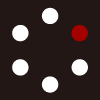
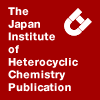
HETEROCYCLES
An International Journal for Reviews and Communications in Heterocyclic ChemistryWeb Edition ISSN: 1881-0942
Published online by The Japan Institute of Heterocyclic Chemistry
e-Journal
Full Text HTML
Received, 28th March, 2009, Accepted, 14th May, 2009, Published online, 15th May, 2009.
DOI: 10.3987/COM-09-S(S)20
■ A New Diterpenoid Alkaloid, Sharwuphinine A from Delphinium sharwurense
Chen Li, Yusuke Hirasawa, Hiroko Arai, Haji Akber Aisa,* and Hiroshi Morita*
Faculty of Pharmaceutical Sciences, Hoshi University, 2-4-41 Ebara, Shinagawa-ku, Tokyo 142-8501, Japan
Abstract
A new diterpenoid alkaloid, sharwuphinine A (1) was isolated from the aerial part of Delphinium sharwurense and the structure possessing a lycoctonine-type C19-diterpenoid alkaloid with a nitrone functionality was elucidated by spectroscopic analysis. Sharwuphinine A (1) showed moderate vasorelaxant activity on rat aorta.Delphinium species have been employed in analgesic balms, sedatives, emetics, and antihelminthics in folk medicine.1 About twenty species are distributed in Xinjiang Uygur autonomous region, People’s Republic of China among approximately 300 species in the world. Diterpenoid alkaloids are the major active constituents of Delphinium plants belonging to Ranunculaceae. We have so far isolated several diterpenoid alkaloids such as elatine from Delphinium sharwurense W. T. Wang,2 which showed muscle relaxing activity.3 On continuing search for chemical constituents of D. sharwurense with biological activity, we have isolated a new diterpenoid alkaloid with a nitrone functionality, sharwuphinine A (1), which showed moderate vasorelaxant activity against rat aorta. This paper describes the isolation and structure elucidation of 1 and vasodilator effect on rat aorta.
The aerial parts of D. sharwurense were extracted with 80% EtOH, and the extract was in turn partitioned between CHCl3 and 5% H2SO4 aq. Water-soluble materials, which were adjusted to pH 10 with saturated Na2CO3 aq., were extracted with CHCl3 and the residual materials were extracted with BuOH. BuOH-soluble materials were subjected to a silica gel column (CHCl3/MeOH 1:0→1:1, CHCl3/MeOH/H2O 5:5:1, and MeOH) and the fractions eluted by CHCl3/MeOH (50:1→5:5) were subjected to an amino silica gel column (CHCl3/MeOH 1:0→1:1) to afford sharwuphinine A (1, 0.0004%) together with lycoctonine (2, 0.002%)4 and deoxymethylenelycoctonine (3, 0.005%).5
Sharwuphinine A 1 {[α]D23 +25 (c 0.2, MeOH)} showed the pseudomolecular ion peak at m/z 454 (M+H)+ in ESIMS, and the molecular formula, C23H35NO8, was established by HRESIMS [m/z 454.2446, (M+H)+ Δ +1.1 mDa]. IR spectrum suggested the presence of hydroxy (3433 cm-1) and an imino (1650 cm-1) groups. The 13C NMR spectrum of 1 (Table 1) disclosed twenty-three carbon signals due to four sp3 quaternary carbons (δC 45.7, 53.0, 78.8, and 86.9), one sp2 methine (δC 144.1), nine sp3 methines (δC 39.4, 43.7, 44.4, 46.1, 79.0, 82.1, 84.1, 85.6, and 91.0), five sp3 methylenes (δC 21.0, 26.3, 32.0, 34.3, and 65.0), and four methoxy groups (δC 56.6, 57.0, 58.1, and 59.3). Since 1 out of 7 elements of unsaturation implied by the molecular formula was accounted for, 1 was inferred to possess 6 rings.
