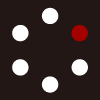
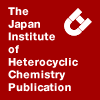
HETEROCYCLES
An International Journal for Reviews and Communications in Heterocyclic ChemistryWeb Edition ISSN: 1881-0942
Published online by The Japan Institute of Heterocyclic Chemistry
e-Journal
Full Text HTML
Received, 27th August, 2009, Accepted, 1st October, 2009, Published online, 2nd October, 2009.
DOI: 10.3987/COM-09-S(S)118
■ Synthesis and Photophysical Properties of 2,3,5,6,7,8-Hexasilabicyclo[2.2.2]octan-1-yl-substituted Arenes
Masaki Shimizu,* Tomoaki Kawaguchi, Hisashi Nakagawa, Katsunari Oda, and Tamejiro Hiyama*
Department of Material Chemistry, Graduate School of Engineering, Kyoto University, Kyoudai-katsura, Nishikyo, Kyoto 615-8510, Japan
Abstract
Incorporation of 2,2,3,3,5,5,6,6,7,7,8,8-dodecamethyl-2,3,5,6,7,8-hexasilabicyclo[2.2.2]octan-1-yl moiety into such chromophores as biphenyl, terphenyl, stilbene, and tolan was found to induce bathochromic shifts of absorption and fluorescence spectra, compared with those of the parent chromophores. Moreover, enhancement of the molar extinction coefficients and the fluorescence quantum yields by the substitution was also observed. These results indicate that σ–π conjugation between the polysilacage moiety and the chromophores is operative. Theoretical study of the polysilacage-substituted chromophores is also presented.INTRODUCTION
Since incorporation of a bicyclo[2.2.2]octane (BCO) moiety into an organic molecule generally impart molecular rigidity and thermal stability into the resulting molecule, the bicyclic group is often utilized as a mesogenic core of calamitic liquid crystalline compounds1 and a rigid core of molecular rods.2 For example, 1-alkyl-4-(4-cyanophenyl)bicyclo[2.2.2]octanes were disclosed to exhibit low birefringence, positive dielectric anisotropy, and higher and wider range of nematic mesophases than the analogs having a benzene or cyclohexane mesogen in place of the cage-like core.3 Silicon is a tetra-coordinate element like carbon, while carbon–silicon and silicon–silicon σ-bonds are much longer than carbon–carbon σ-bonds4 and can act as the module of σ-conjugation due to the relatively high energy levels of HOMO and LUMO of the σ-bonds.5 Hence, substitution of methylene groups in cycloalkanes by dialkylsilylene moieties makes the molecular size bigger than the parent one, and is expected to induce unique molecular rigidity, structure, and electronic property as well as intermolecular interaction in the condensed phases, different from those of the parent cycloalkanes. Based on this idea, we have designed 2,2,3,3,5,5,6,6,7,7,8,8-dodecamethyl-2,3,5,6,7,8-hexasilabicyclo[2.2.2]octane (HSBCO) as a novel polysilabicycloalkane (Figure 1)6,7 and disclosed that 1-alkyl-4-aryl- and 1-alkyl-substituted HSBCO exhibited columnar mesophases.8 Thus the HSBCO derivatives markedly contrasts the corresponding BCO-based liquid crystalline compounds which exhibit the nematic and/or smectic phases. Another unique characteristic of HSBCO is that the polysilacage moiety can conjugate with π-conjugated system introduced at the bridgehead. When the 2,3,5,6,7,8-hexasilabicyclo[2.2.2]octan-1-yl moiety was introduced into such arenes as biphenyl, terphenyl, and tolan, bathochromic shifts of absorption maxima and fluorescence maxima as well as the enhancement of molecular extinction coefficients were observed, compared with the parent arenes.9 Thus, the HSBCO moiety was found to act as a novel and unique cage framework that exhibited not only steric but also electronic effects. We report herein the preparation, photophysical properties, and theoretical study of HSBCO-substituted biphenyl 1, terphenyl 2, stilbene 3, and tolan 4 in detail (Figure 2).
RESULTS AND DISCUSSION
Preparation of HSBCO-substituted arenes
HSBCO-substituted arenes 1-4 were prepared from 1-(4-iodophenyl)-substituted HSBCO8a by use of palladium-catalyzed coupling reactions as shown in Scheme 1. Treatment of 1-phenyl-HSBCO with iodine in the presence of CF3CO2Ag in CHCl3 at room temperature gave the iodophenyl derivative in 88% yield.10 HSBOC-substituted biphenyl 1 and -terphenyl 2 were synthesized by the Suzuki-Miyaura coupling reaction of the iodide with the corresponding arylboronic acids in excellent yields.11 The Heck reaction of styrene and the iodide gave trans-stilbene 3 as a single stereoisomer in 51% yield. Tolan 4 was obtained in excellent yield by the Sonogashira coupling of phenylacetylene and the iodide in the presence of Pd(PPh3)2Cl2 and copper iodide as the catalysts. The HSBCO moiety tolerated all the basic reaction conditions. Products 1-4 were purified by column chromatography on silica gel and stable in air.
