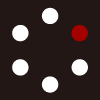
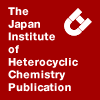
HETEROCYCLES
An International Journal for Reviews and Communications in Heterocyclic ChemistryWeb Edition ISSN: 1881-0942
Published online by The Japan Institute of Heterocyclic Chemistry
e-Journal
Full Text HTML
Received, 27th July, 2009, Accepted, 31st August, 2009, Published online, 2nd September, 2009.
DOI: 10.3987/COM-09-S(S)78
■ Synthesis of 6-Hydroxymethylpterin α- and β-D-Glucosides
Tadashi Hanaya,* Hiroki Baba, Kazumasa Ejiri, and Hiroshi Yamamoto
Graduate School of Natural Science and Technology, Okayama University, 3-1-1 Tsushima-naka, Okayama 700-8530, Japan
Abstract
The key precursor, N2-(N,N-dimethylaminomethylene)-6-hydroxymethyl-3-[2-(4-nitrophenyl)ethyl]pterin (11) was efficiently prepared from 2,5,6-triamino-4-hydroxypyrimidine (8) in 5 steps. The first, unequivocal synthesis of 6-hydroxymethylpterin α-D-glucoside (6a) was achieved by treatment of 11 with 4,6-di-O-acetyl-2,3-di-O-(4-methoxybenzyl)-α-D-glucopyranosyl bromide (16) in the presence of tetraethylammonium bromide and N-ethyldiisopropylamine, followed by removal of the protecting groups, while 6-hydroxymethylpterin β-D-glucoside (6b) was prepared by means of selective glycosylation of 11 with 2,3,4,6-tetra-O-benzoyl-α-D-glucopyranosyl bromide (12) in the presence of silver triflate and tetramethylurea.INTRODUCTION
Certain pterin glycosides carrying various kinds of sugars attached to the side-chain at C-6 of the pteridine ring were found to be produced by some prokaryotes as exemplified by glycosides of biopterin (1): 2’-O-(α-D-glucopyranosyl)biopterin (2)1-4 and its β-D-ribofuranosyl analog (3)5 isolated from various cyanobacteria and 2'-O-(2-acetamido-2-deoxy-β-D-glucopyranosyl)biopterin (4)6 from a green sulfur photosynthetic bacterium. As for glycosides of other pterins, 2-acetamido-2-deoxy-β-D-glucoside of ciliapterin (tepidopterin)7 and 2-amino-2-deoxy-α-D-glucoside of neopterin (solfapterin)8 were isolated from a green sulfur photosynthetic bacterium and a thermophilic archaebacterium, respectively, whereas
glycosides of 6-hydroxymethylpterin (5a), such as D-glucoside (6)9,10 and cyanopterin (7),11 were found in cyanobacterium Synechocysts sp.
As shown in these examples, anomeric structures of some pterin glycosides are α-type and those of others are β-type depending on the combination of the pterin and sugar moieties. Despite a considerable interest from the viewpoint of their biological activities and functions,12 attempts at preparation of natural pterin glycosides including selective glycosylation have so far scarcely been made, except for our synthetic studies on biopterin and ciliapterin glycosides.5,13-15 Therefore we undertook to explore an efficient protocol for selective α- and β-glycosilation of pterin derivatives by employing 6-hydroxymethylpterin (5a) as a model pterin substrate. In addition, we give herein the first synthesis of a natural pterin glycoside, 6-[(D-glucopyranosyloxy)methyl]pterin (6).
RESULTS AND DISCUSSION
Although the preparation of 6-hydroxymethylpterin (5a) has been achieved by the condensation of 2,5,6-triamino-4-hydroxypyrimidine dihydrochloride (8) with dihydroxyacetone and the subsequent oxidation,16 formation of 7-hydroxymethyl isomer (5b) and the detailed regioselectivity of the condensation have not been reported because 5a was isolated by repeated recrystallizations. We therefore checked the selectivity of the pteridine-ring formation by treatment of compound 8 with dihydroxyacetone in the presence of sodium acetate, followed by air-oxidation (Scheme 1). The resulting products were confirmed by 1H NMR spectrum to be an 82:18 mixture consisting of 5a and 5b. On the contrary, the same condensation in an aqueous sodium bicarbonate solution resulted in the predominant formation of the 7-subsituted pterin (5b) in a ratio of 11:89. The reversal of the selectivity is most likely caused by the condensation of 8 with glyceraldehyde transformed from dihydroxyacetone under the basic conditions.
Due to the effectively stabilized intramolecular hydrogen bonding in the solid state,17 pterin derivatives including 1 and 5a are generally little soluble in nonpolar aprotic solvents used for conventional glycosylation. To overcome this problem, we tried to introduce appropriate protecting groups into 5a: N,N-dimethylaminomethylene group for 2-amino and 2-(4-nitrophenyl)ethyl (NPE) group for N(3) of the ring.18 Thus, treatment of the 82:18 mixture of 5a,b with N,N-dimethylformamide dimethyl acetal in DMF, followed by acetylation of a hydroxy group, afforded 6-acetoxymethyl-N2-(N,N-dimethylaminomethylene) derivatives (9a,b), whose N(3) position was then protected with NPE group by Mitsunobu reaction with 2-(4-nitrophenyl)ethanol in the presence of triphenylphoshine and diethyl azodicarboxylate (DEAD) to give the fully protected products 10a and 10b. These were then separated by column chromatography over silica gel into the desired 6-acetoxymethyl derivative (10a) (46% overall yield from 8) and the 7-acetoxymethyl isomer (10b) (10%).
