ 7.028, 6.97, 6.94, 6.93 (each 2H, s)], a DHDG unit [δ 7.11 and 6.37 (each 1H, d, J = 2Hz), and 7.027 (1H, s)], an HHDP group [δ 6.58 and 6.50 (each 1H, s)], and two glucose cores in the β-configuration and the C1 confirmation (Table 1). These are the same structural units as in hirtellin G (11) (Figure 4).6 The 13C NMR spectral data (Table 2 and experimental section) of 8 are consistent with these units. The 1H-1H COSY data listed in Table 1 indicated the location of the HHDP group at O-4/O-6 of glucose-2. The HMBC spectrum of 8 exhibited correlations of the DHDG proton signals [δ 7.11, 6.37 (each 1H, d) and 7.027 (1H, s)] and the galloyl-C (see formula in Figure 3) proton signal (δ 6.97) with H-1 of glucose-1 (δ 5.90), H-2 of glucose-2 (δ 5.521) and H-1 of glucose-2 (δ 5.44) through a non-differentiated cluster of ester carbonyl carbon peaks (δ 164.7, 3C). Resonance of the glucose-2 anomeric proton showed an upfield shift (δ 5.44) relative to that of glucose-1 (δ 5.90), which is explained by the anisotropic effect of the galloyl part, bearing an isolated proton (H-6’), of the DHDG unit at O-2 of glucose-2.3 The binding mode of the DHDG unit in 8 was thus determined to be identical to that in 11. Other important HMBC correlations are shown by the arrows in Figure 3 and are consistent with structure 8. The CD spectrum of 8 indicated the S configuration of the HHDP group. Based on these data, structure 8, a regioisomer of 11 concerning the position of the HHDP group, was assigned for nilotinin D6. Although the 1H NMR spectral data for 8 are identical to those reported for a hydrolysate from hirtellin A (hydrolysate-2),6 its physicochemical data, including [α]D, UV, and CD, as well as the detailed aromatic proton and full carbon assignments, are reported for the first time in this paper.
Similar to other tamaricaceous plants,2-7 T. nilotica produces DHDG, isoDHDG, and macrocyclic ellagitannins. This emphasizes the chemotaxonomic importance of these tannins.
EXPERIMENTAL
General procedures. HRESIMS were performed using a Bruker MicroTOF-Q spectrophotometer with positive ion mode. FABMS was performed using an Auto Spec OA-Tof spectrophotometer. m-Nitrobenzyl alcohol was used as the matrix agent. Normal-phase HPLC was conducted on a YMC-Pack SIL A-003 (YMC, Japan) column (4.6 i.d. × 250 mm) developed with n-hexane– MeOH–tetrahydrofuran–formic acid (47:39:13:1) containing oxalic acid (450 mg/L; flow rate, 1.5 mL/min; 280 nm UV detection) at room temperature. Analytical reverse-phase HPLC was performed on a YMC-Pack ODS-A A-303 (YMC) column (4.6 i.d. × 250 mm) developed with 0.01 M H3PO4–0.01 M KH2PO4–MeOH (2:2:1; flow rate, 1 mL/min; 280 nm UV detection) at 40 ºC. Preparative reverse-phase HPLC was performed on a YMC-Pack ODS-A A-324 (YMC) column (10 i.d. × 300 mm) developed with 0.01 M H3PO4–0.01 M KH2 PO4–MeOH (2:2:1; flow rate, 2 mL/min; 280 nm UV detection) at 40 ºC. The plant materials (T. nilotica), other instruments, and chromatographic methods used in this study have been described previously.1