UV absorption spectra of HSBCO-substituted arenes
UV absorption spectra of 1–4 are shown in Figures 3 and 4, and the photophysical properties are summarized in Table 1, together with those of biphenyl, terphenyl, stilbene, and tolan for comparison, respectively. UV spectra were measured in cyclohexane upon irradiation with a UV light (λ = 265 nm).
As can be seen in Figures 3 and 4, substitution of HSBCO moiety into arenes increased the molecular extinction coefficients considerably and induced the red-shift of absorption spectra in comparison with the parent arenes, while the vibrational structures of 1–4 were almost similar with those of the parent arenes. Noteworthy is that absorption spectra of 1 was almost identical to that of terphenyl. These results indicates that σ–π (σ∗–π∗) electronic interaction between the HSBCO moiety and the π-conjugated arenes is operative which leads to the decrease of the HOMO–LUMO gaps.12
Fluorescence spectra of HSBCO-substituted arenes
Fluorescence spectra of 1–4 are shown in Figures 5 and 6, together with those of biphenyl, terphenyl, and stilbene for comparison (the spectrum of tolan is not shown due to the extremely low intensity and
quantum yield). The substitution by HSBCO led to the bathochromic shift of the emission spectra and the maxima by 10 to 20 nm, but did not affect the vibronic fine structures of the spectra. Moreover, the quantum yields (Φf) of 1–4 were higher than those of the parent arenes, as summarized in Table 1. For example, Φf of 1 and 4 were 4.6 and 43 times larger than those of biphenyl and tolan, respectively. Thus, HSBCO was found to dramatically enhance Φf of arenes.
To gain insight into the effect of HSBCO on the photophysical properties of arenes, we examined fluorescence decay of 1 and 2. The results are illustrated in Figure 7. Biphenyl 1 showed the decay time of τ = 1.50 ns, 11-fold shorter than that of biphenyl (τ = 16 ns), while the decay time of 2 (τ = 0.79 ns) was slightly shorter than that of terphenyl (τ = 0.95 ns). The radiative rate (kf) of 1 was 55 x 107 s–1, which was 50 times faster than that of biphenyl (kf = 1.1 x 107 s–1), whereas the nonradiative rate (kn) of 1 (12 x 107 s–1) was only 4-fold as compared with that of biphenyl (5.1 x 107 s–1). On the other
hand, the HSBCO substitution into terphenyl (kf = 83 x 107 s–1; kn = 23 x 107 s–1) induced slight enhancement of the radiative rate (kf = 123 x 107 s–1) and considerable decline of the nonradiative rate (kn = 3.1 x 107 s–1). Therefore, the introduction of the HSBCO moiety into arenes apparently results in enhancement of radiative rates, whereas the substitution effect on nonradiative rate of arenes is found to vary depending on the kinds of the parent arenes.
Photophysical properties of silylmethyl-substituted tolans
To disclose the features of the HSBCO group as a σ-conjugation module, UV absorption and fluorescence spectra of related silylmethyl-substituted tolans 5–7 (Figure 8) were measured as shown in Figures 9 and 10, together with those of 4 and the results are summarized in Table 1. HSBCO-substituted tolan 4 and model compounds 5–7 showed absorption spectra with vibrational fine structures similar to that of tolan. As compared with tolan, the absorption spectra red-shifted in the order of 7 < 6 < 5 < 4. Thus, a tris(trimethylsilyl)methyl group that contains a silylmethyl moiety triply conjugates with the π-conjugated system of tolan more effectively than a single silylmethyl group (5 vs
7). Meanwhile, the comparison of 6 and 7 reveals that a pentamethyldisilanyl group at the benzylic position is more beneficial to the extension of the conjugation length than a trimethylsilyl group. Consequently, the electronic effect of the HSBCO group seems to originate from the structural characteristic that the cage framework consists of triple disilanyl groups. The fluorescence quantum yields increased in the same order (Table 1). Considering that the orthogonal arrangement of carbon–silicon σ-bond and π-conjugated plane is favorable for attaining effective σ–π (σ∗–π∗) conjugation, the trend may be closely related to the probability of conformers in which a carbon–silicon σ-bond orients perpendicular to the benzene ring of arenes (conformation A) or one carbon–silicon σ-bond locating in plane and two carbon–silicon σ-bonds above and below the π-plane (conformation B) (Figure 11).
