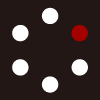
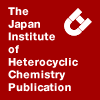
HETEROCYCLES
An International Journal for Reviews and Communications in Heterocyclic ChemistryWeb Edition ISSN: 1881-0942
Published online by The Japan Institute of Heterocyclic Chemistry
e-Journal
Full Text HTML
Received, 22nd September, 2009, Accepted, 9th November, 2009, Published online, 10th November, 2009.
DOI: 10.3987/COM-09-S(S)132
■ Heteroaromatic Trapping of Tricyclic 2-Oxidocyclopentenyl Cations: A Surprisingly Efficient Example of Intermolecular Interrupted Nazarov Reaction
Curtis J. Rieder, Ryan J. Fradette, and Frederick G. West*
Gunning/Lemieux Chemistry Center, Department of Chemistry, University of Alberta, Edmonton, Alberta T6G 2G2, Canada
Abstract
Bis(cycloalkenyl) ketones 2a and 2f underwent Nazarov cyclization and intermolecular trapping by electrophilic aromatic substitution with furans, thiophenes, pyrroles, indoles and dimethoxybenzene. Only the cis-anti-cis diastereomer was isolated with dicyclopentenyl ketone 2a, whereas a mixture of diastereomers was seen with 2f (albeit with complete regioselectivity). Comparable interrupted Nazarov trapping was not seen with acyclic dienones 2b-e, indicating the possible involvement of conformation effects in the polycyclic intermediates derived from 2a and 2f.INTRODUCTION
Introduction of an aryl group adjacent to a ketone is a potentially valuable transformation. A number of methods based on metal catalyzed cross-coupling reactions using aryl halides are available,1 as well as nucleophilic aromatic substitution2 and benzyne-mediated3 strategies. On the other hand, it is difficult to take advantage of straightforward electrophilic aromatic substitution chemistry due to the difficulty in accessing the necessary electrophile. The interrupted Nazarov reaction offers a strategy for gaining umpollung type reactivity α to a cyclopentanone carbonyl group (Scheme 1).4 Cross-conjugated dienones undergo Lewis acid-mediated electrocyclic closure to form 2-oxidocyclopentenyl cations 1 that can be intercepted with a variety of intra- or intermolecular nucleophiles. The resulting cyclopentanone products are bonded to the former nucleophile at the 2 position, equivalent to having reacted via a formal α-keto carbocation.
While there are several examples of intramolecular trapping by electron-rich arenes,5 effective intermolecular interception by simple aromatic traps remained elusive until recently. In 2008, we reported that dienone substrates in which both alkenes are encased within 5- or 6-membered rings can be trapped by unsubstituted electron-rich heteroaromatic compounds and 1,3-dimethoxybenzene.6 Shortly after, Basak and Tius disclosed a related process in which siloxy-substituted dienynes underwent Brønsted acid-mediated Nazarov cyclization with trapping of the intermediate by substituted indoles.7 A conceptually similar process was also described by Föhlisch and Joachimi, in which an unsubstituted 2-oxidocyclopentenyl cation generated by ionization of 2-chlorocyclopentanone was trapped by 2-methylfuran (eq 1).8 Here we report a full account of our studies, including the results with a number of substituted heteroaromatic traps.
RESULTS AND DISCUSSION
Our initial studies employed the known dicyclopentenyl ketone 2a (Scheme 2), a Nazarov substrate that had been successfully trapped with allylsilanes in a formal 3+2 cycloaddition.9 In an attempt to broaden its reactivity scope to include the corresponding [4+3]-cycloaddition,10 this dienone was stirred with one equiv each of furan and BF3•OEt2 at room temperature, resulting in complete consumption of 2a after 2 h. Surprisingly, however, neither of the two isolated products was the expected [4+3]-cycloadduct. Instead, products 3a and 4a resulting from trapping by simple electrophilic aromatic substitution reaction were obtained in a combined 85% yield. The basic connectivity of these adducts, entailing a bridgehead 2-furyl substituent on a linear triquinane, was determined via standard 2-dimensional NMR experiments. The cis-anti-cis relative stereochemistry assigned to the triquinane skeleton was inferred on the basis of the well established conrotatory electrocyclization mechanism of the Nazarov cyclization11 (leading to anti intermediate 1a), and the previously described preference for nucleophilic attack cis to the adjacent bridgehead substituent to provide the less strained ring fusion9 (confirmed by the observation of TROESY correlations between the the 3-furyl proton and the neighboring bridgehead proton). Finally, protonation of the resulting enolate 5 cis to the neighboring bridgehead proton to furnish the more stable cis-fused skeleton is expected to be the major or exclusive stereochemical outcome, due to the large enthalpic advantage. The stereochemical relationship between the two triquinane units in 4a could not be determined, due to the distance separating them.