The structural assignments of 10a and 10b were made primarily on the basis of their 13C-NMR spectral data (see, Experimental). The signals of C-6 and C-7 of 6-alkylpteridines generally appear at a similar field, whereas C-7 signals of 7-alkyl derivatives shifts to a lower field (ca. 20 ppm) from those of C-6.14,19 Therefore, the close values of 10a (C-6: δ 147.35, C-7: δ 149.48) and the distant values of 10b (C-6: δ 139.28, C-7: δ 158.12) indicate the 6-substituted pterin for the former and the 7-substituted pterin for the latter.
Methanolysis of 6-acetoxymethylpterin derivative (10a) in the presence of sodium methoxide provided the 6-hydroxymethyl derivative (11), a versatile precursor for glycosylation. Glycosylation of 11 with two glycosyl donors (12 and 16) in chloroform20 was then extensively investigated under various conditions in the presence of activators (Scheme 2, Table 1).
Glycosylation of 11 with 2,3,4,6-tetra-O-benzoyl-α-D-glucopyranosyl bromide (12)21 (3.0 mol equiv.) in chloroform at room temperature in the presence of tin(IV) chloride (2.0 mol equiv.)5,13 did not proceed apparently due to deposition of the substrate, whereas the same reaction under reflux conditions resulted in the formation of unidentified, decomposed compounds (Entries 1,2). On the other hand, while treatment of 11 with 12 at room temperature in the presence of siver triflate (2.0 mol equiv.) and tetramethylurea (TMU) (1.0 mol equiv.)22 scarcely proceeded, efficient glycosylation of 11 was attained by refluxing the same reaction mixture after having been left at room temperature to generate an acyloxonium ion intermediate, furnishing 6-O-[(2,3,4,6-tetra-O-benzoyl-β-D-glucopyranosyloxy)methyl]pterin derivative (13) in 63% yield (Entries 3,4). Use of larger amounts of the glycosyl donor (4.5 equiv.) and the activator (4.0 equiv.) brought about production of 6-benzoyloxymethylpterin derivative (15) (Entry 5). Refluxing of 11 with 12 in chloroform in the presence of tetraethylammonium bromide (2.0 mol equiv.) and N-ethyldiisopropylamine (2.0 mol equiv.)23 afforded α-D-glucopyranose-1,2-orthobenzoate derivative (14) (in 93% yield) instead of the pterin glycoside (Entry 6).
A possible pathway for the formation of 14 and 15 from the glycosyl donor (12) is illustrated in Scheme 3. Namely, the tetra-O-benzoylglucopyranosyl bromide (12) reacts with the glycosyl accepter (11) under Koenigs-Knorr conditions to initially yield a 1,2-orthobenzoate intermediate (B) via acyloxonium ion (A).24 Although the intermediate (B) isomerizes to yield the thermodynamic product, 1,2-trans-glycoside (13) by the action of Lewis acid, the presence of an amine is likely to prompt the intermediate (B) to cause deprotonation leading to the production of 1,2-orthobenzoate derivative (14). Meanwhile, formation of benzoylated alcohol (15) may result from an alternative pathway involving intramolecular rearrangement from the intermediate C, which was supported by the fact of recovery of 2-O-debenzoylated compound (D) from the reaction mixture.
As the stereoselective formation of the β-glycoside (13) from 11 was mainly caused by participation of the 2-O-benzoyl group of the glycosyl donor (12) through the formation of an acyloxonium ion intermediate, glycosylation of 11 was then examined by use of a glycosyl donor whose 2-OH was protected with an ether substituent having no neighboring group participation. 4,6-Di-O-acetyl-2,3-di-O-(p-methoxybenzyl)-α-D-glucopyranosyl bromide (16),15 a novel glycosyl donor developed for preparation of pterin α-glycoside, was thus employed.
Glycosylation of 11 with the glycosyl donor (16) in chloroform in the presence of siver triflate (2.0 mol equiv.) and tetramethylurea (TMU) (1.0 mol equiv.) scarcely proceeded at room temperature, whereas the same reaction under reflux conditions resulted in the formation of decomposed compounds (Entries 7,8). The carboxonium ion intermediate derived from 16 having no stabilization by the neighboring 2- O-(p-methoxybenzyl) group suggests the facile decomposition at a higher temperature. While treatment of 11 with 16 in the presence of tetraethylammonium bromide (2.0 mol equiv.) and N-ethyldiisopropylamine (2.0 mol equiv.) facilitated no glycosylation at room temperature, the same reaction under refluxing conditions resulted in the formation of 6-[(2,3,4,6-tetra-O-benzoyl-α-D-glucopyranosyloxy)methyl]pterin derivative (17) as a sole product (69% yield) (Entries 9,10). The α-anomeric structure of 17 was derived from its J1,2 value (3.5 Hz) of 1H-NMR in comparison with the larger J1,2 value (8.0 Hz) of the β-form 13.
Removal of the protecting groups of β-D-glucoside (13) was performed by the three successive treatment with sodium methoxide (to cleave benzoyl groups), aqueous ammonia (to cleave the N,N-dimethylaminomethylene group), and DBU (to cleave the NPE group)18 to furnish 6-hydroxymethylpterin β-D-glucopyranoside (6b) in 87% overall yield (Scheme 4).
Similarly, removal of the protecting groups of α-D-glucoside (17) was conducted by the following 4-step-procedures: cleavage of PMB by use of DDQ, followed by acetylation, afforded the 2,3,4,6-tetra-O-acetyl-α-D-glucopyranosyl derivative (18), which was treated with aqueous ammonia and then with DBU, thus providing 6-hydroxymethylpterin α-D-glucopyranoside (6a) in 74% overall yield from 17. Structures of 6a and 6b were unambiguously established as the corresponding pentaacetyl derivatives (19a,b) obtained by usual acetylation. Treatment of 19a and 19b with aqueous ammonia respectively regenerated 6a and 6b quantitatively. The precise 1H- and 13C-NMR parameters of 6a,b are summarized in Table 2.