Molecular orbital calculation
Molecular orbital calculation of model compounds 1′–4′ in which SiMe2 group in 1–4 were replaced by SiH2, respectively (Figure 12), was performed by the density functional theory (DFT) method at the HF/6-31G(d)//B3LYP/6-31G(d) level using the Gaussian 98 package.13 The results on HOMO and LUMO energy levels are summarized in Figure 13, and calculated HOMO and LUMO orbitals are visualized in Figure 14. It is obvious that HOMO level of 1′ is raised by 0.20 eV as compared with biphenyl, while LUMO level is lowered by 0.50 eV. Thus, the energy gap between HOMO and LUMO became narrow, resulting in red-shift of absorption spectrum/maxima of 1 as compared with biphenyl. HOMO of 1′ is not only localized on the biphenyl moiety, but also is extended to two Si–C(bridgehead) σ-bonds, suggesting that the rise of HOMO energy originates from σ-π conjugation (Figure 14). On the other hand, LUMO of 1′ delocalizes over the biphenyl moiety and the C–C σ-bond connecting HSBCO and biphenyl. This reflects mixing of the arene π* orbital and two Si-C(bridgehead) σ*
orbitals. Thus, the lower-lying LUMO energy level of 1′ is attributed to σ*-π* conjugation. The HSBCO effect on the HOMO and LUMO behaviors was observed with 2′-4′. Therefore, HSBCO moiety is disclosed to participate in electronic communication with an aryl group at the bridgehead via both σ-π and σ*-π* conjugation.
CONCLUSION
We have synthesized 2,3,5,6,7,8-hexasilabicyclic[2.2.2]octan-1-yl (HSBCO)-substituted biphenyl, terphenyl, stilbene, and tolan from a HSBCO-substituted iodobenzene using Pd-catalyzed coupling reactions. UV absorption and fluorescence spectra of HSBCO-substituted arenes exhibited a bathochromic shift compared with the parent arenes. The incorporation of HSBCO moiety also resulted in the enhancement of molecular extinction coefficients and fluorescence quantum yields. Thus, the electronic communication between the HSBCO moiety and π-conjugated systems is demonstrated. Theoretical calculations suggest that the HSBCO group contributes to the rise of HOMO levels via σ-π conjugation as well as lowering LUMO levels via σ*-π* conjugation. These results demonstrate that the HSBCO moiety can serve as a useful σ-conjugation module to improve optical and/or electronic properties of the parent π-conjugated systems.
EXPERIMENTAL
Melting points were determined using a Seiko Instrument Inc. DSC6200. 1H NMR spectra were measured on a JEOL EX270 (270 MHz), JMN ECP-500 (500 MHz), a Varian Mercury 200 (200 MHz), 300 (300 MHz), and 400 (400 MHz) spectrometers. The chemical shifts of 1H NMR are expressed in parts per million downfield relative to the internal tetramethylsilane (δ = 0 ppm) or chloroform–d (δ = 7.26 ppm), benzene–d6 (δ = 7.16 ppm). Splitting patterns are indicated as s, singlet; d, doublet; t, triplet; q, quartet; m, multiplet; brs, broad singlet. 13C NMR spectra were measured on a JEOL EX270 (68 MHz), JMN ECP-500 (125 MHz), a Varian Mercury 200 (50 MHz), 300 (75 MHz), and 400 (100 MHz) spectrometers with tetramethylsilane as an internal standard (δ = 0 ppm) or chloroform–d (δ = 77.0 ppm), benzene–d6 (δ = 128.4 ppm). 29Si NMR spectra were measured on a JEOL EX270 (54 MHz), JMN ECP-500 (99 MHz), and a Varian Mercury 400 (80 MHz) spectrometer with tetramethylsilane as an internal standard (δ = 0 ppm). Chemical shift values are given in parts per million downfield relative to the internal standard. UV absorption and fluorescence spectra were measured at room temperature in a 1-cm quartz cell with Shimadzu UV–2100PC and Shimadzu RF–5300PC spectrometers, respectively. Spectral grade cyclohexane was degassed by Ar bubbling for 20 min. Infrared spectra (IR) were recorded on a Shimadzu FTIR-8400 spectrometer. GC–MS analyses were performed with a JEOL JMS–700 spectrometer by electron ionization at 70 eV. FAB–MS analyses were performed with a JEOL JMS–HX110A spectrometer with 3-nitrobenzyl alcohol or thioglycerol matrix. TLC analyses were performed by means of Merck Kieselgel 60 F254, and column chromatography was carried out using Wakogel C–200 or Merck Kieselgel 60 (230–400 mesh). Preparative recycling gel permeation chromatography (GPC) was performed with a JAI LC–908 chromatograph equipped with JAIGEL–1H and –2H columns (chloroform as an eluent). Preparative recycling high performance liquid chromatography (HPLC) was performed with a JAI LC–908 chromatograph equipped with 5SL–II columns (hexane and ethyl acetate as an eluent). THF, Et2O, CHCl3, and TMEDA were distilled before use under an argon atmosphere. All the manipulation of oxygen- and moisture-sensitive materials was carried out under an argon atmosphere.
