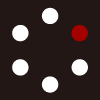
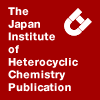
HETEROCYCLES
An International Journal for Reviews and Communications in Heterocyclic ChemistryWeb Edition ISSN: 1881-0942
Published online by The Japan Institute of Heterocyclic Chemistry
e-Journal
Full Text HTML
Received, 2nd July, 2009, Accepted, 31st July, 2009, Published online, 4th August, 2009.
DOI: 10.3987/COM-09-S(S)47
■ Titanium Tetraisopropoxide Promoted Reactions for the Synthesis of Substituted Coumarins
Takanori Tanaka, Kohei Yamashita, and Masahiko Hayashi*
Kobe University, 1-1 Rokkodai-cho, Nada-ku, Kobe 657-8501, Japan
Abstract
Substituted coumarins are synthesized by the reaction of salicylaldehyde derivatives with malonitrile, isopropyl cyanoacetate or diisopropyl malonate promoted by Ti(O-i-Pr)4. When i-PrOH is used as a solvent, these reactions proceeded by the catalytic amount of Ti(O-i-Pr)4 (~0.1 equivalent).The coumarin moiety exists widely such as in perfume compounds, dye compounds, and pharmaceutical agents.1,2 Furthermore, coumarin derivatives have utilities in a wide range of scientific fields. For example, the coumarins are known as fluorescent probe molecules for measurements of solvation dynamics.3 Therefore, several synthetic methods of the functionalized coumarins have been developed. There are fundamentally two methods, one is the so-called Pechmann condensation, that is, acid-mediated condensation of phenol with β-ketoesters to produce coumarins.4 In these reactions, strong acids such as H2SO4 and AlCl3 have been used. The other methods for synthesis of the coumarins is the reaction of salicylaldehydes (o-hydroxybenzaldehydes) with the active methylene compounds via Brønsted base-promoted Knoevenagel5 reaction and following Brønsted acid-promoted intramolecular Pinner reaction.6
During the course of our mechanistic study for the enantioselective reaction between aldehydes and diketene catalyzed by the chiral Schiff base__Ti(O-i-Pr)4 complex,7 we observed the Knoevenagel reaction promoted by Ti(O-i-Pr)4 at room temperature.8 Then, we reported an Knoevenagel reaction of aldehydes or ketones with malononitrile, isopropyl cyanoacetate and diisopropyl malonate catalyzed by Ti(O-i-Pr)4 in i-PrOH as a solvent.9 When i-PrOH was used as a solvent, the Knoevenagel reaction proceeded by the only catalytic amount of Ti(O-i-Pr)4 (~5 mol%). In this paper, we will describe the mild synthesis of substituted coumarins via Ti(O-i-Pr)4-promoted Knoevenagel reaction and following Pinner reaction.
We first examined the reaction of salicylaldehyde (1a) with malononitrile (2a) in the presence of Ti(O-i-Pr)4 in MeCN and i-PrOH (Table 1). In the case of using MeCN as a solvent, 3-cyanocoumarin (3a) was obtained in 78 and 62% yield, respectively, with two and one equivalent of Ti(O-i-Pr)4-promoted Knoevenagel reaction and following Pinner reaction at room temperature. On the other hand, the use of i-PrOH as a solvent improved the reactivity of this transformation. The reaction in the presence of one equivalent of Ti(O-i-Pr)4 proceeded smoothly to produce 3a in 91% yield in i-PrOH. Furthermore, this process proceeded by the catalytic amount of Ti(O-i-Pr)4 (0.1 equivalent) to afford 3a in 70% yield.
Based on the results of Table 1, we found that i-PrOH was available as asolvent. So, we then applied this reaction for the synthesis of substituted coumarin derivatives in i-PrOH (Table 2). The introduction of isopropoxycarbonyl group to the 3-position of coumarin was achieved by using isopropyl cyanoacetate (2b) in the presence of one equivalent of Ti(O-i-Pr)4 in i-PrOH to give 3-isopropoxycarbonylcoumarin (3b). In this reaction, 3-cyanocoumarin (3a) was not formed. The reaction of diisopropyl malonate (2c) was so sluggish that the yield of 3b was only moderate (37%). Substituted salicylaldehydes (1b and 1c) were acceptable as substrate for this reaction. Furthermore, we found only in the case of malononitrile (2a) was employed, the use of catalytic amount of Ti(O-i-Pr)4 gave the products, that is, 6,8-di-tert-butyl-3-cyanocoumarin (3c) and 6-chloro-3-cyanocoumarin (3d) were obtained by this system in 52% and 58% yield, respectively (entries 3 and 4).
So far, we employed the reactions using malononitrile (2a), isopropyl cyanoacetate (2b) and diisopropyl malonate (2c) those generate their enolate species easily under the reaction conditions. On the other hand, by the examination of the NMR study, we have revealed that titanium enolate species were generated by the reaction between Ti(O-i-Pr)4 and diketene.8 Therefore, we thought this titanium enolate species can also react with salicylaldehydes to afford 3-acetylcoumarins (Scheme 1). Actually, the Ti(O-i-Pr)4-promoted Knoevenagel reaction followed by Pinner reaction in i-PrOH gave 3-acetylcoumarin (3e) and 6-chloro-3-acetylcoumarin (3f) in 59% and 53% yield, respectively.