Extraction and isolation. The 40% MeOH fraction (10.4 g), previously obtained from a 70% aq. acetone homogenate of the dried leaves of T. nilotica (200 g), was chromatographed over a Toyopearl HW-40 column (coarse grade, 2.2 i.d. × 43 cm) with 70% EtOH, 70% EtOH-70% acetone (9:1→ 8:2→ 7:3→ 5:5, v/v), and 70% acetone, collecting 1000-drop fractions (Tfrs). Separation of the respective fractions was achieved by monitoring either normal- or reverse-phase HPLC. The 70% EtOH eluate (Tfrs. 70-97, 240 mg) was applied to MCI gel CHP-20P column (1.1 i.d. × 22 cm) eluted with aqueous MeOH (10% → 20% → 25% → 30% → 40%, v/v). Nilotinin D6 (8) (2 mg) was purified from the 25% MeOH eluates (26 mg) by preparative HPLC. Tfrs. 98–190 (1.7 g) was further chromatographed over Sephadex LH-20 column (2.2 i.d. × 32 cm) with 70% EtOH, collecting 700-drop fractions, and yielded Sfrs. Sfrs. 46–59 (216 mg) was rechromatographed on the same column with 70% EtOH followed by preparative HPLC purification to give nilotinin D5 (7) (4 mg). Tfrs. 191–218 (285 mg) was subjected to column chromatography over MCI gel CHP-20P (1.1 i.d. × 35 cm) eluted with H2O→ aqueous MeOH (10% → 20% → 30% →40%, v/v). The 40% MeOH eluate was purified by preparative HPLC giving nilotinin D4 (6) (2 mg). In a separate experiment and guided by the first one, extraction and fractionation for the same dried leaves (1 kg) was carried out. Nilotinins M2 (4) (7.2 mg) and M3 (5) (15 mg) were purified from a MCI-gel fraction (20% MeOH eluate from MCI chromatography of the 70% EtOH eluate from the Toyopearl chromatography) by preparative HPLC. Additionally, enrichment of 7 (7 mg) and 8 (4 mg) from the corresponding fractions was achieved.
Nilotinin M2 (4): An off-white amorphous powder, [α]D27 +31.2 (c 1.0, MeOH). FABMS m/z: 991 [M + Na]+. HRESIMS m/z: 991.10463 [M + Na]+ (calcd. for C42H32O27 + Na, 991.10232). UV λ max (MeOH) nm (log ε): 220 (4.90), 273 (4.56). CD (MeOH): [θ]237 +8.2×104, [θ]265 –3.2×104, [θ]283 +2.2×104. 1H NMR (acetone-d6–D2O, 9:1) δ: 7.24 (1H, d, J = 2.0 Hz, DHDG H-6), 7.05 (1H, s, DHDG H-6’), 6.92 [2H, s, galloyl H-2,H-6), 6.62 (1H, d, J = 2.0 Hz, DHDG H-2), 6.60 (1H, s, HHDP H-3), 6.48 (1H, s, HHDP H-3’), 3.07 (3H, s, -OCH3), glucose protons (Table 1). 13C NMR (acetone-d6–D2O, 9: 1) δ: 56.9 (-OCH3), 107.1 (DHDG C-2), 107.7 (HHDP C-3’), 107.8 (HHDP C-3), 110.1 (2C, galloyl C-2, C-6), 110.2 (DHDG C-6’), 111.9 (DHDG C-6), 113.8 (DHDG C-1’), 115.6 (HHDP C-1), 115.8 (HHDP C-1’), 120.0 (galloyl C-1), 121.9 (DHDG C-1), 125.5, 126.1 (HHDP C-2, C-2’), 136.2 (HHDP C-5), 136.5 (HHDP C-5’), 136.6 (DHDG C-2’), 139.1 (DHDG C-4), 139.3 (galloyl C-4), 140.4 ( DHDG C-3’), 140.5 (DHDG C-4’), 143.4 (DHDG C- 5’), 144.3 (2C, HHDP C-6, C-6’), 145.1, 145.2 (HHDP C-4, C-4’), 145.7 (2C, galloyl C-3, C-5), 146.2 (DHDG C-5), 148.0 (DHDG C-3), 164.6 (DHDG C-7’), 166.9 (galloyl C-7), 167.8 (HHDP C-7’), 168.1 (DHDG C-7), 168.4 (HHDP C-7), glucose carbons (Table 2).