The present work thus demonstrates an effective way for both selective α- and β-glycosylation of pterin derivatives. By employing these synthetic procedures, the first synthesis of 6-hydoxymethylpterin α- and β-D-glucoside (6a,b) was unequivocally achieved. Extension of this work including improvement of yield for glycosylation as well as applications of these findings in synthesizing other natural pterin glycosides is in progress.
EXPERIMENTAL
All reactions were monitored by TLC (Merck Silica gel 60 F254) with an appropriate solvent system [(A) AcOEt, (B) 1:9 MeOH-CHCl3, and (C) 5:3:1 2-PrOH-AcOEt-H2O]. Column chromatography was performed with Daiso Silica Gel IR-60/210w. Components were detected by exposing the plates to UV light and/or 20% H2SO4-EtOH, with subsequent heating. The UV/Vis spectra were taken on a JASCO V-530 spectrophotometer. Optical rotations were measured with a JASCO P-1020 polarimeter. The NMR spectra were measured in CDCl3 with Varian Unity Inova AS600 (600 MHz for 1H, 151 MHz for 13C) at 23 °C, unless otherwise stated. The solvent peak was used as an internal standard for chemical shifts: in CDCl3, δ 7.26 for 1H, 77.00 for 13C; in DMSO-d6, δ 2.50 for 1H, 39.70 for 13C. The assignments of 13C signals were made with the aid of 2D C-H COSY measurements.
6-Hydroxymethylpterin (5a)24 and 7-hydroxymethylpterin (5b).
A. With AcONa. The following modification of the literature procedures16 was made. To a solution of 2,5,6-triamino-4-hydroxypyrimidine dihydrochloride (8) (800 mg, 3.74 mmol) and L-cystein hydrochloride monohydrate (657 mg, 3.74 mmol) dissolved in 4M aqueous sodium acetate (40 mL), was added a solution of dihydroxyacetone (1.01 g, 11.2 mmol) in water (10 mL). After stirring at rt for 2 h, air was introduced into the mixture at the same temperature for 24 h. The mixture was cooled at 0 °C and then the precipitates were collected by filtration and washed with cold water. The precipitates were suspended in hot water (30 mL) and then 1M aqueous NaOH was added dropwide. The mixture was treated with active charcoal and filtered. The filtrate was cooled to rt and then the pH was adjusted to 6.0 with 1M HCl. After cooling to 0 °C, the precipitates were collected by filtration, washed with cold water and methanol, and then dried under reduced pressure to give an 82:18 mixture (580 mg, 80%) of 5a and 5b as a yellow solid: Rf = 0 (B). 1H NMR for 5a (DMSO-d6) δ 4.61 (2H, d, JCH2,OH = 5.7 Hz, CH2-6), 5.56 (1H, t, OH), 6.90 (2H, br s, H2N-2), 8.72 (1H, s, H-7), 11.44 (1H, br s, H-N(3)); 1H NMR for 5b (DMSO-d6) δ 4.61 (2H, d, JCH2,OH = 5.9 Hz, CH2-7), 5.66 (1H, t, OH), 6.90 (2H, br s, H2N-2), 8.47 (1H, s, H-6), 11.44 (1H, br s, H-N(3)) .
B. With NaHCO3. Compound 8 (200 mg, 0.934 mmol), L-systein hydrochloride monohydrate (164 mg, 0.934 mmol), and dihydroxyacetone (252 mg, 2.80 mmol) were dissolved in 1M aqueous sodium bicarbonate (10 mL). After stirring at rt for 2 h, the mixture was subjected to air-bubbling and the following same treatment described above, giving an 11:89 mixture (129 mg, 72%) of 5a and 5b.
6-Acetoxymethyl-N2-(N,N-dimethylaminomethylene)pterin (9a)25 and its 7-acetoxymethyl isomer (9b).
The 82:18 mixture of 5a,b (580 mg, 3.00 mmol) was dissolved in dry DMF (15 mL) and then N,N-dimethylformamide dimethyl acetal (1.30 mL, 9.86 mmol) was added. The mixture was stirred at rt for 4 h and concentrated in vacuo. The residue was dissolved in dry pyridine (12 mL) and then acetic anhydride (4.00 mL, 42.3 mmol) was added at 0 °C. The mixture was stirred at rt for 12 h and then concentrated in vacuo. The residue was purified by column chromatography with 1:19 MeOH-CHCl3 to give an inseparable mixture (82:18) of 9a,b (668 mg, 77%) as a pale yellow solid [lit,25 65% yield (9a from 5a)]: Rf = 0.03 (A), 0.40 (B).
9a: 1H-NMR δ 2.15 (1H, s, Ac), 3.18, 3.24 (3H each, 2s, Me2N), 5.35 (2H, s, CH2-6), 8.82 (1H, s, H-7), 8.98 (1H, s, CH=N-2), 9.62 (1H, br s, H-N(3)); 13C-NMR δ 20.79 (COCH3), 35.52, 41.77 (2s, Me2N), 64.82 (CH2–C-6), 130.28 (C-4a), 147.19 (C-6), 149.46 (C-7), 156.09 (C-8a), 157.67 (C-2), 159.82 (NCH=N), 161.80 (C-4), 170.53 (COCH3).
9b: 1H-NMR δ 2.17 (1H, s, Ac), 3.16, 3.22 (3H each, 2s, Me2N), 5.29 (2H, s, CH2-6), 8.56 (1H, s, H-6), 8.96 (1H, s, CH=N-2), 9.99 (1H, br s, H-N(3)); 13C-NMR δ 20.74 (COCH3), 35.40, 41.65 (2s, Me2N), 64.87 (CH2–C-6), 130.72 (C-4a), 139.08 (C-6), 155.77 (C-8a), 156.72 (C-7), 157.93 (C-2), 159.82 (NCH=N), 162.01 (C-4), 170.34 (COCH3).