While 2:1 adduct 4a possesses a unique and potentially useful structure, the simple 1:1 adduct 3a was of more immediate interest, as this was the first observation of intermolecular arene trapping of a Nazarov intermediate. To optimize the formation of the 1:1 adduct, we increased the amount of furan to 10 equiv, and under these conditions only 3a was obtained. These conditions were then applied to dienone 2a and a variety of simple heteroarenes (Table 1), although with traps other than furan 2 equiv was sufficient to assure exclusive formation of 1:1 adducts 3. Thiophene, N-tosylpyrrole and N-benzylpyrrole cleanly trapped intermediate 1a to provide adducts 3b-d. Substituted thiophenes and furans also provided arylated products (3e-g) as single regioisomers, although in only modest yields in the case of 2,5-dimethylfuran (entry 7). In analogy to the results described by the Tius group,7 N-protected indole and 5-methoxyindole underwent trapping at the 3 position in high yields (entries 8 and 9). To further test the limits of this method, we also examined two electron-rich benzene systems (entries 10 and 11). 1,3-Dimethoxybenzene proved to be a highly effective trap, providing 2,4-dimethoxyphenyl-substituted triquinane 3j in good yield. However, no trapping was seen with the less activated anisole. Instead, the simple eliminative Nazarov product 6a was obtained in good yield.
The generally efficient formation of adducts 3a-j raised a question: why did the cationic intermediate 1a derived from dienone 2a consistently undergo bimolecular arene trapping? Nazarov intermediates from acyclic dienones were already known to react with furan in [4+3]-cycloadditions.10 For example, dienones 2b-d all furnished keto-bridged cyclooctenes 7b-d in good yield, with no evidence of simple
arene trapping (Scheme 3). However, treatment of 2b with heteroarenes possessing less diene character such as thiophene or N-tosylpyrrole did not result in any simple arylation products analogous to 3, as was also the case with dimethoxybenzene. A possible rationale for the differing reactivity of 2a and 2b with these arenes centers on the lifetime of the cationic intermediate following Nazarov electrocyclization. The polycyclic 1a may persist long enough to undergo bimolecular trapping because the conventional elimination pathway is disfavored as a result of diminished conformational mobility.12 On the other hand, with furan 1a may not be able to undergo [4+3]-cycloaddition due to the greater steric demand likely to be present in the transition state for this concerted process.
With these differences in mind, we also examined the more hindered dienone 2e, a substrate which had shown broad generality in a variety of trapping processes. In the event, no adducts were seen with the heteroarene traps. However, with dimethoxybenzene an interesting new product was isolated in good yield. This product clearly contained elements of both reactants, with one fewer arene proton suggesting some sort of aromatic substitution process. Notably, there was no quaternary center adjacent to the cyclopentanone carbonyl, suggesting that trapping in analogy to 2a to give 3l had not occurred. Moreover, one of the former methyl groups flanking the carbonyl was missing, and an unexpected benzylic methylene was present, adjacent to one of the cyclopentanone α carbons. Based on these data, we assigned structure 8 to this adduct. The all-trans relative configuration was supported by 1H NMR coupling constants.6
Adduct 8 may arise via conjugate addition of dimethoxybenzene to methylidenecyclopentanone 9, formed by exocyclic elimination of the cyclized intermediate 1e (Scheme 4). In general, elimination of the Nazarov intermediate occurs in an endocyclic mode to form the most substituted (Saytzeff) alkene. However, in this case the alternative elimination may be favored to avoid the severe steric congestion resulting from enone 6e.13 The lack of enone β substituents on 9 would then permit the subsequent conjugate addition to occur.14,15
Since arene effective trapping of the Nazarov intermediate was not possible with acyclic dienones, we next chose to examine the higher homologue of 2a, cyclohexenyl cyclopentenyl ketone 2f, prepared in two steps from cyclohexenecarboxaldehyde (eq 2). This unsymmetrical example was expected to address several interesting questions. First, would the presence of one larger ring shorten the lifetime of the cationic intermediate through greater conformational mobility, and hence cause decreased efficiency in the bimolecular trapping process? Second, if trapping occurred, would any regioselectivity be observed (i.e., trapping at the diquinane or the hydrindan bridgehead)? Finally, would there be any erosion in the complete stereoselectivity in favor of the cis-anti-cis ring-fusion stereochemistry observed with 2a?