4-(2,2,3,3,5,5,6,6,7,7,8,8-Dodecamethyl-2,3,5,6,7,8-hexasilabicyclo[2.2.2]octan-1-yl)iodobenzene
To a suspension of (2,2,3,3,5,5,6,6,7,7,8,8-dodecamethyl-2,3,5,6,7,8-hexasilabicyclo[2.2.2]octan -1-yl)benzene8a (0.18 g, 0.40 mmol) and silver trifluoroacetate (0.11 g, 0.57 mmol) in CHCl3 (10 mL) was added dropwise a CHCl3 (8 mL) solution of iodine (0.12 g, 0.47 mmol) at room temperature. The mixture was stirred for 2 h, when the reaction was completed as evidenced by TLC. Insoluble materials were filtered off. The residue was extracted with sat. aq. Na2S2O3 (15 mL x 3) and dried over anhydrous magnesium sulfate before concentration in vacuo. The residue was purified by column chromatography on silica gel (eluent, hexane) to give 1-(4-iodophenyl)-substituted HSBCO (0.20 g, 88 % yield) as colorless solid. Mp 269–270 °C. Rf 0.40 (hexane). 1H NMR (270 MHz, CDCl3) δ 0.24 (s, 18H), 0.26 (s, 18H), 7.03 (d, J = 8.8 Hz, 2H), 7.52 (d, J = 8.8 Hz, 2H); 13C NMR (67.8 MHz, CDCl3) δ 2.3, 3.0, 3.4, 21.2, 88.1, 132.6, 136.7, 143.0; 29Si NMR (54 MHz, CDCl3) δ –21.1, –20.4; IR (KBr): 2982, 2943, 2895, 2841, 1479, 1387, 1256, 1151, 1076, 1001, 968, 878, 849, 812, 712, 679, 644, 594, 505, 428 cm-1; MS (FAB) m/z 577 (M++1), 576 (M+). HRMS (FAB) Calcd for C20H41ISi6: M+, 576.0869. Found: 576.0842. Acceptable data of elemental analysis were not obtained presumably due to the incomplete combustion caused by the existence of multiple silicon atoms. This is also true of the other compounds.
4-(2,2,3,3,5,5,6,6,7,7,8,8-Dodecamethyl-2,3,5,6,7,8-hexasilabicyclo[2.2.2]octan-1-yl)biphenyl (1): To a mixture of Pd(OAc)2 (1.5 mg, 6.5 µmol), PPh3 (5.1 mg, 20 µmol), PhB(OH)2 (15 mg, 0.12 mmol), and 1-(4-iodophenyl)-substituted HSBCO (58 mg, 0.10 mmol) were added benzene (2 mL), 0.25 M aq. Na2CO3 (0.6 mL), and water (0.4 mL). The mixture was stirred at 80 °C for 4 h before quenching with sat. aq. NaCl (25 mL). Thereafter, Et2O (50 mL) was added and the separated aq. phase was extracted with Et2O (50 mL x 2). The combined organic layer was washed with sat. aq. NaCl (80 mL) and dried over anhydrous magnesium sulfate, and concentrated in vacuo to afford a crude product, which was purified by column chromatography on silica gel, giving rise to 1 (59 mg, 93% yield) as colorless solid. Mp 292 °C. Rf 0.24 (hexane). 1H NMR (200 MHz, CDCl3) δ –0.02 (s, 1H), 0.27 (s, 18H), 0.29 (s, 18H), 7.20–7.70 (m, 9H); 13C NMR (68 MHz, CDCl3) δ 0.6, 2.5, 3.6, 6.6, 126.1, 126.5, 126.6, 128.6, 130.8, 131.1, 140.6, 142.1; 29Si NMR (54 MHz, CDCl3) δ –21.2, –20.4; IR (KBr): 3030, 2984, 2943, 2893, 2852, 1603, 1485, 1253, 1157, 1072, 968, 881, 856, 812, 735, 714, 692, 679, 613, 592 cm-1; MS (FAB) m/z 526 (M+), 527 (M++1). HRMS (FAB) Calcd for C26H46Si6: M+, 526.2215. Found: 526.2213.