6-Acetoxymethyl-N2-(N,N-dimethylaminomethylene)-3-[2-(4-nitrophenyl)ethyl]pterin (10a)25 and its 7-acetoxymethyl isomer (10b).
To a solution of 9a,b (668 mg, 2.30 mmol), 2-(p-nitrophenyl)ethanol (588 mg, 3.50 mmol) and triphenylphosphine (1.80 g, 6.86 mmol) in dry 1,4-dioxane (30 mL), was added DEAD (0.710 mL, 3.86 mmol). The mixture was stirred at rt for 12 h and then concentrated in vacuo. The residue was separated by column chromatography with 2:1 AcOEt-hexane to give 10a (763 mg, 46% yield from 8) and 10b (167 mg, 10% yield).
10a: Pale yellow crystals; Rf = 0.09 (A), 0.71 (B); mp 212–213 °C (from AcOEt) (lit.,25 198–200 °C); 1H-NMR δ 2.16 (1H, s, Ac), 3.16 [2H, t, 3J = 7.6 Hz, CH2CH2–N(3)], 3.18, 3.23 (3H each, 2s, Me2N), 4.61 [2H, t, CH2–N(3)], 5.37 (2H, s, CH2-6), 7.40, 8.12 (2H each, 2d, Jo,m = 8.8 Hz, C6H4 of NPE), 8.82 (1H, s, H-7), 8.84 (1H, s, CH=N-2); 13C-NMR δ 20.81 (COCH3), 34.08 (CH2CH2N), 35.48, 41.57 (2s, Me2N), 43.71 (CH2N), 64.90 (CH2–C-6), 123.67 (C(m) of NPE), 129.11 (C-4a), 129.77 (C(o) of NPE), 146.61, 146.71 (2s, C(ipso, p) of NPE), 147.35 (C-6), 149.48 (C-7), 153.98 (C-8a), 157.85 (C-2), 159.26 (NCH=N), 161.84 (C-4), 170.54 (COCH3).
10b: Pale yellow crystals; Rf = 0.14 (A), 0.71 (B); mp 184–185 °C (from AcOEt); 1H-NMR δ 2.20 (1H, s, Ac), 3.17 [2H, t, 3J = 7.6 Hz, CH2CH2–N(3)], 3.18, 3.23 (3H each, 2s, Me2N), 4.62 [2H, t, CH2–N(3)], 5.32 (2H, s, CH2-6), 7.40, 8.13 (2H each, 2d, Jo,m = 8.8 Hz, C6H4 of NPE), 8.60 (1H, s, H-6), 8.85 (1H, s, CH=N-2); 13C-NMR δ 20.77 (COCH3), 34.12 (CH2CH2N), 35.48, 41.57 (2s, Me2N), 43.64 (CH2N), 64.93 (CH2–C-6), 123.68 (C(m) of NPE), 129.63 (C-4a), 129.74 (C(o) of NPE), 139.28 (C-6), 146.63, 146.73 (2s, C(ipso, p) of NPE), 153.65 (C-8a), 156.79 (C-2), 158.12 (C-7), 159.26 (NCH=N), 161.74 (C-4), 170.37 (COCH3). Anal. Calcd for C20H21N7O5: C, 54.67; H, 4.82. Found: C, 54.88; H, 4.99.
N2-(N,N-Dimethylaminomethylene)- 6-hydroxymethyl-3-[2-(4-nitrophenyl)ethyl]pterin (11).
Compound 26a (1.47 g, 3.36 mmol) was dissolved in dry MeOH (20 mL) and dry CH2Cl2 (20 mL) and then sodium methoxide (28% in MeOH, 0.16 mL, 0.84 mmol) was added at 0 °C. The mixture was stirred at rt for 2 h and neutralized with Amberlite IR-120(H+). The resin was filtered off and the filtrate was evaporated in vacuo. The residue was recrystallized from CHCl3 to give 27 as pale yellow solid. An additional amount of 27 was obtained from the mother liquor by column chromatographic purification: total yield 1.30 g (97%); Rf = 0.44 (B); mp 220–223 °C; 1H-NMR δ 3.13 (1H, br s, HO), 3.17 [2H, t, 3J = 7.6 Hz, CH2CH2–N(3)], 3.19, 3.24 (3H each, 2s, Me2N), 4.62 [2H, t, CH2–N(3)], 4.96 (2H, s, CH2-6), 7.41, 8.14 (2H each, 2d, Jo,m = 8.8 Hz, C6H4 of NPE), 8.85 (1H, s, H-7), 8.86 (1H, s, CH=N-2); 13C-NMR δ 34.13 (CH2CH2N), 35.48, 41.57 (2s, Me2N), 43.77 (CH2N), 63.32 (CH2–C-6), 123.72 (C(m) of NPE), 128.60 (C-4a), 129.80 (C(o) of NPE), 146.63, 146.76 (2s, C(ipso, p) of NPE), 151.41 (C-6), 148.76 (C-7), 153.86 (C-8a), 157.56 (C-2), 159.21 (NCH=N), 162.02 (C-4). Anal. Calcd for C18H19N7O4: C, 54.40; H, 4.82. Found: C, 54.19; H, 4.95.
N2-(N,N-Dimethylaminomethylene)-3-[2-(4-nitrophenyl)ethyl]-6-[(2,3,4,6-tetra-O-benzoyl-β-D- glucopyranosyloxy)methyl]pterin (13).