Treatment of 2f with the same heteroarenes used with 2a furnished good yields of the adducts 10 and 11 (Table 2), except in the case of 2,5-dimethylfuran (entry 7). As before, complete regioselectivity was observed with respect to substitution on the arene. However, in all successful cases, inseparable mixtures of adducts were obtained, in ratios of 2:1–5:1. Two possibilities were considered for the source of the mixtures: (1) regioisomeric trapping of 1f at the two bridgehead positions (i.e., A vs. B, Scheme 5), or diastereomeric mixtures of a single regioisomer (i.e., A vs. A’). Fortuitously, with 1,3-dimethoxybenzene, the adducts (10j and 11j) were separable. Examination of the mass spectral fragmentation patterns of these compounds revealed an important correlation with that of 3j. Specifically, 3j (and other triquinane adducts derived from 2a) undergo loss of a neutral C5H8CO fragment, presumably producing arylcyclopentenyl cation radical 12j (m/z 204.115). Both 10j and 11j displayed the same fragment (the base peak) in their mass spectra, corresponding to loss of C6H10CO. With the observation of the same aryl-containing cation radical from all three adducts, it can be inferred that both 10j and 11j are substituted with a dimethoxyphenyl group at the diquinane bridgehead rather than the hydrindan bridgehead, and hence must differ in their ring fusion relative configuration. Given
the low likelihood of a trans diquinane ring fusion, a mixture of cis- and trans-fused hydrindans, arising from protonation of either face of the enolate precursor, was considered. If this position were the only point of difference, it might be possible to interconvert 10j and 11j through base-mediated epimerization.16 In the event, treatment of either pure isomer with DBU resulted in identical equilibrium mixtures of 10j and 11j, offering strong evidence for the structural assignment. The same fragmentation pattern was seen in the mass spectra of all of the other adduct 10 and 11, and they were also assigned as epimers in analogy to 10j and 11j.
Finally, the contrast between dienones 2a and 2f vs acyclic dienones 2b-e prompted the examination of a hybrid substrate 2g with a single cyclopentene ring17 (eq 3). Although the cationic intermediate in this case would not be tricyclic, it would possess the electrophilic diquinane bridgehead site that was crucial for trapping in 2a and 2f. In the event, when 2g was treated with 1,3-dimethoxybenzene under the standard conditions, two adducts were obtained in good yield, and in a ratio of 5:1. While the minor isomer could not be obtained in sufficient purity to be characterized, the major product 13 was found to be a single diastereomer from trapping at the diquinane bridgehead position.18 The minor product is inferred to be the epimer of 13, in analogy to the mixtures obtained from 2f.
CONCLUSIONS
Cross-conjugated dienones 2a and 2f were found to undergo a high-yielding and general interrupted Nazarov process, in which the tricyclic cationic intermediates were trapped by a variety of heteroaromatic nucleophiles. Both substrates were also trapped by 1,3-dimethoxybenzene. This process entails a direct α-arylation adjacent to a cyclopentanone carbonyl group, giving rise to linearly fused bridgehead-arylated products. For the unsymmetrical substrate 2f, complete regioselectivity was seen in favor of attack at the diquinane bridgehead. Acyclic dienones did not undergo an analogous trapping process, but in one case a novel adduct resulting from conjugate addition of dimethoxybenzene to an α-methylenecyclopentanone resulting from conventional Nazarov elimination was observed. A monocyclic substrate, 2g, also underwent trapping with dimethoxybenzene, again at the diquinane bridgehead.