The present method has the following characteristic features: (1) Ti(O-i-Pr)4-mediated synthesis of substituted coumarins was achieved in non-toxic i-PrOH. (2) In the case of malononitrile, only
a catalytic amount (0.1 equiv) of Ti(O-i-Pr)4 was required for smooth reaction. (3) As for reaction temperature, room temperature was enough to obtain substituted coumarins.
EXPERIMENTAL
General: All reactions were carried out in oven-dried glassware with magnetic stirring. All melting points were measured on a Yanaco MP-500D and uncorrected. 1H and 13C NMR spectra (400 and 100.6 MHz, respectively) were recorded on a JEOL JNM-LA 400 using Me4Si as the internal standard (0 ppm). The following abbreviations are used: s = singlet, d = doublet, t = triplet, q = quartet, m = multiplet. IR spectra were measured with a PERKIN ELMER FT-IR Spectrometer SPECTRUM 1000. Elemental analyses were performed with a Yanaco CHN Corder MT-5. Mass spectra were measured on a Thermo Quest LCQ DECA plus. Preparative column chromatography was carried out on Fuji Silysia BW-820MH or YMC*GEL Silica (6 nm I-40-63 um). Thin-layer chromatography (TLC) was carried out on Merck 25 TLC aluminum sheets Silica gel 60 F254.
Typical procedure for synthesis of coumarins via Ti(O-i-Pr)4 promoted Knoevenagel reaction and Pinner reaction: Malononitrile (330 mg, 5 mmol) and i-PrOH (Wako dehydrated grade) 6 mL were placed in a Schlenk tube under argon atmosphere. To this solution, salicylaldehydes (0.52 mL, 5 mmol) then Ti(O-i-Pr)4 (0.15 mL, 0.5 mmol) were added and stirred at room temperature (25 ºC) for 11 h. After confirmation of the completion of the reaction, the reaction mixture was poured into 1M HCl aq. and vigorously stirred for 1 h. It was extracted by EtOAc and the extract was washed with aqueous sodium bicarbonate and brine solution. The organic layer was dried with anhydrous sodium sulfate and evaporated. Purification of the residues by recrystallization using EtOH afforded 3-cyanocoumarin (599.0 mg, 70%).
3-cyanocoumarin (3a). Rf = 0.30 (2:1 hexane–EtOAc); mp 184–186 ºC (lit.,10 180–182 ºC); IR (KBr, νmax (cm-1)): 2229 (CN), 1728 (C=O), 1604 (C=C); 1H NMR: δ (CDCl3) 8.28 (s, 1H), 7.73 (t, 1H, J = 8.0 Hz), 7.62 (dd, 1H, J = 8.0, 1.6 Hz), 7.43 (t, 1H, J = 8.0 Hz), 7.42 (t, 1H, J = 8.0 Hz); 13C NMR: δ (CDCl3) 155.5, 154.0, 136.5, 130.8, 126.5, 118.5, 118.3, 117.9, 115.2, 103.8; MS m/z (relative intensity): 171 (M+, 100%), 143 (99%), 115 (50%), 88 (27%), 63 (24%), 62 (23%).
3-isopropoxycarboxycoumarin (3b). Rf = 0.62 (3:1 hexane–EtOAc); mp 89–90 ºC (lit.,11 84–86 ºC); IR (KBr, νmax (cm-1)): 1750 (C=O), 1606 (C=C); 1H NMR: δ (CDCl3) 8.47 (s, 1H), 7.64 (t, 1H, J = 8.0 Hz), 7.61 (dd, 1H, J = 8.0, 1.6 Hz), 7.36 (d, 1H, J = 8.0 Hz), 7.34 (t, 1H, J = 8.0 Hz), 5.32–5.23 (m, 1H), 1.4 (d, 6H, J = 6.0 Hz); 13C NMR: δ (CDCl3) 162.4, 156.7, 155.1, 148.0, 134.2, 129.4, 124.8, 118.7, 117.9, 116.8, 69.7, 21.8. MS m/z (relative intensity): 232 (M+, 26%), 174 (35%), 173 (98%), 146 (100%), 118 (41%), 101 (22%), 89 (41%), 43 (49%).
6,8-di-tert-butyl-3-cyanocoumarin (3c). Rf = 0.70 (2:1 hexane–EtOAc); mp 146–149 ºC (lit.,10 143–144 ºC); IR (KBr, νmax (cm-1)): 2234 (CN), 1751 (C=O), 1615 (C=C); 1H NMR: δ (CDCl3) 8.24 (s, 1H), 7.75 (d, 1H, J = 2.4 Hz), 7.37 (d, 1H, J = 2.4 Hz), 1.50 (s, 9H), 1.36 (s, 1H); 13C NMR: δ (CDCl3) 156.4, 153.1, 151.6, 148.3, 138.3, 131.2, 123.7, 117.2, 113.9, 101.9, 35.3, 34.8, 31.2, 29.8; MS m/z (relative intensity): 283 (M+, 10%), 268 (100%), 240 (22%), 212 (14%), 127 (12%), 57 (55%).
