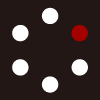
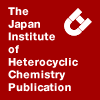
HETEROCYCLES
An International Journal for Reviews and Communications in Heterocyclic ChemistryWeb Edition ISSN: 1881-0942
Published online by The Japan Institute of Heterocyclic Chemistry
e-Journal
Full Text HTML
Received, 20th May, 2009, Accepted, 15th June, 2009, Published online, 16th June, 2009.
DOI: 10.3987/COM-09-S(S)29
■ A New Entry for Preparation of 2-Substituted Azaindoles
Montaser Shaykoon Ahmed Shaykoon, Fuyuhiko Inagaki, and Chisato Mukai*
Division of Pharmaceutical Sciecnes, Graduate School of Natural Science and Technology, Kanazawa University, Kakuma, Kanazawa, Ishikawa 920-1192, Japan
Abstract
A series of 2-substituted 5-, 6- and 7-azaindoles were synthesized from iodo-N-(tert-butoxycarbonyl)aminopyridines via the corresponding allenyl derivatives.Recently, we reported a novel method for the one-step synthesis of 2-methyl-3-substituted indoles 3 (R3=H) under the typical Stille conditions1 in the presence of tetrabutylammonium chloride (TBAC) that effected the coupling reaction between N-acyl-2-iodoanilines 1 and the 1-(tributylstannyl)-1-substituted allenes 2, followed by the formal endo-mode cyclization of the resulting allenyl species.2 An alternative one-pot procedure including a successive Stille reaction (with or without TBAC) and a subsequent TBAF treatment resulted in the efficient formation of the other types of 2-alkyl-3-substituted indoles (Scheme 1). This method could successfully be applied to the synthesis of indomethacin.
The azaindole frameworks,3 a bioisostere of indole, are involved as a core framework in various natural products and pharmaceuticals.4 We describe here a new synthetic protocol for the preparation of 2-substituted azaindole derivatives based on the our own newly developed method for the construction of the indole skeleton.
At the beginning of this program, synthesis of 2-substituted 7-azaindole was examined (Table 1).
According to the previously established conditions, which directly led N-acylanilines to the corresponding indole derivatives, 2-aminopyridine derivative 4 was treated with 5a5 in DMF in the presence of 3 mol % Pd2(dba)3, tri-2-furylphosphine (TFP, 24 mol %), CuI (10 mol %), and TBAC (3 equiv) at room temperature (condition A) for 60 h to afford unexpectedly a complex mixture (entry 1). When the reaction was carried out in the absence of TBAC (condition B), the Stille coupling product 6a was obtained in 86% yield (entry 2). Changing the solvent from DMF to THF decreased the chemical yield of 6a (entry 3). Treatment of 6a with TBAF in THF gave 7-azaindole 7a in 58% yield as expected (Scheme 2).
Compound 4 was exposed to other allenes 5b-f in the presence of a palladium catalyst providing the corresponding Stille coupling products 6b-f in satisfactory yields as summarized in Table 2. As can be seen in Table 2, the condition without TBAC (condition B) consistently produced the allenyl derivatives 6b-f (entries 1-5), whereas the condition with TBAC (condition A) was again found not to be effective for our purpose (entries 6-10). This was not the case in the reaction of 1 with 2 where the condition with TBAC generally afforded indole frameworks directly and the Stille coupling products were obtained
under the condition without TBAC. Cyclization of allenylaminopyridine derivative 6b with TBAF gave 2-methyl-7-azaindole (7b) in 69% yield (Table 3, entry 1). In the reaction of 6c, 2-vinyl-7-azaindole (7c’) (67%) was obtained instead of 7c (entry 2).6 2-Hexyl- and 2-isobutyl-7-azaindoles 7d,f were synthesized from 6d,f in 74% and 68% yield, respectively (entries 3,5). In the case of 6e, the corresponding cyclized product 7e was obtained in a rather low yield along with the recovery of the starting material (50% yield)(entry 4). Thus, it was shown that the palladium-catalyzed coupling reaction of 2-aminopyridines with 3-substituted allenylstannanes, followed by base treatment results in the production of the corresponding 2-substituted-7-azaindoles.
Our efforts were then directed toward synthesis of 6-azaindoles. Treatment of 3-amino-4-iodopyridine derivative 8 with 1,2-butadienyltributylstannane (5a) in the presence of Pd2(dba)3, TFP, and CuI in DMF at room temperature (condition B), however, afforded neither allenylpyridine 9a nor 6-azaindole 10a (Table 4, entry 1). In contrast to the conversion of 4 into 7, the condition with TBAC (condition A) worked well in this case providing directly 2-ethyl-6-azaindole (10a) in 82% yield (entry 2). Other 6-azaindoles 10b-f possessing Me, (CH2)2OTBS, and hexyl substituents at the C2-position were easily prepared in high yields under the condition A (entries 3-5). In the case of tributyl(5-phenyl- 1,2-pentadienyl)stannane (5e), 6-azaindole derivative 10e was isolated in a moderate yield (entry 6). The condition with TBAC (condition A) was not useful for the preparation of 10f. In fact, a mixture of 10f and the allenyl derivative 9f was obtained in 31% yield when exposed to condition A (entry 7).
An efficient synthesis of 2-isobutyl-6-azaindole (10f) (66%) was accomplished by using condition B, followed by base treatment as shown in Scheme 3.