EXPERIMENTAL
General Information. Reactions were carried out in flame-dried glassware under a positive nitrogen atmosphere unless otherwise stated. Transfer of anhydrous solvents and reagents was accomplished with oven-dried syringes or cannulae. Solvents were distilled before use: methylene chloride from calcium hydride, tetrahydrofuran, diethylether and benzene from sodium/benzophenone ketyl, toluene from sodium metal. Thin layer chromatography was performed on glass plates precoated with 0.25 mm Kieselgel 60 F254 (Merck). Liquid chromatography-mass spectrometry (LC-MS) was carried out using Agilent-1100 series. Flash chromatography column were packed with 230-400 mesh silica gel (Silicycle). Proton nuclear magnetic resonance spectra (1H NMR) were recorded at 300 MHz, 400 MHz, or 500 MHz on Varian Inova 300, Inova 400, Mercury 400, Inova 500 and Unity 500 instruments. Coupling constants (J) are reported in Hertz (Hz). The chemical shifts are reported on the δ scale (ppm) and the spectra are referenced to tetramethylsilane (0 ppm, 1H; 13C) or to deuteriochloroform (7.26 ppm, 1H; 77.23 ppm, 13C) as internal standard. Carbon nuclear magnetic resonance spectra (13C NMR) were recorded on the same instruments at 100 MHz or 125 MHz. Infrared (IR) spectra were measured with a Mattson Galaxy Series FT-IR 3000 spectrophotometer. Mass spectra were determined on a PerSeptive Biosystem Mariner high-resolution electrospray spectrometer in the positive mode.
Standard Procedure for Arene Trapping. BF3⋅OEt2 (0.11 mmol) was added to a stirred solution of dienone (0.10 mmol) and arene (0.20 mmol) in CH2Cl2 (at a concentration of 10 mM) at room temperature. After 30 minutes the reaction was worked up with aq. saturated NaHCO3 (5 mL). The organic layer was drawn off and the aqueous phase was extracted with CH2Cl2 (3 x 5 mL). The combined organic phase was then dried over MgSO4, concentrated and purified via flash chromatography (10% EtOAc in hexanes).19
3d: 20mg (64%): pale yellow oil; IR (film) 3100, 30.62, 3029, 2952, 2866, 1730, 1605, 1469, 1477, 1452, 1420, 1329, 1298, 1086, 1030, 713 cm-1; 1H NMR (500MHz, CDCl3) δ 7.31 (m, 2H), 7.25 (m, 1H), 7.05 (d, 2H, J = 7.4 Hz), 6.51 (dd, 1H, J = 2.7, 1.9 Hz), 6.07 (dd, 1H, J = 3.5, 3.0 Hz), 6.00 (dd, 1H, J = 3.6, 1.8 Hz), 5.26 (d, 1H, J = 16.2 Hz), 5.21 (d, 1H, J = 16.2 Hz), 2.91 (ddd, 1H, J = 9.6, 4.9, 4.9 Hz), 2.66 (m, 1H), 2.35 (dddd, 1H, J = 9.0, 9.0, 4.5, 4.5 Hz), 2.13 (m, 1H), 2.04 (m, 2H), 1.84 (m, 3H), 1.74 (m, 3H), 1.57 (m, 2H), 1.46 (m, 1H); 13C NMR (125MHz, CDCl3) δ 221.9, 139.2, 132.8, 128.5, 127.2, 126.8, 123.6, 106.9, 106.9, 62.8, 53.6, 52.3, 51.8, 45.3, 37.8, 34.1, 33.4, 30.2, 25.7, 25.1; HRMS calc for C22H25NO (M+) 319.1936; found 319.1932 (68%), 223.1361 [M-C6H8O]+ (100%).
3e: 21mg (81%): pale yellow oil; IR (film) 2950, 2868, 1736, 1679, 1612, 1448, 1223, 1153, 945, 801 cm-1; 1H NMR (400MHz, CDCl3) δ 5.91 (s, 1H), 2.86 (ddd, 1H, J = 9.4, 9.4, 4.6 Hz), 2.67 (ddd, 1H, J = 7.3, 3.3, 3.3), 2.33 (m, 1H), 2.16 (s, 3H), 2.07 (m, 3H), 1.93 (m, 3H), 1.90 (s, 3H), 1.77 (m, 1H), 1.68 (m, 2H), 1.58 (m, 3H); 13C NMR (100MHz, CDCl3) δ 222.9, 153.6, 146.8, 114.5, 109.1, 63.0, 53.4, 52.6, 46.7, 37.9, 35.3, 34.6, 30.4, 26.1, 26.0, 11.6, 10.1; HRMS calc for C17H22O2 (M+) 258.1620; found 258.1615 (40%), 162.1045 [M-C6H8O]+ (100%).