Nilotinin M3 (5): An off-white amorphous powder, [α]D25 –5.4 (c 1.0, MeOH). FABMS m/z: 977 [M + Na]+. HRESIMS m/z: 977.08857 [M + Na]+ (calcd. for C41H30O27 + Na 977. 08667). UV λ max (MeOH) nm (log ε): 217 (5.05), 266 (4.70). CD (MeOH): [θ]237 +9.3×104, [θ]261 –3.2×104, [θ]285 +1.6×104; 1H NMR (acetone-d6–D2O, 9:1) δ: 7.16 (2H, s, isoDHDG H-2, H-6), 7.03 (2H, s, galloyl H-2, H-6), 6.90 (1H, s, isoDHDG H-6’), 6.60 (1H, s, HHDP H-3), 6.47 (1H, s, HHDP H-3’), glucose protons (Table 1). 13C NMR (acetone-d6–D2O, 9: 1) δ: 107.7 (HHDP C-3’), 107.8 (HHDP C-3), 108.2 (isoDHDG C-6’), 110.1 (2C, galloyl C-2, C-6), 110.8 (2C, isoDHDG C-2, C-6), 115.0 (isoDHDG C-1’), 115.7, 115.8 (HHDP C-1, C-1’), 120.8 (galloyl C-1), 125.4 (isoDHDG C-1), 125.7, 126.1 (HHDP C-2, C-2’), 136.2 (HHDP C-5), 136.4 (HHDP C-5’), 139.0 (galloyl C-4), 139.1 (isoDHDG C-4’), 139.61 (isoDHDG C-4), 139.4, 139.65 (isoDHDG C-2’, C-3’), 142.3 (isoDHDG C-5’), 144.27, 144.29 (HHDP C-6, C-6’), 145.13 (HHDP C-4’), 145.15 (HHDP C-4), 145.7 (2C, galloyl C-3, C-5), 150.7 (2C, isoDHDG C-3, C-5), 165.3 (isoDHDG C-7), 167.1 (galloyl C-7), 167.9 (HHDP C-7’), 168.3 (HHDP C-7), 170.7 (isoDHDG C-7’), glucose carbons (Table 2).
Nilotinin D4 (6): An off-white amorphous powder, [α]D24 +11.8 (c 1.0, MeOH). FABMS m/z: 1745 [M + Na]+. HRESIMS m/z: 1745.16764 [M + Na]+ (calcd. for C75H54O48 + Na, 1745.16767). UV λ max (MeOH) nm (log ε): 220 (5.37), 274 (5.09). CD (MeOH): [θ]237 +3.2×105, [θ]263 –8.5×104, [θ]287 +3.8×104. 1H NMR (acetone-d6–D2O, 9: 1) δ: 7.07, 7.04, 7.02, 6.97 [each 2H, s, galloyl (H-2, H-6) × 3 and isoDHDG (H-2, H-6)], 6.77 (1H, s, isoDHDG H-6’), 6.61, 6.60, 6.48, 6.47 [each 1H, s, HHDP (H-3, H-3’) × 2], glucose protons (Table 1).