4-(2,2,3,3,5,5,6,6,7,7,8,8-Dodecamethyl-2,3,5,6,7,8-hexasilabicyclo[2.2.2]octan-1-yl)terphenyl (2): To a mixture of Pd(OAc)2 (2.0 mg, 9.1 µmol), PPh3 (7.0 mg, 0.027 mmol), 4-biphenylboronic acid (35 mg, 0.174 mmol), and 1-(4-iodophenyl)-substituted HSBCO (80 mg, 0.139 mmol) were added benzene (3 mL), 0.25 M aq. Na2CO3 (0.9 mL), and water (0.6 mL). The mixture was stirred at 80 °C for 4 h before quenching with sat. aq. NaCl (25 mL) and diluted with Et2O (50 mL). The separated aqueous phase was extracted with diethyl ether (50 mL x 2). The combined organic layer was washed with sat. aq. NaCl (80 mL) and dried over anhydrous magnesium sulfate, and concentrated in vacuo to afford a crude product, which was purified by column chromatography on silica gel, giving rise to 2 (72 mg, 87% yield) as colorless solid. Mp 299 °C. Rf 0.12 (hexane). 1H NMR (200 MHz, CDCl3) δ –0.02 (s, 1H), 0.27 (s, 18H), 0.30 (s, 18H), 7.30–7.80 (m, 13H); 13C NMR (68 MHz, CDCl3) δ 1.1, 2.5, 3.6, 6.6, 126.0, 126.8, 126.9, 127.3, 128.7, 130.8, 131.1, 134.9, 139.3, 139.5, 140.6, 142.3; 29Si NMR (54 MHz, CDCl3) δ –21.1, –20.3; IR (KBr): 3030, 2982, 2945, 2895, 2851, 1599, 1483, 1440, 1255, 1157, 1065, 968, 881, 814, 764, 746, 725, 712, 696, 679, 594 cm-1; MS (FAB) m/z 602 (M+), 603 (M++1). HRMS (FAB) Calcd for C32H50Si6: M+, 602.2528. Found: 602.2509.
(E)-1-{4-(2,2,3,3,5,5,6,6,7,7,8,8-Dodecamethyl-2,3,5,6,7,8-hexasilabicyclo[2.2.2]octan-1-yl)phenyl}-2-phenylethene (3): All the manipulation was carried out in the dark (when the reaction was carried out without shading, the product was obtained as an E/Z mixture). To a N,N-dimethylformamide (2.4 mL) mixture of 1-(4-iodophenyl)-substituted HSBCO (0.15 g, 0.27 mmol), palladium acetate (16 mg, 0.071 mmol), tri-o-tolylphosphine (44 mg, 0.15 mmol) and triethylamine (0.29 mL) was added styrene (90 µL, 82 mg, 0.79 mmol). The reaction mixture was stirred at 100 °C before quenching with water (10 mL). To the mixture was added CH2Cl2 (40 mL), and the separated aqueous phase was extracted with CH2Cl2 (15 mL x 3). The combined organic layer was dried over anhydrous magnesium sulfate and concentrated in vacuo to afford a crude product, which was purified by column chromatography on silica gel (eluent, hexane) followed by preparative GPC to give 3 (93 mg, 63% yield) as colorless solid. Mp 272 °C. Rf 0.30 (hexane). 1H NMR (270 MHz, CDCl3) δ –0.02 (s, 1H), 0.27 (s, 18H), 0.28 (s, 18H), 7.07 (s, 2H), 7.20–7.44 (m, 7H), 7.46–7.54 (m, 2H); 13C NMR (68 MHz, CDCl3) δ 2.5, 3.2, 3.6, 21.8, 125.8, 126.2, 127.1, 127.1, 128.5, 128.5, 130.7, 132.2, 137.6, 142.9; 29Si NMR (54 MHz, CDCl3) δ –21.1, –20.3; IR (KBr): 3024, 2982, 2943, 2895, 2841, 1630, 1595, 1502, 1448, 1406, 1383, 1256, 1151, 964, 814, 752, 712, 691, 679, 664, 652, 594, 552, 542 cm-1; MS (FAB) m/z 552 (M+). HRMS (FAB) Calcd for C28H48Si6: M+, 552.2372. Found: 552.2368.