3f: 21mg (81%): pale yellow oil; IR (film) 3060, 2950, 2866, 1734, 1653, 1552, 1470, 1448, 1230, 1166, 1107, 1060, 769 cm-1; 1H NMR (400MHz, CDCl3) δ 6.68 (d, 1H, J = 3.6 Hz), 6.58 (m, 1H), 2.90 (ddd, 1H, J = 8.7, 5.6, 5.6 Hz), 2.69 (ddd, 1H, J = 7.3, 3.3, 3.3 Hz), 2.44 (s, 3H), 2.38 (dddd, 1H, J = 8.1, 8.1, 4.3, 4.3 Hz), 2.19 (m, 2H), 2.04 (ddd, 1H, J = 13.6, 7.0, 7.0 Hz), 1.89 (m, 3H), 1.74 (m, 3H), 1.55 (m, 3H); 13C NMR (100MHz, CDCl3) δ 222.7, 145.0, 138.5, 124.9, 123.6, 64.3, 55.3, 53.1, 46.7, 42.4, 35.2, 34.2, 30.6, 26.1, 26.0, 15.5; HRMS calc for C16H20OS (M+) 260.1235; found 260.1236 (44%), 164.0660 [M-C6H8O]+ (100%).
3g: 16mg (31%): pale yellow oil; IR (microscope) 2950, 2868, 1732, 1679, 1623, 1577, 1450, 1328, cm-1; 1H NMR (400MHz, CDCl3) δ 5.77 (s, 1H), 2.89 (ddd, 1H, J = 8.2, 8.2, 8.2 Hz), 2.46 (m, 1H), 2.34 (dddd, 1H, J = 8.7, 8.7, 4.4, 4.4 Hz), 2.26 (s, 3H), 2.21 (s, 3H), 2.15 (m, 1H), 2.00 (m, 2H), 1.87 (m, 3H), 1.76 (m,3H), 1.58 (m, 1H), 1.52 (m, 2H); 13C NMR (100MHz, CDCl3) δ 223.6, 148.6, 146.0, 121.5, 107.1, 61.1, 54.1, 52.7, 46.0, 39.4, 34.4, 34.3, 30.4, 26.0, 25.5, 14.0, 13.6; HRMS calc for C17H22O2 (M+) 258.1620; found 258.1615 (56%), 162.1045 [M-C6H8O]+ (100%).
3h: 31mg (84%): pale yellow oil; IR (film) 3087, 3061, 3031, 2949, 2865, 1728, 1612, 1542, 1496, 1481, 1467, 1453, 1371, 1355, 1335, 1199, 1180, 1017, 736, 696 cm-1; 1H NMR (400MHz, CDCl3) δ 7.69 (d, 1H, J = 7.9 Hz), 7.28 (m, 4H), 7.17 (ddd, 1H, J = 4.2, 6.9, 8.1 Hz), 7.12 (m, 1H), 7.08 (m, 2H), 6.88 (s, 1H), 5.29 (d, 1H, J = 16.2 Hz), 5.26 (d, 1H, J = 16.2 Hz), 2.91 (m, 2H), 2.46 (dddd, 1H, J = 8.7, 8.7, 4.4, 4.4 Hz), 2.28 (m, 3H), 1.82 (m, 6H), 1.47(m, 3H); 13C NMR (100MHz, CDCl3) δ 225.4, 137.9, 137.8, 128.9, 127.7, 126.9, 126.6, 125.8, 122.0, 121.2, 119.2, 117.3, 110.2, 62.2, 53.2, 52.8, 50.2, 46.7, 39.7, 35.4, 34.8, 31.1, 26.2, 26.1; HRMS calc for C26H27NO (M+) 369.2093; found 369.2096 (50%), 273.1518 [M-C6H8O]+ (100%).