To a solution of 11 (25.6 mg, 0.0644 mmol), 2,3,4,6-tetra-O-benzoyl-α-D-glucopyranosyl bromide (12) (127 mg, 0.194 mmol) and TMU (0.008 mL, 0.064 mmol) in dry CHCl3 (10 mL), was added silver triflate (33.0 mg, 0.13 mmol). The mixture was stirred at rt for 3 h in the dark and then refluxed for 3 h. The mixture was cooled to rt, diluted with CHCl3, and filtered through Celite. The filtrate was washed with aqueous NaHCO3, dried (Na2SO4), and evaporated in vacuo. The residue was separated by column chromatography with 2:1 AcOEt-hexane to give 13 (39.7 mg, 63%) and 11 (4.1 mg, 16% recovery).
13: Pale yellow crystals; mp 139–141 °C (from AcOEt); Rf = 0.24 (A), 0.68 (B); 1H-NMR δ 3.15 [2H, t, 3J = 7.6 Hz, CH2CH2-N(3)], 3.16, 3.21 (3H each, 2s, Me2N), 4.20 (1H, ddd, J4,5 = 9.9, J5,6b = 5.2, J5,6a = 3.1 Hz, H-5*), 4.46 (1H, dd, J6a,6b = 12.2 Hz, Hb-6*), 4.61 (1H, dd, Ha-6*), 4.59 [2H, t, CH2-N(3)], 5.08 (1H, d, J1,2 = 7.9 Hz, H-1*), 5.66 (dd, J2,3 = 9.9 Hz, H-2*), 5.70 (1H, t, J3,4 = 9.6 Hz, H-4*), 5.93 (1H, t, H-3*), 5.01, 5.21 (1H each, 2d, 2JCH2 = 13.7 Hz, CH2-6), 7.34, 8.12 (2H each, 2d, Jo,m = 8.6 Hz, C6H4), 7.26-7.54 [12H, m, Bz(m,p)*], 7.82, 7.89, 7.92, 7.99 [2H each, 4dd, Jo,m = 8.0, Jo,p = 1.7 Hz, Bz(o)*], 8.81 (1H, s, CH=N-2), 8.84 (1H, s, H-7), *for glycosyl moiety. Anal. Calcd for C52H45N7O13: C, 63.99; H, 4.65. Found: C, 64.11; H, 4.71.
6-Benzoyloxy-N2-(N,N-Dimethylaminomethylene)-3-[2-(4-nitrophenyl)ethyl]pterin (15).
To a solution of 11 (30.0 mg, 0.0755 mmol), 12 (230 mg, 0.349 mmol) and TMU (0.018 mL, 0.15 mmol) in dry CHCl3 (12 mL), was added silver triflate (77.6 mg, 0.302 mmol). The mixture was stirred at rt for 3 h in the dark and then refluxed for 3 h. The mixture was worked up by use of the same procedures described above to give 13 (31.4 mg, 43%) and 15 (13.0 mg, 34%).
15: Pale yellow crystals; mp 208–210 °C (from AcOEt); Rf = 0.60 (B); 1H-NMR δ 3.17 [2H, t, 3J = 7.6 Hz, CH2CH2–N(3)], 3.18, 3.23 (3H each, 2s, Me2N), 4.63 [2H, t, CH2–N(3)], 5.64 (2H each, s, CH2-6), 7.41, 8.13 (2H each, 2d, Jo,m = 8.6 Hz, C6H4 of NPE), 7.45 [2H, t, Jo,m = Jm,p = 7.8 Hz, Bz(m)], 7.58 [1H, td, Jo,m = 1.7 Hz, Bz(p)], 8.09 [2H, dd, Bz(o)], 8.85 (1H, s, CH=N-2), 8.94 (1H, s, H-7). Anal. Calcd for C25H23N7O5: C, 59.87; H, 4.62. Found: C, 59.98; H, 4.79.
3,4,6-Tri-O-benzoyl-α-D-glucopyranose-1,2-{[N2-(N,N-dimethylaminomethylene)-3-(4-nitrophenylethyl)pterin-6-yl]methyl}orthobenzoate (14).
To a solution of 11 (20.0 mg, 0.0503 mmol) and 12 (100 mg, 0.152 mmol) in CHCl3(8.0 mL) were added tetraethylammonium bromide (21.0 mg, 0.116 mmol) and N-ethyldiisopropylamine (0.018 mL, 0.10 mmol). The mixture was then refluxed for 42 h. After cooling, the mixture was diluted with CHCl3, washed with water, dried (Na2SO4), and evaporated in vacuo. The residue was purified by column chromatography with AcOEt to give 14 (45.8 mg, 93% yield) as a pale yellow syrup: Rf = 0.26 (A), 0.73 (B); 1H-NMR δ 3.12 [2H, t, 3J = 7.6 Hz, CH2CH2–N(3)], 3.15, 3.20 (3H each, 2s, Me2N), 4.11 (1H, ddd, J4,5 = 8.8, J5,6b = 5.1, J5,6a = 2.9 Hz, H-5*), 4.37 (1H, dd, J6a,6b = 12.1 Hz, Hb-6*), 4.52 (1H, dd, Ha-6*), 4.57 [2H, t, CH2–N(3)], 4.69, 4.72 (1H each, 2d, 2JCH2 = 13.3 Hz, CH2-6), 4.90 (1H, ddd, J1,2 = 5.1, J2,3 = 3.2, 4J2,4 = 1.2 Hz, H-2*), 5.49 (1H, dt, J3,4 = 1.5 Hz, H-4*), 5.74 (1H, dd, H-3*), 6.13 (1H, d, H-1*), 7.26, 7.42, 7.425, 7.43 [2H each, 4t, Jo,m = Jm,p = 8.0 Hz, Ph(m)*], 7.36, 8.10 (2H each, 2d, Jo,m = 8.6 Hz, C6H4 of NPE), 7.44, 7.48, 7.58, 7.59 [1H each, 4tt, Jo,p = 1.7 Hz, Ph(p)*], 7.83, 7.925, 7.93, 8.06 [2H each, 4dd, Ph(o)*], 8.81 (1H, s, CH=N-2), 8.83 (1H, s, H-7), *for glycosyl moiety. Anal. Calcd for C52H45N7O13: C, 63.99; H, 4.65. Found: C, 64.18; H, 4.44.