1-(2,2,3,3,5,5,6,6,7,7,8,8-Dodecamethyl-2,3,5,6,7,8-hexasilabicyclo[2.2.2]octan-1-yl)-4-(phenylethynyl)benzene (4): To a mixture of 1-(4-iodophenyl)-substituted HSBCO (60 mg, 0.11 mmol), Pd(PPh3)2Cl2 (8.8 mg, 0.013 mmol), and diethylamine (12 mL) was added successively copper iodide (4.1 mg, 0.022 mmol) and ethynylbenzene (15 µL, 0.14 mmol) under an argon atmosphere at room temperature. The resulting mixture was stirred at room temperature for 2.5 h. The progress of the reaction was monitored by thin layer chromatography. The reaction mixture was concentrated by evaporation of diethylamine under reduced pressure and the residue was dissolved in water. The aqueous solution was extracted with benzene. The combined organic layer was dried over anhydrous magnesium sulfate and concentrated in vacuo. The residue was purified by column chromatography on silica gel to give 4 (53 mg, 93% yield) as colorless solid. Mp 296.5–297.0 °C. Rf 0.30 (hexane). 1H NMR (500 MHz, CDCl3) δ –0.02 (s, 1H), 0.261 (s, 18H), 0.266 (s, 18H), 7.26–7.36 (m, 5H), 7.38–7.42 (m, 2H), 7.50–7.53 (m, 2H); 13C NMR (68 MHz, CDCl3) δ 2.3, 3.0, 3.4, 22.2, 89.1, 89.6, 117.8, 123.7, 127.9, 128.3, 130.5, 131.0, 131.5, 144.1; 29Si NMR (99 MHz, CDCl3) δ –20.5, –19.8; IR (KBr): 2941, 2895, 1595, 1506, 1256, 1161, 1136, 964, 858, 814, 752, 712, 679, 652, 594, 550 cm-1; MS (FAB) m/z 551 (M++1), 550 (M+). HRMS (FAB) Calcd for C28H46Si6: M+, 550.2215. Found 550.2219.
Silylmethyl-substituted tolans 5-7 were prepared in a manner similar to the procedure for 4.
1-Tris(trimethylsilyl)methyl-4-(phenylethynyl)benzene (5): 97% yield. Colorless solid, mp 82.0–83.0 °C. Rf 0.50 (hexane). 1H NMR (500 MHz, CDCl3) δ 0.25 (s, 27H), 7.30–7.45 (m, 7H), 7.52–7.56 (m, 2H); 13C NMR (125 MHz, CDCl3) δ 4.1, 22.3, 89.1, 89.5, 118.0, 123.6, 128.0, 128.3, 130.7, 131.41, 131.48, 144.4; 29Si NMR (99 MHz, CDCl3) δ 1.8; IR (KBr): 2955, 2901, 1595, 1506, 1443, 1408, 1256, 1155, 1130, 1070, 1018, 866, 760, 677, 646, 619, 542, 519, 430 cm-1; MS (FAB) m/z 409 (M++1), 408 (M+). HRMS (FAB) Calcd for C24H36Si3: M+, 408.2125. Found 408.2125.
1-(Pentamethyldisilyl)methyl-4-(phenylethynyl)benzene (6): 95% yield. Colorless solid, mp 64.4–65.0 °C. Rf 0.30 (hexane). s 1H NMR (500 MHz, CDCl3) δ 0.036 (s, 6H), 0.041 (s, 9 H), 2.19 (s, 2H), 6.99 (d, J = 8.3 Hz, 2H), 7.30–7.37 (m, 3H), 7.41 (d, J = 8.2 Hz, 2H), 7.51–7.55 (m, 2H); 13C NMR (125 MHz, CDCl3) δ –4.3, –2.2, 25.1, 88.5, 89.8, 118.5, 123.6, 127.9, 128.0, 128.3, 131.5, 141.5; 29Si NMR (99 MHz, CDCl3) δ –16.1, 18.9; IR (KBr): 2949, 2891, 2212, 1593, 1508, 1495, 1443, 1402, 1246, 1205, 1142, 835, 802, 787, 752, 731, 691, 610, 544, 525, 503 cm-1; MS (FAB) m/z 323 (M++1), 322 (M+). HRMS (FAB) Calcd for C20H26Si2: M+, 322.1573. Found 322.1578.
1-(Trimethylsilyl)methyl-4-(phenylethynyl)benzene (7): 70% yield. Colorless oil. Rf 0.30 (hexane). 1H NMR (500 MHz, CDCl3) δ 0.01 (s, 9H), 2.12 (s, 2H), 6.99 (d, J = 7.9 Hz, 2H), 7.30–7.45 (m, 5H), 7.50–7.60 (m, 2H); 13C NMR (125 MHz, CDCl3) δ –1.8, 27.6, 88.5, 89.8, 118.5, 123.5, 127.8, 127.9, 128.2, 131.35, 131.38, 141.3; 29Si NMR (99 MHz, CDCl3) δ –1.6; IR (neat): 2953, 2893, 2216, 1595, 1510, 1491, 1443, 1412, 1250, 1209, 1151, 1078, 851, 754, 691, 596, 544, 523, 502, 415 cm-1; MS (FAB) m/z 265 (M++1), 264 (M+). HRMS (FAB) Calcd for C18H20Si: M+, 264.1334. Found 264.1342.