Nilotinin D5 (7): An off-white amorphous powder, [α]D26 +11.2 (c 1.0, MeOH). ESIMS m/z: 1593 [M + Na]+. HRESIMS m/z: 1593.15579 [M + Na]+ (calcd. for C68H50O44 + Na, 1593.15672). UV λ max (MeOH) nm (log ε): 218 (5.38), 276 (5.04). CD (MeOH): [θ]236 +1.5×105, [θ]265 –6.0×104, [θ]294 +3.0×104; 1H NMR (acetone-d6–D2O, 9: 1) δ: 7.13 [2H, s, galloyl-B (H-2, H-6)], 7.03 [2H, s, galloyl-A (H-2, H-6)], 6.770, 6.769 [each 2H, s, isoDHDG (H-2, H-6) × 2], 6.61 (1H, s, HHDP H-3), 6.51 (1H, s, isoDHDG-A H-6’), 6.493 (1H, s, isoDHDG-B H-6’), 6.49 (1H, s, HHDP H-3’), glucose protons (Table 1). 13C NMR (acetone-d6–D2O, 9: 1) δ: 107.2, 107.3 [isoDHDG (C-6’) × 2], 107.7 (HHDP C-3’), 107.9 (HHDP C-3), 110.0 [2C, galloyl-B (C-2, C-6)], 110.1 [2C, galloyl-A (C-2, C-6)], 110.2, 110.6 [each 2C, isoDHDG (C-2, C-6) × 2], 115.6 (HHDP C-1), 116.0 (HHDP C-1’), 116.1, 116.3 [isoDHDG (C-1’) × 2], 120.1 (galloyl-A C-1), 120.8 (galloyl-B C-1), 122.8, 123.2 [isoDHDG (C-1) × 2], 125.4, 126.1 (HHDP C-2, C-2’), 136.3 (HHDP C-5), 136.6 (HHDP C-5’), 138.4 [2C, isoDHDG (C-4’) × 2], 138.5 [2C, isoDHDG (C-4) × 2], 138.6, 138.7, 139.6, 139.7, 141.5, 141.6 [isoDHDG (C-2’, C-3’, C-5’) × 2], 139.2 (galloyl-B C-4), 139.4 (galloyl-A C-4), 144.3 (2C, HHDP C-6, C-6’), 145.2 (2C, HHDP C-4, C-4’), 145.8 [2C, galloyl-A (C-3, C-5)], 145.9 [2C, galloyl-B (C-3, C-5)], 149.0 [4C, isoDHDG (C-3, C-5) × 2], 164.8 (isoDHDG-A C-7), 165.2 (isoDHDG-B C-7), 166.7 (isoDHDG-A C-7’), 166.8 (isoDHDG-B C-7’), 167.0 (galloyl-A C-7), 167.3 (galloyl-B C-7), 167.8 (HHDP C-7’), 168.3 (HHDP C-7), glucose carbons (Table 2).
Nilotinin D6 (8): An off-white amorphous powder, [α]D23 +83.1 (c 1.0, MeOH). ESIMS m/z: 1595 [M + Na]+. HRESIMS m/z: 1595.17064 [M + Na]+ (calcd. for C68H52O44 + Na, 1595.17237). UV λmax (MeOH) nm (log ε): 218 (5.17), 278 (4.84). CD (MeOH): [θ]238 +1.0×105, [θ]263 –1.0×104, [θ]286 +2.7×104. 1H NMR (acetone-d6–D2O, 9: 1) δ: 7.11 (1H, d, J = 2 Hz, DHDG H-6), 7.028 (2H, s, galloyl-B H-2, H-6), 7.027 (1H, s, DHDG H-6’), 6.97 (2H, s, galloyl-C H-2, H-6), 6.94 (2H, s, galloyl-D H-2, H-6), 6.93 (2H, s, galloyl-A H-2, H-6), 6.58 (1H, s, HHDP H-3), 6.50 (1H, s, HHDP H-3’), 6.37 (1H, d, J = 2 Hz, DHDG H-2), glucose protons (Table 1). 13C NMR (acetone-d6–D2O, 9: 1) δ: 106.9 (DHDG C-2), 107.8 (HHDP C-3’), 107.9 (HHDP C-3), 110.1 (DHDG C- 6’), 109.9, 110.0, 110.2, 110.3 [each 2C, galloyl (C-2, C-6) × 4], 112.7 (DHDG C-6), 113.4 (DHDG C-1’), 115.7 (HHDP C-1), 115.9 (HHDP C-1’), 119.1, 119.84, 119.87, 120.1, 120.8 [DHDG C-1, and galloyl (C-1) × 4], 125.4, 126.0 (HHDP (C-2, C-2’), 136.3 (HHDP C-5), 136.6 [2C, HHDP C-5’, DHDG C-2’], 139.0, 139.25, 139.34, 139.8 (2C) [galloyl (C-4) × 4 and DHDG C-4], 140.2, 140.7 (DHDG C-3’, C-4’), 143.4 (DHDG C-5’), 144.4 [2C, HHDP (C-6, C-6’)], 145.1, 145.2 [HHDP (C-4, C-4’)], 145.7, 145.77, 145.78, 145.81 [each 2C, galloyl (C-3, C-5) × 4] 145.9 (DHDG C-5), 147.8 (DHDG C-3), 164.7 [3C, DHDG (C-7, C-7’) and galloyl-C (C-7)], 166.2 (galloyl-A C-7), 166.77 (galloyl-B C-7), 166.82 (galloyl-D C-7), 167.8 (HHDP C-7’), 168.3 (HHDP C-7), glucose carbons (Table 2).