N2-(N,N-Dimethylaminomethylene)-3-[2-(4-nitrophenyl)ethyl]-6-{[4,6-di-O-acetyl-2,3-di-O-(4- methoxybenzyl)-α-D-glucopyranosyloxy]methyl}pterin (17).
To a solution of 11 (30.0 mg, 0.0755 mmol) and 16 (127 mg, 0.224 mmol) in CHCl3 (6.0 mL) was added tetraethylammonium bromide (28.0 mg, 0.155 mmol) and N-ethyldiisopropylamine (0.027 mL, 0.155 mmol). The mixture was refluxed for 24 h, diluted with CHCl3, washed with water, dried (Na2SO4), and evaporated in vacuo. The residue was purified by column chromatography with AcOEt to give 17 (45.5 mg, 69% yield) as a pale yellow syrup: Rf = 0.07 (A), 0.80 (B); 1H-NMR δ 1.94, 2.06 (3H each, 2s, AcO-4,6*), 3.19, 3.24 (3H each, 2s, Me2N), 3.18 [2H, t, 3J = 7.8 Hz, CH2CH2–N(3)], 3.62 (1H, dd, J2,3 = 9.5, J1,2 = 3.7 Hz, H-2*), 3.78, 3.79 (3H each, 2s, MeO*), 3.79 (1H, ddd, J4,5 = 10.0, J5,6a = 5.6, J5,6b = 2.2 Hz, H-5*), 3.93 (1H, dd, J6a,6b = 12.7 Hz, Hb-6*), 3.94 (1H, t, J3,4 = 9.3 Hz, H-3*), 4.22 (1H, dd, Ha-6*), 4.57, 4.72 (2H each, 2d, 2J = 11.5 Hz, CH2O-2 or 3*), 4.58, 4.80 (2H each, 2d, 2J = 11.2 Hz, CH2O-2 or 3*), 4.63 [2H, t, CH2–N(3)], 4.82, 4.97 (1H each, 2d, 2JCH2 = 13.7 Hz, CH2-6), 4.85 (1H, d, H-1*), 5.00 (1H, dd, H-4*), 6.83, 6.85, 7.20, 7.25 (2H each, 4 d, Jo,m = 8.6 Hz, C6H4 of PMB*), 7.41, 8.13 (2H each, 2d, Jo,m = 8.7 Hz, C6H4 of NPE), 8.86 (1H, s, CH=N-2), 9.03 (1H, s, H-7), *for glycosyl moiety. Anal. Calcd for C44H49N7O13: C, 59.79; H, 5.59. Found: C, 59.55; H, 5.79.
N2-(N,N-Dimethylaminomethylene)-3-[2-(4-nitrophenyl)ethyl]-6-[(2,3,4,6-tetra-O-acetyl-α-D-glucopyranosyloxy)methyl]pterin (18).
To a solution of 17 (47.0 mg, 0.0532 mmol) in CH2Cl2 (4.0 ml) containing water (0.4 mL) was added DDQ (120 mg, 0.529 mmol). The mixture was stirred at rt for 2 h and then diluted with CHCl3. The mixture was washed with aqueous NaHCO3, dried (Na2SO4), and evaporated in vacuo. The residue was dissolved in dry pyridine (2.0 mL) and then acetic anhydride (0.25 mL, 2.66 mmol) was added at 0 °C. The mixture was stirred at rt for 12 h and then evaporated in vacuo. The residue was purified by column chromatography with AcOEt to give 18 (26.8 mg, 69% yield) as a pale yellow syrup: Rf = 0.06 (A), 0.71 (B); 1H-NMR δ 2.02, 2.03, 2.09, 2.12 (3H each, 4s, AcO-2,3,4,6*), 3.17 [2H, t, 3J = 7.7 Hz, CH2CH2–N(3)], 3.19, 3.24 (3H each, 2s, Me2N), 4.13 (1H, ddd, J4,5 = 10.3, J5,6a = 4.6, J5,6b = 2.2 Hz, H-5*), 4.08 (1H, dd, J6a,6b = 12.2 Hz, Hb-6*), 4.29 (1H, dd, Ha-6*), 4.62 [2H, t, CH2–N(3)], 4.86, 5.03 (1H each, 2d, 2JCH2 = 13.4 Hz, CH2-6), 4.96 (1H, dd, J1,2 = 3.9, J2,3 = 10.3 Hz, H-2*), 5.09 (1H, t, J3,4 = 9.5 Hz, H-4*), 5.21 (1H, d, H-1*), 5.54 (1H, t, H-3*), 7.40, 8.13 (2H each, 2d, Jo,m = 8.7 Hz, C6H4 of NPE), 8.84 (1H, s, CH=N-2), 8.91 (1H, s, H-7), *for glycosyl moiety. Anal. Calcd for C32H37N7O13: C, 52.82; H, 5.13. Found: C, 52.69; H, 5.01.
6-[(α-D-Glucopyranosyloxy)methyl]pterin (6a).