ACKNOWLEDGEMENTS
This work was supported by Grants-in-Aid for Creative Scientific Research, No 16GS0209, COE Research on Elements Science and 21st century COE program for a united approach for new materials science from Ministry of Education, Culture, Sports, Science and Technology, Japan. The authors acknowledge Professor S. Ito and Professor H. Ohkita at Kyoto University for the measurement of fluorescence decay.
References
1. Handbook of Liquid Crystals, Vol. 1-4, ed. by D. Demus, J. Goodby, G. W. Gray, H.-W. Spiess, and V. Vill, Wiley-VCH, Weinheim, 1998.
2. P. F. H. Schwab, M. D. Levin, and J. Michl, Chem. Rev., 1999, 99, 1863. CrossRef
3. (a) G. W. Gray and S. M. Kelly, Angew. Chem., Int. Ed. Engl., 1981, 20, 393; CrossRef (b) G. W. Gray and S. M. Kelly, J. Chem. Soc., Perkin Trans. 2, 1981, 26; CrossRef (c) N. Carr, G. W. Gray, and S. M. Kelly, Mol. Cryst. Liq. Cryst., 1985, 129, 301. CrossRef
4. J. Emsley, The Elements Third Edition, Oxford University Press, Oxford, 2000.
5. (a) M. Kumada and K. Tamao, Adv. Organomet. Chem., 1968, 6, 19; CrossRef (b) E. F. Hengge, J. Organomet. Chem. Lib., 1979, 9, 261; (c) H. Sakurai, J. Organomet. Chem., 1980, 200, 261; CrossRef (d) R. West, Organopolysilanes, Vol. 2, Pergamon Press, Oxford, 1982; (e) R. West, Pure & Appl. Chem., 1982, 54, 1041; CrossRef (f) H. Watanabe and Y. Nagai, Chemistry of Small Ring Polysilanes, Ellis Horwood, Chichester, 1985; (g) R. West, J. Organomet. Chem., 1986, 300, 327; CrossRef (h) R. D. Miller and J. Michl, Chem. Rev., 1989, 89, 1359; CrossRef (i) T. Tsumuraya, S. A. Batcheller, and S. Masamune, Angew. Chem. Int. Ed. Engl., 1991, 30, 902; CrossRef (j) R. West, Organopolysilanes, Vol. 2, Pergamon, Oxford, 1995; (k) A. Sekiguchi and H. Sakurai, Adv. Organomet. Chem., 1995, 37, 1; CrossRef (l) H. Isaka, H. Teramae, M. Fujiki, and N. Matsumoto, Macromolecules, 1995, 28, 4733; CrossRef (m) T. Sanji, H. Hanao, and H. Sakurai, Chem. Lett., 1997, 1121; CrossRef (n) S. Yamaguchi and K. Tamao, J. Chem. Soc., Dalton Trans., 1998, 3693; CrossRef (o) H. Tsuji, J. Michl, and K. Tamao, J. Organomet. Chem., 2003, 685, 9; CrossRef (p) S. Yamaguchi and K. Tamao, Chem. Lett., 2005, 34, 2. CrossRef
6. (a) M. Shimizu, N. Inamasu, Y. Nishihara, and T. Hiyama, Chem. Lett., 1998, 1145; CrossRef (b) M. Shimizu, S. Ishizaki, H. Nakagawa, and T. Hiyama, Synlett, 1999, 1772; CrossRef (c) M. Shimizu, T. Hiyama, T. Matsubara, and T. Yamabe, J. Organomet. Chem., 2000, 611, 12; CrossRef (d) M. Shimizu, S. Sugimoto, M. Mizukoshi, and T. Hiyama, J. Organomet. Chem., 2003, 685, 138; CrossRef (e) M. Shimizu, H. Mizukoshi, and T. Hiyama, Synthesis, 2004, 1363. CrossRef