2-Substitued-5-azaindoles were our final target compounds in this investigation. By taking into account the aforementioned two conditions A and B (condition with or without TBAC), we attempted the conversion of 3-iodo-4-aminopyridine derivative 11 into the targeted compounds. Treatment of 11 with allenylstannane 5a under condition A gave no desired products at all. Instead, the formation of 3-allenyl-4-aminopyridine derivative 12a was observed in 80% yield under condition B (Table 5, entry 1). Condition A was found to be effective for the direct synthesis of 2-methyl-5-azaindole derivative 13b in a satisfactory yield (entry 2). 2-Silyloxyethyl congener 13c was also directly formed under condition A, but
the chemical yield was rather low (entries 3). Allenylstannanes 5d-f having n-pentyl, phenethyl, and i-propyl groups on the R position reacted with 11 under condition B to give the corresponding 3-allenyl-4-aminopyridine derivatives 12d-f in good yields (entry 4-6). Cyclization of 12a,d-f with TBAF treatment proceeded without any difficulty to provide the 2-substituted-5-azaindoles 13a,d-f in satisfactory yields. The results are summarized in Table 6.
In summary, we have developed a new procedure for the synthesis of 2-substituted-7-azaindoles on the basis of successive Stille coupling of 2-amino-3-iodopyridines with the 1-(tributylstannyl)-3-substituted allenes, followed by cyclization of the resulting allenyl derivatives with TBAF treatment. 2-Substituted-6-azaindoles could be directly synthesized in a one-pot process under the palladium-catalyzed condition with TBAC. Synthesis of 2-substituted-5-azaindoles could be achieved by a proper choice of reaction conditions (with or without TBAC/base treatment). These results (formation of allene and/or azaindole) would reflect the electronic property of the amino functionality of the starting pyridine derivatives. Thus, we could add an alternative method for the preparation of 2-substituted-5-, 6-, and 7-azaindoles.
ACKNOWLEDGEMENTS
This work was supported in part by a Grant-in-Aid for Scientific Research from the Ministry of Education, Culture, Sports, Science and Technology, Japan, for which we are grateful. M. S. A. S. thanks the Ministry of Higher Education and Scientific Research, Egypt, for a fellowship.
References
1. J. K. Stille, Angew. Chem., Int. Ed. Engl., 1986, 25, 508; CrossRef V. Farina, V. Krishnamurphy, and W. J. Scott, Org. React., 1997, 50, 1.
2. N. Kuroda, Y. Takahashi, K. Yoshinaga, and C. Mukai, Org. Lett., 2006, 8, 1843; CrossRef C. Mukai and Y. Takahashi, Org. Lett., 2005, 7, 5793. CrossRef
3. For some references, see: F. Popowycz, J.-Y. Mérour, and B. Joseph, Tetrahedron, 2007, 63, 8689; CrossRef F. Popowycz, S. Routier, B. Joseph, and J.-Y. Mérour, Tetrahedron, 2007, 63, 1031; CrossRef Z. Zhang, Z. Yang, N. A. Meanwell, J. F. Kadow, and T. Wang, J. Org. Chem., 2002, 67, 2345; CrossRef D. Hands, B. Bishop, M. Cameron, J. S. Edwards, I. F. Cottrell, and S. H. B. Wright, Synthesis, 1996, 877; CrossRef I. Mahadevan and M. Rasmussen, J. Heterocycl. Chem., 1992, 29, 359; CrossRef M. H. Fisher and A. R. Matzuk, J. Heterocycl. Chem., 1969, 6, 775; CrossRef R. H. Dodd, X. Doisy, and P. Potier, Heterocycles, 1989, 28, 1101. CrossRef
4. A. Trejo, H. Arzeno, M. Browner, S. Chanda, S. Cheng, D. D. Comer, S. A. Dalrymple, P. Dunten, J. Lafargue, B. Lovejoy, J. Freire-Moar, J. Lim, J. Mcintosh, J. Miller, E. Papp, D. Reuter, R. Roberts, F. Sanpablo, J. Saunders, K. Song, A. Villasenor, S. D. Warren, M. Welch, P. Weller, P. E. Whiteley, L. Zeng, and D. M. Goldstein, J. Med. Chem., 2003, 46, 4702; CrossRef C. N. Hodge, P. E. Aldrich, Z. R. Wasserman, C. H. Fernandez, G. A. Nemeth, A. Arvanitis, R. S. Cheeseman, R. J. Chorvat, E. Ciganek, T. E. Christos, P. J. Gilligan, P. Krenitsky, E. Scholfield, and P. Strucely, J. Med. Chem., 1999, 42, 819; CrossRef C. Marot, P. Chavatte, L. Morin-Allory, M. C. Viaud, G. Guillaumet, P. Renard, D. Lesieur, and A. Michel, J. Med. Chem., 1998, 41, 4453; CrossRef J. R. Henry, K. C. Rupert, J. H. Dodd, I. J. Turchi, S. A. Wadsworth, D. E. Cavender, B. Fahmy, G. C. Olini, J. E. Davis, J. L. Pellegrino-Gensey, P. H. Schafer, and J. J. Siekierka, J. Med. Chem., 1998, 41, 4196; CrossRef J. R. Henry and J. H. Dodd, Tetrahednm Lett., 1998, 39, 8763; CrossRef J. J. Kulagowski, H. B. Broughton, N. R. Curtis, I. M. Mawer, M. P. Ridgill, R. Baker, F. Emms, S. B. Freedman, R. Marwood, S. Patel, S. Patel, C. I. Ragan, and P. D. Leeson, J. Med. Chem., 1996, 39, 1941. CrossRef
5. All allenes used in this study were prepared by the method of Marshall, see: D. R. Williams, L. Mi, R. J. Mullins, and R. E. Stites, Tetrahedron Lett., 2002, 43, 4841; CrossRef J. A. Marshall and C. M. Grant, J. Org. Chem., 1999, 64, 8214; CrossRef J. A. Marshall and X.-J. Wang, J. Org. Chem., 1992, 57, 1242. CrossRef
6. Compound 7c (23%) was isolated after heating a solution of 6c in DMF at 80 °C for 3 h.