A. From 18. Compound 18 (27.2 mg, 0.0374 mmol) was dissolved in MeOH (2.0 mL) and 28% aqueous ammonia solution (2.0 mL) was added. The mixture was stirred at rt for 12 h and then evaporated in vacuo. The residue was dissolved in DMF (2.0 mL) and then DBU (0.039 mL, 0.26 mmol) was added. The mixture was stirred at rt for 12 h, diluted with water (4.0 mL) and neutralized with Amberlite FPC3500(H+). The resin was filtered off and the filtrate was evaporated in vacuo. The residue was washed with CHCl3 and dried under reduced pressure to give 6a (12.0 mg, 90%).
B. From 19a. Compound 19a (18.2 mg, 0.0322 mmol) was dissolved in MeOH (1.0 mL) and 28% aqueous ammonia solution (1.0 mL) was added. The mixture was stirred at rt for 12 h and then evaporated in vacuo. The residue was washed with CHCl3 and dried under reduced pressure to give 6a (10.4 mg, 91% yield) as a pale yellow solid: mp 222–224 °C (dec.); Rf = 0.12 (C); [α]D28 +47.1° (c 0.65, H2O); UV (pH 7) λmax 236 nm (log ε 3.95), 275 (4.01), 346 (3.65); 1H and 13C-NMR, see Table 2. Anal. Calcd for C13H17N5O7: C, 43.95; H, 4.82. Found: C, 44.09; H, 4.90.
N2-Acetyl-6-[(2,3,4,6-tetra-O-acetyl-α-D-glucopyranosyloxy)methyl]pterin (19a).
Compound 6a (12.0 mg, 0.0338 mmol) was dissolved in dry DMF (1.0 mL) and dry pyridine (2.0 mL) and then acetic anhydride (0.10 mL, 1.04 mmol) was added at 0 °C. The mixture was stirred at rt for 12 h and then evaporated in vacuo. The residue was purified by column chromatography with 1:99 MeOH-CHCl3 to give 19a (18.2 mg, 95%) as a pale yellow syrup: Rf = 0.60 (B); 1H-NMR δ 2.02, 2.025, 2.09, 2.11 (3H each, 4s, AcO-2,3,4,6*), 2.49 (3H, s, AcN), 4.11 (1H, ddd, J4,5 = 10.3, J5,6a = 4.4, J5,6b = 2.4 Hz, H-5*), 4.09 (1H, dd, J6a,6b = 12.0 Hz, Hb-6*), 4.28 (1H, dd, Ha-6*), 4.88, 5.07 (1H each, 2d, 2JCH2 = 13.5 Hz, CH2-6), 4.97 (1H, dd, J2,3 = 10.3, J1,2 = 3.9 Hz, H-2*), 5.10 (1H, t, J3,4 = 9.5 Hz, H-4*), 5.25 (1H, d, H-1*), 5.53 (1H, t, H-3*), 9.00 (1H, s, H-7), 11.04 [1H, br s, H-N(3)], 12.75 (1H, br s, AcNH), *for glycosyl moiety. Anal. Calcd for C23H27N5O12: C, 48.85; H, 4.81. Found: C, 48.98; H, 4.99.
6-[(β-D-Glucopyranosyloxy)methyl]pterin (6b).
A. From 13. Compound 13 (32.0 mg, 0.0328 mmol) was dissolved in dry MeOH (1.0 mL) and dry CH2Cl2 (1.0 mL) and then sodium methoxide (28% in MeOH, 0.007 mL, 0.03 mmol) was added at 0 °C. The mixture was stirred at rt for 2 h and neutralized with Amberlite IR-120(H+). The resin was filtered off and the filtrate was evaporated in vacuo. The residue was dissolved in MeOH (2.0 mL) and then 28% aqueous ammonia solution (2.0 mL) was added. The mixture was stirred at rt for 12 h and evaporated in vacuo. The residue was dissolved in DMF (2.0 mL) and then DBU (0.029 mL, 0.20 mmol) was added. The mixture was stirred at rt for 18 h, diluted with water (4.0 mL), and neutralized with Amberlite FPC3500(H+). The resin was filtered off and the filtrate was evaporated in vacuo. The residue was washed with CHCl3 and dried under reduced pressure to give 6b (10.1 mg, 87%).
B. From 19b. By use of same procedures for 6a from 19a, compound 6b (15.1 mg, 0.0267 mmol) was converted into 6b (8.7 mg, 92%) as a pale yellow solid: mp 238–240 °C (dec.); Rf = 0.12 (C); [α]D28 –6.3° (c 0.12, H2O); UV (pH 7) λmax 237 nm (log ε 3.94), 276 (4.04), 347 (3.63); 1H and 13C-NMR, see Table 2. Anal. Calcd for C13H17N5O7: C, 43.95; H, 4.82. Found: C, 44.12; H, 4.70.
N2-Acetyl-6-[(2,3,4,6-tetra-O-acetyl-β-D-glucopyranosyloxy)methyl]pterin (19b).
By use of same procedures for 19a from 6a, compound 6b (10.1 mg, 0.0284 mmol) was converted into 19b (15.1 mg, 94%): pale yellow syrup; Rf = 0.29 (B); 1H-NMR δ 2.01, 2.03, 2.06, 2.07 (3H each, 4s, AcO-2,3,4,6*), 2.45 (3H, s, AcN), 3.73 (1H, ddd, J4,5 = 10.0, J5,6a = 4.9, J5,6b = 2.2 Hz, H-5*), 4.10 (1H, dd, J6a,6b = 12.5 Hz, Hb-6*), 4.19 (1H, dd, Ha-6*), 4.71 (1H, d, J1,2 = 7.8 Hz, H-1*), 5.00, 5.08 (1H each, 2d, 2JCH2 = 14.0 Hz, CH2-6), 5.10 (1H, t, J3,4 = 9.3 Hz, H-4*), 5.12 (1H, dd, J2,3 = 9.8 Hz, H-2*), 5.22 (1H, t, H-3*), 8.90 (1H, s, H-7), 10.68 [1H, br s, H-N(3)], 12.80 (1H, br s, AcNH), *for glycosyl moiety. Anal. Calcd for C23H27N5O12: C, 48.85; H, 4.81. Found: C, 49.04; H, 4.77.