6-chloro-3-cyanocoumarin (3d). Rf = 0.54 (2:1 hexane–EtOAc); mp 191–192 ºC (lit.,10 191–192 ºC); IR (KBr, νmax (cm-1)): 2243 (CN), 1735 (C=O), 1614 (C=C); 1H NMR: δ (CDCl3) 8.19 (s, 1H), 7.67 (dd, 1H, J = 8.8, 2.4 Hz), 7.59 (d, 1H, J = 2.4 Hz), 7.37 (d, 1H, J = 8.8 Hz); 13C NMR: δ (CDCl3) 155.7, 152.9, 150.5, 135.4, 131.2, 128.3, 118.9, 118.0, 113.1, 104.7; MS m/z (relative intensity): 205 (M+, 91%), 177 (100%), 149 (13%), 114 (90%), 88 (26%).
3-acetylcoumarin (3e). Rf = 0.47 (2:1 hexane–EtOAc); mp 119–120 ºC (lit.,12 119–121 ºC); IR (KBr, νmax (cm-1)): 1740 (C=O), 1676 (C=O), 1614 (C=C); 1H NMR: δ (CDCl3) 8.51 (s, 1H), 7.70–7.62 (m, 2H), 7.41–7.32 (m, 2H), 2.73 (s, 1H); 13C NMR: δ (CDCl3) 195.5, 159.2, 155.4, 147.5, 134.4, 130.2, 125.0, 124.6, 118.3, 116.7, 30.6; MS m/z (relative intensity): 188 (M+, 48%), 173 (100%), 145 (14%), 89 (27%).
3-acetyl-6-chlorocoumarin (3f). Rf = 0.58 (2:1 hexane–EtOAc); mp 209–210 ºC (lit.,13 219 ºC); IR (KBr, νmax (cm-1)): 1737 (C=O), 1676 (C=O), 1610 (C=C); 1 H NMR: δ (CDCl3) 8.41 (s, 1H), 7.63 (d, 1H, J = 2.4 Hz), 7.60 (dd, 1H, J = 8.8, 2.4 Hz), 7.33 (d, 1H, J = 8.8 Hz), 2.73 (s, 3H); 13C NMR: δ (CDCl3) 225.1, 195.0, 153.6, 146.1, 134.2, 130.3, 129.1, 125.5, 119.2, 118.2, 30.5; MS m/z (relative intensity): 222 (M+, 42%), 207 (100%), 179 (14%), 152 (22%), 123 (25%).
ACKNOWLEDGEMENTS
We thank Dr. Hideki Amii for helpful discussions. This work was supported by a Grant-in-Aid for Scientific Research on Priority Areas "Advanced Molecular Transformations of Carbon Resources" and No. B17340020 from the Ministry of Education, Culture, Sports, Science and Technology, Japan. T. T. is grateful to Grant-in-Aid for JSPS Fellows.
References
1. R. O'Kennedy and R. D. Thornes, “Coumarins: Biology, Applications and Mode of Action”, Wiley, West Sussex, 1997.
2. S. R. Trenor, A. R. Shultz, B. J. Love, and T. E. Long, Chem. Rev., 2004, 104, 3059. CrossRef
3. M. Maroncelli, J. Mol. Liq., 1993, 57, 1. CrossRef
4. H. Pechmann and C. Duisberg, Ber. Dtsch. Chem. Ges., 1883, 16, 2119. CrossRef
5. E. Knoevenagel, Ber. Dtsch. Chem. Ges., 1894, 27, 2345. CrossRef
6. A. Pinner and F. Klein, Ber. Dtsch. Chem. Ges., 1877, 10, 1889. CrossRef
7. M. Hayashi, T. Inoue, and N. Oguni, J. Chem. Soc., Chem. Commun., 1994, 341; CrossRef M. Hayashi, T. Inoue, Y. Miyamoto, and N. Oguni, Tetrahedron, 1994, 50, 4385. CrossRef
8. M. Hayashi, N. Nakamura, and K. Yamashita, Tetrahedron, 2004, 60, 6777. CrossRef
9. K. Yamashita, T. Tanaka, and M. Hayashi, Tetrahedron, 2005, 61, 7981. CrossRef
10. F. Fringuelli, O. Piermatti, and F. Pizzo, Synthesis, 2003, 2331. CrossRef
11. P. K. Kadaba, Synthesis, 1972, 628. CrossRef
12. H. Valizadeh, A. Shockravi, and H. Gholipur, J. Heterocycl. Chem., 2007, 44, 867. CrossRef
13. B. S. Jayashree, D. Anuradha, and K. N. Venugopala, Asian J. Chem., 2005, 17, 2093.