7. Other examples of silicon-containing bicyclo[2.2.2]octanes: (a) R. West and A. Indriksons, J. Am. Chem. Soc., 1972, 94, 6110; CrossRef (b) M. Ishikawa, M. Watanabe, J. Iyoda, H. Ikeda, and M. Kumada, Organometallics, 1982, 1, 317; CrossRef (c) A. Ochida, K. Hara, H. Ito, and M. Sawamura, Org. Lett., 2003, 5, 2671; CrossRef (d) W. Setaka, N. Hamada, and M. Kira, Chem. Lett., 2004, 33, 626; CrossRef (e) W. Setaka, N. Hamada, C. Kabuto, and M. Kira, Chem. Commun., 2005, 4666; CrossRef (f) A. Ochida, S. Ito, T. Miyahara, H. Ito, and M. Sawamura, Chem. Lett., 2006, 35, 294; CrossRef (g) G. Hamasaka, A. Ochida, K. Hara, and M. Sawamura, Angew. Chem. Int. Ed., 2007, 46, 5381; CrossRef (h) A. Ochida, G. Hamasaka, Y. Yamauchi, S. Kawamorita, N. Oshima, K. Hara, H. Ohmiya, and M. Sawamura, Organometallics, 2008, 27, 5494; CrossRef (i) S. Kawamorita, H. Ohmiya, K. Hara, A. Fukuoka, and M. Sawamura, J. Am. Chem. Soc., 2009, 131, 5058. CrossRef
8. (a) M. Shimizu, K. Watanabe, H. Nakagawa, T. Becker, S. Sugimoto, and T. Hiyama, Chem. Lett., 2001, 1090; CrossRef (b) M. Shimizu, M. Nata, K. Watanabe, and T. Hiyama, Trans. Mater. Res. Soc. Jpn, 2004, 29, 793; (c) M. Shimizu, M. Nata, K. Watanabe, T. Hiyama, and S. Ujiie, Mol. Cryst. Liq. Cryst., 2005, 441, 237; CrossRef (d) M. Shimizu, M. Nata, K. Mochida, T. Hiyama, S. Ujiie, M. Yoshio, and T. Kato, Angew. Chem. Int. Ed., 2007, 46, 3055. CrossRef
9. M. Shimizu, T. Kawaguchi, K. Oda, and T. Hiyama, Chem. Lett., 2007, 36, 412. CrossRef
10. R. A. Glennon, R. Young, F. Benington, and R. D. Morin, J. Med. Chem., 1982, 25, 1163. CrossRef
11. (a) N. Miyaura and A. Suzuki, Chem. Rev., 1995, 95, 2457; CrossRef (b) A. Suzuki, in Metal-catalyzed Cross-coupling Reactions, ed. by F. Diederich and P. J. Stang, Wiley-VCH, Weinheim, 1998, p. 49; (c) A. Suzuki, J. Organomet. Chem., 1999, 576, 147; CrossRef (d) N. Miyaura, in Top. Curr. Chem., Vol. 219, ed. by N. Miyaura, Springer-Verlag, Berlin, 2002, p. 11; (e) N. Miyaura, in Metal-Catalyzed Cross-Coupling Reactions, Vol. 1, ed. by A. de Meijere and F. Diederich, Wiley-VCH, Weinheim, 2004, p. 41. CrossRef
12. Photochemical and photophysical studies on benzylsilanes: (a) H. Sakurai, M. Ochiai, and M. Kira, Chem. Lett., 1972, 87; CrossRef (b) J. B. Lambert and R. A. Singer, J. Am. Chem. Soc., 1992, 114, 10246; CrossRef (c) H. Hiratsuka, S. Kobayashi, T. Minegishi, M. Hara, T. Okutsu, and S. Murakami, J. Phys. Chem. A, 1999, 103, 9174; CrossRef (d) H. Maeda, K. I. Nishimura, K. Mizuno, M. Yamaji, J. Oshima, and S. Tobita, J. Org. Chem., 2005, 70, 9693; CrossRef (e) M. Shimizu, H. Tatsumi, K. Mochida, and T. Hiyama, Chem. Commun., 2008, 2134. CrossRef
13. Gaussian 98 (Revision A.7), M. J. Frisch, G. W. Trucks, H. B. Schlegel, G. E. Scuseria, M. A. Robb, J. R. Cheeseman, V. G. Zakrzewski, J. A. Montgomery, R. E. Stratmann, J. C. Burant, S. Dapprich, J. M. Millam, A. D. Daniels, K. N. Kudin, M. C. Strain, O. Farkas, J. Tomasi, V. Barone, M. Cossi, R. Cammi, B. Mennucci, C. Pomelli, C. Adamo, S. Clifford, J. Ochterski, G. A. Petersson, P. Y. Ayala, Q. Cui, K. Morokuma, D. K. Malick, A. D. Rabuck, K. Raghavachari, J. B. Foresman, J. Cioslowski, J. V. Ortiz, B. B. Stefanov, G. Liu, A. Liashenko, P. Piskorz, I. Komaromi, R. Gomperts, R. L. Martin, D. J. Fox, T. Keith, M. A. Al-Laham, C. Y. Peng, A. Nanayakkara, C. Gonzalez, M. Challacombe, P. M. W. Gill, B. G. Johnson, W. Chen, M. W. Wong, J. L. Andres, M. Head-Gordon, E. S. Replogle, J. A. Pople, Gaussian, Inc., Pittsburgh, PA, 1998.