Isomerization of nilotinin D4 (6) into nilotinin D1 (1): A solution of 6 (0.7 mg) in a phosphate buffer (pH 7.4; 200 μL) was incubated for 1 h at 40 °C. The reaction mixture was acidified with 1 N HCl and passed through a Sep-Pak C18 cartridge. After the cartridge was washed with water, the adsorbed material was recovered with MeOH. The MeOH was evaporated to give 1 (0.5 mg), which was determined by co-chromatographic analyses with samples isolated from the plant on normal-phase and reverse-phase HPLC, [α]D, and the 1H-NMR spectral comparison.
ACKNOWLEDGEMENTS
We thank Prof. Mo’men Mostafa Zareh, Department of Botany, Assiut University, for identification of the plant. The NMR instrument used in this study is the property of the SC-NMR Laboratory of Okayama University. The first author thanks the Egyptian Government (Ministry of Higher Education and State for Scientific Research) for his scholarship.
References
1. Part 2 in the series “Hydrolyzable tannins of tamaricaceous plants”. See part 1, M. A. A. Orabi, S. Taniguchi, and T. Hatano, Phytochemistry, in press.
2. T. Yoshida, T. Hatano, A. F. Ahmed, A. Okonogi, and T. Okuda, Tetrahedron, 1991, 47, 3575. CrossRef
3. T. Yoshida, A. F. Ahmed, M. U. Memon, and T. Okuda, Chem. Pharm. Bull., 1991, 39, 2849.
4. T. Yoshida, A. F. Ahmed, and T. Okuda, Chem. Pharm. Bull., 1993, 41, 672.
5. T. Yoshida, A. F. Ahmed, M. U. Memon, and T. Okuda, Phytochemistry, 1993, 33, 197. CrossRef
6. A. F. Ahmed, T. Yoshida, and T. Okuda, Chem. Pharm. Bull., 1994, 42, 246.
7. A. F. Ahmed, T. Yoshida, M. U. Memon, and T. Okuda, Chem. Pharm. Bull., 1994, 42, 254.
8. T. Okuda, T. Yoshida, T. Hatano, T. Koga, N. Toh, and K. Kuriyama, Tetrahedron Lett., 1982, 23, 3937. CrossRef
9. S. Aubert, P. Choler, J. Pratt, R. Douzet, E. Gout, and R. Bligny, J. Exp. Bot., 2004, 55, 2179. CrossRef
10. K. Ichimura, K. Kohata, M. Koketsu, Y. Yamaguchi, H. Yamaguchi, and K. Suto, Biosci. Biotech. Biochem., 1997, 61, 1734.
11. T. Yoshida, H. Ohbayashi, K. Ishihara, W. Ohwashi, K. Haba, Y. Okano, T Shingu, and T. Okuda, Chem. Pharm. Bull., 1991, 39, 2233.