Four partial structures a (C-1 ~ C-3), b (C-5 ~ C-6), c (C-9 ~ C-14), and d (C-15 ~ C-16) were assigned by detailed analyses of 2D NMR data (1H-1H COSY and HMQC) of 1. Connectivity of units a and b through C-4 and C-11 was implied by HMBC correlations for H2-3 and H-5 to C-4 (δC 45.7), and H-1 and H-5 to C-11 (δC 53.0). The presence of an imino group at C-19 was verified by the IR absorption (1650 cm-1) and a methine carbon resonance (δ 144.1, C-19). The UV absorption (λmax 250 nm) corroborated the presence of a nitrone group.6 The HMBC cross-peak of H-19 to C-17 (δC 79.0) and H2-18 to C-4 placed the nitrone between C-17 and C-19 and a hydroxymethylene at C-4, respectively. HMBC correlations were observed for H-5 to C-10 (δC 43.7), H-6 to C-7 (δC 86.9) and C-8 (δC 78.8), and H2-15 to C-7, C-8, and C-9 (δC 44.4), suggesting that a cyclopentane ring (unit c) was connected through C-7, C-8, and C-11 to unit b in a decahydroazulene ring system and also a cyclohexane ring was formed with unit d through C-8. In addition, connectivities between four methoxy groups and C-1, C-6, C-14, and C-16 were implied by HMBC correlations for each methoxy group to C-1, C-6, C-14, and C-16, respectively. Αll of this evidence indicated that sharwuphinine A (1) is a lycoctonine-type C19-diterpenoid alkaloid and 1 was a nitrone of lycoctonine without N-ethyl group.
The relative stereochemistry of 1 was elucidated by NOESY correlations. It was no doubt that one relative stereostructure existed for fused-tetracyclic ring system (C-1 ~ C-11, C-17, C-19 and N) with no strain, which was satisfied with NOESY correlations of H-5/H-9 and H-19/H-3a and H-6. The NOESY correlation of H-2a/H-5 indicated that the cyclohexane ring (C-1 ~ C-5 and C-11) took boat conformation. In addition, H-1 was correlated with both H-10 and H-12a, and H-6 with H-19 indicating that H-1 was β-oriented and H-6 was α-oriented. Furthermore, the relative configurations at C-14 and C-16 were deduced from NOESY correlations of H-14/H-10 and H-12a, and those among H-12b, H-16, and H-17 as shown in computer-generated 3D drawing (Figure 2).
Compound 1 was named sharwuphinine A, and the first natural diterpene alkaloid with a nitrone functionality. Biogenetically sharwuphinine A might be derived from lycoctonine by formation of N-oxide followed by N-deethylation and then production of a hydroxylamine derivative.
The vasodilators are useful for treatment of cerebral vasospasm and hypertension, and for improvement of peripheral circulation.7 Many natural products evoked vasodilatation through multiple mechanisms such as the nitric oxide system, potassium channel, and calcium channel functions. Among diterepenoid alkaloids, mesaconitine, one of the aconite alkaloids, has been inhibited against phenylephrine-induced contraction in the endothelium-intact, but not endothelium-denuded, aortic rings.8 When phenylephrine (PE) 3 × 10-7 M was applied to thoracic aortic rings with endothelium after achieving a maximal response, we added sharwuphinine A (1), lycoctonine (2), and deoxymethylenelycoctonine (3). Among them, sharwuphinine A (1) showed a moderate vasorelaxant activity (40% relaxation at 10-4 M), whereas lycoctonine (2) and deoxymethylenelycoctonine (3) did not show vasorelaxant actions. Vasodilation may seem to be influenced by the hydrophobicity of a nitrogen atom. Efforts are currently underway to determine the mode of action of sharwuphinine A (1) on vasorelaxant activity.
EXPERIMENTAL
General Experimental Procedures. 1D and 2D NMR spectra were recorded on a JEOL ECA600 spectrometer and chemical shifts were reported using residual CD3OD (δH 3.31 and δC 49.0) as internal standard. HMQC experiments were optimized for 1JCH=145 Hz and HMBC experiments for nJCH=8Hz. Mass spectra were recorded on a Micromass LCT spectrometer.
Plant Material. The aerial parts of D. sharwurense W. T. Wang were collected in Xinjiang autonomous region of the Chinese People’s Republic during flowering in July 2005. The aerial parts of this plant were identified by Prof. Shi Ming Duan of Xinjiang Institute of Ecology and Geography. A voucher specimen was deposited at the Xinjiang Technical Institute of Physics and Chemistry.