ACKNOWLEDGEMENTS
We are grateful to the SC-NMR Laboratory of Okayama University for the NMR measurements and to WESCO Scientific Promotion Foundation (to T. H.) which partially supported this work.
References
1. H. S. Forrest, C. Van Baalen, and J. Myers, Arch. Biochem. Biophys., 1958, 78, 95. CrossRef
2. T. Matsunaga, J. G. Burgess, N. Yamada, K. Komatsu, S. Yoshida, and Y. Wachi, Appl. Microbiol. Biotechnol., 1993, 39, 250.
3. Y. K. Choi, Y. K. Hwang, Y. H. Kang, and Y. S. Park, Pteridines, 2001, 12, 121.
4. Y. Noguchi, A. Ishii, A. Matsushima, D. Haishi, K. Yasumuro, T. Moriguchi, T. Wada, Y. Kodera, M. Hiroto, H. Nishihara, M. Sekine, and Y. Inada, Mar. Biotechnol., 1999, 1, 207. CrossRef
5. T. Hanaya, K. Torigoe, K. Soranaka, H. Fujita, H. Yamamoto, and W. Pfleiderer, Pteridines, 2008, 19, 72.
6. K. W. Cha, W. Pfleiderer, and J. J. Yim, Helv. Chim. Acta, 1995, 78, 600. CrossRef
7. S.-H. Cho, J.-U. Na, H. Youn, C.-S. Hwang, C.-H. Lee, and S.-O. Kang, Biochim. Biophys. Acta, 1998, 1379, 53.
8. X. Lin and R. H. White, J. Bacteriol., 1988, 170, 1396.
9. D. L. Hatfield, C. van Baalen, and H. S. Forrest, Plant Physiol., 1961, 36, 240. CrossRef
10. Although the hydrolyzed products of natural 6-[(D-glucopyranosyloxy)methyl]pterin (6) were identified to be 6-hydroxymethylpterin (5a) and D-glucose, no physical data including NMR spectra for 6 were recorded in Ref. 9. Therefore the anomeric structure of 6 remains to be established.
11. H. W. Lee, C. H. Oh, A. Geyer, W. Pfleiderer, and Y. S. Park, Biochim. Biophys. Acta, 1999, 1410, 61. CrossRef
12. (a) Some inhibitory activities against tyrosinase were reported for biopterin α-D-glucoside (2): Y. Wachi, S. Yoshida, K. Komatsu, and T. Matsunaga, Jpn. Patent, 05,286,989, 1993 (Chem. Abstr., 1994, 120, 161782t); (b) Photostabilization of photosynthetic pigments was also recorded for 2: T. Saito, H. Ishikawa, Y. Hada, K. Fukui, Y. Kodera, A. Matsushima, and Y. Inada, Dyes Pigments, 2003, 56, 203. CrossRef
13. T. Hanaya, K. Soranaka, K. Harada, H. Yamaguchi, R. Suzuki, Y. Endo, H. Yamamoto, and W. Pfleiderer, Heterocycles, 2006, 67, 299. CrossRef
14. (a) T. Hanaya, H. Toyota, and H. Yamamoto, Synlett, 2006, 2075; CrossRef (b) T. Hanaya, H. Baba, H. Toyota, and H. Yamamoto, Tetrahedron, 2008, 64, 2090; CrossRef (c) T. Hanaya, H. Baba, M. Kanemoto, and H. Yamamoto, Heterocycles, 2008, 76, 635. CrossRef
15. (a) T. Hanaya, H. Baba, and H. Yamamoto, Heterocycles, 2009, 77, 747; CrossRef (b) T. Hanaya, H. Baba, H. Toyota, and H. Yamamoto, Tetrahedron, 2009, 65, 7989. CrossRef
16. C. M. Baugh and E. Shaw, J. Org. Chem., 1964, 29, 3610. CrossRef
17. W. Pfleiderer, ’Physical Methods in Heterocyclic Chemistry’, Vol. 1, ed. by A. R. Katritzky, Academic Press, New York, 1963, pp. 177–188.
18. T. Hanaya, K. Torigoe, K. Soranaka, H. Yamamoto, Q. Yao, and W. Pfleiderer, Pteridines, 1995, 6, 1.
19. (a) J. P. Geerts, A. Nagel, and H. C. Van der Plas, Org. Magn. Reson., 1976, 8, 606; CrossRef (b) T. Hanaya, D. Takayama, and H. Yamamoto, Heterocycles, 2006, 70, 355. CrossRef
20. Compound 11 is more soluble in chloroform than in dichloromethane, but a considerable amount of chloroform was still needed to dissolve 11.
21. R. K. Ness, H. G. Fletcher, Jr., and C. S. Hudson, J. Am. Chem. Soc., 1950, 72, 2200. CrossRef
22. S. Hanessian and J. Banoub, Methods Carbohydr. Chem., 1980, 8, 247.
23. R. U. Lemieux, K. B. Hendriks, R. V. Stick, and K. James, J. Am. Chem. Soc., 1975, 97, 4056. CrossRef
24. J. Banoub and D. R. Bundle, Can. J. Chem., 1979, 57, 2091. CrossRef
25. Q. Yao and W. Pfleiderer, Helv. Chim. Acta, 2003, 86, 1. CrossRef