Extraction and Isolation. The aerial parts of D. sharwurense (2.9 kg) were dried, grounded, and extracted with 80% EtOH at rt and the EtOH extract was partitioned between CHCl3 and 5% H2SO4 aq. Water-soluble materials, which were adjusted to pH 10 with saturated Na2CO3 aq., were extracted with CHCl3. Water-soluble materials were extracted with BuOH. BuOH-soluble materials were subjected to a silica column (CHCl3/MeOH 1:0→1:1, CHCl3/MeOH/H2O 5:5:1, and MeOH) to afford thirteen fractions. The fractions eluted by CHCl3/MeOH (50:1→5:5) were subjected to an amino silica gel column (CHCl3/MeOH 1:0→1:1) to afford sharwuphinine A (1, 11.6 mg, 0.0004%), lycoctonine (2, 58 mg, 0.002%),4 and deoxymethylenelycoctonine (3, 145 mg, 0.005%).5
Sharwuphinine A (1): brown solid; [α]D23 +25 (c 0.2, MeOH ); IR (film) νmax 3433, 2943, 2882, 2824, 1650, 1605, 1461, 1385, 1223, and 1088 cm-1; UV (MeOH) λmax 250 (ε 6500) nm; 1H and 13C NMR data (Table 1); ESIMS m/z 454 (M+H)+; HRESITOFMS m/z 454.2446[(M+H)+, calcd for C23H36NO8, 454.2435].
Vasodilation Assay.7 A male Wistar rat weighting 260 g was sacrificed by bleeding from carotid arteries under an anesthetization. A section of the thoracic aorta between the aortic arch and the diaphragm was removed and placed in oxygenated, modified Krebs-Henseleit solution (KHS: 118.0 mM NaCl, 4.7 mM KCl, 25.0 mM NaHCO3, 1.8 mM CaCl2, 1.2 mM NaH2PO4, 1.2 mM MgSO4, and 11.0 mM glucose). The aorta was cleaned of loosely adhering fat and connective tissue and cut into ring preparations 3 mm in length. The tissue was placed in a well-oxygenated (95% O2, 5% CO2) bath of 5 mL KHS solution at 37 °C with one end connected to a tissue holder and the other to a force-displacement transducer (Nihon Kohden, TB-611T). The tissue was equilibrated for 60 min under a resting tension of 1.0 g. During this time the KHS in the tissue bath was replaced every 20 min.
After equilibration, each aortic ring was contracted by treatment with 3 x 10-7 M PE. The presence of functional endothelial cells was confirmed by demonstrating relaxation to 10-5 M acetylcholine (ACh), and aortic ring in which 80% relaxation occurred, were regarded as tissues with endothelium. When the PE-induced contraction reached a plateau, each sample (1 – 3, 10-4 M) was added.
These animal experimental studies were conducted in accordance with the Guiding Principles for the Care and Use of Laboratory Animals, Hoshi University and under the supervision of the Committee on Animal Research of Hoshi University, which is accredited by the Ministry of Education, Science, Sports Culture, and Technology of Japan.
ACKNOWLEDGMENTS
This work was supported by a Grant-in-Aid for Scientific Research from the Ministry of Education, Culture, Sports, Science, and Technology of Japan, and grants from the Research Foundation for pharmaceutical Sciences and The Open Research Center Project. We also acknowledge the financial support provided by Chinese Academy of Sciences, People’s Republic of China.
References
1. N. G. Bisset, J. Ethnopharmacol., 1981, 4, 247. CrossRef
2. D. Y. Gu, H. A. Aisa, and S. K. Usmanova, Chemistry of Natural Compounds, 2007, 43, 298; CrossRef Y. Yang, D. Gu, H. Wu, H. A. Aisa, T. Zhang, and Y. Ito, J. Liq. Chromatogr. & Related Techno., 2008, 31, 3012. CrossRef
3. P. Dobelis, J. E. Madl, J. A. Pfister, G. D. Manners, and J. P. Walrond, J. Pharmacology and Experimental Therapeutics, 1999, 291, 538; J. A. Pfister, D. R. Gardner, K. E. Panter, G. D. Manners, M. H. Ralphs, B. L. Stegelmeier, and T. K. Schoch, J. Natural Toxins, 1999, 8, 81.
4. F. Shaheen, M. Ahmad, M. T. H. Khan, S. Jalil, A. Ejaz, M. N. Sultankhodjaev, M. Arfan, M. I. Choudhary, and A. Rahman, Phytochemistry, 2005, 66, 935. CrossRef
5. A. J. Jones and M. H. Benn, Can. J. Chem., 1973, 51, 486. CrossRef
6. J. Golik, H. Wong, B. Krishnan, D. M. Vyas, and T. W. Doyle, Tetrahedron Lett., 1991, 32, 1851. CrossRef
7. H. Morita, T. Iizuka, C. Y. Choo, K. L. Chan, K. Takeya, and J. Kobayashi, Bioorg. Med. Chem. Lett., 2006, 16, 4609. CrossRef
8. M. Mitamura, S. Horie, M. Sakaguchi, A. Someya, S. Tsuchiya, J. Van de Voorde, T. Murayama, and K. Watanabe, European Journal of Pharmacology, 2002, 436, 217. CrossRef