3i: 34mg (85%): brown oil; IR (film) 3063, 3030, 2948, 2866, 1228, 1621, 1575, 1487, 1451, 1355, 1343, 1288, 1258, 1222, 1180, 1043, 1030, 793, 731, 705 cm-1; 1H NMR (400MHz, CDCl3) δ 7.28 (m, 3H), 7.13 (m, 2H), 7.07 (m, 2H), 6.87 (s, 1H), 6.84 (dd, 1H, J = 8.9, 2.4 Hz), 5.53 (br s, 2H), 3.88 (s, 3H), 2.93 (ddd, 1H, J = 9.2, 9.2, 5.1 Hz), 2.85 (ddd, 1H, J = 7.3, 3.2, 3.2 Hz), 2.46 (dddd, 1H, J = 8.5, 8.5, 4.2, 4.2 Hz), 2.25 (m, 3H), 1.85 (m, 6H), 1.51 (m, 3H); 13C NMR (100MHz, CDCl3) δ 225.2, 153.7, 137.9, 133.3, 128.9, 127.7, 127.0, 126.8, 126.5, 116.6, 111.9, 110.9, 103.5, 62.3, 56.1, 53.1, 52.8, 50.4, 46.5, 39.1, 35.2, 34.8, 31.1, 26.2, 25.9; HRMS calc for C27H29NO2 (M+) 399.2198; found 399.2196 (28%), 303.1623 [M-C6H8O]+ (69%), 91.0548 [M-C20H22NO2]+ (100%).
10d and 11d: 52 mg (78%) isolated as a 2:1 mixture of diastereomers. Small quantities of pure diastereomers were obtained using radial chromatography.
10d: off-white solid; IR (film) 3108, 3037, 2997, 2930, 2861, 1727, 1686, 1498, 1477, 1466, 1457, 1447, 1376, 1264, 1236, 1087, 1028, 773, 708 cm-1; 1H NMR (500MHz, CDCl3) δ 7.31 (m, 2H), 7.25 (m, 1H), 7.06 (m, 2H), 6.52 (dd, 1H, J = 2.3, 2.3 Hz), 6.07 (d, 2H, J = 2.3 Hz), 5.51 (d, 1H, J = 16.1 Hz), 5.43 (d, 1H, J = 16.1 Hz), 3.07 (ddd, 1H, J = 8.3, 4.9, 4.4 Hz), 2.57 (dddd, 1H, J = 6.5, 6.5, 6.5, 6.5 Hz), 2.07 (m, 2H), 1.98 (m, 2H), 1.83 (m, 1H), 1.65 (m, 4H), 1.52 (m, 2H), 1.34 (m, 4H); 13C NMR (125MHz, CDCl3) δ 219.3. 139.7, 134.7, 128.5, 127.0, 126.7, 123.8, 106.8, 106.6, 59.6, 52.2, 50.6, 48.9, 39.3, 39.1, 32.5, 28.3, 26.6, 23.7, 23.4, 23.1; HRMS calc for C23H27NO (M+) 333.2093; found 333.2093 (40%), 223.1364 [m-C7H10O]+ (100%).
11d: pale yellow oil; IR (film) 3063, 3030, 2930, 2856, 1733, 1605, 1496, 1476, 1450, 1359, 1294, 1157, 1086, 1076, 1029, 727 cm-1; 1H NMR (500MHz, CDCl3) δ 7.30 (m, 2H), 7.24 (m, 1H), 7.04 (m, 2H), 6.50 (dd, 1H, J = 2.8, 1.8 Hz), 6.10 (dd, 1H, J = 3.7, 1.9 Hz), 6.07 (dd, 1H, J = 3.7, 2.8 Hz), 5.53 (d, 1H, J = 16.1 Hz), 5.28 (d, 1H, J = 16.1 Hz), 2.85 (dd, 1H, = 10.1, 7.2 Hz), 2.39 (m, 1H), 2.23 (ddd, 1H, J = 14.0, 10.8, 3.4 Hz), 2.11 (m, 1H), 1.91 (m, 1H), 1.81 (m, 2H), 1.68 (m, 4H), 1.47 (m, 1H), 1.16 (m, 4H), 0.90 (m, 1H); 13C NMR (125MHz, CDCl3) δ 215.6, 139.4, 134.8, 128.5, 127.1, 126.8, 124.1, 107.0, 106.4, 60.2, 52.4, 52.1, 51.5, 43.4, 35.4, 32.1, 29.7, 26.2, 25.9, 25.1, 25.0; HRMS calc for C23H27NO (M+) 333.2093; found 333.2093 (40%), 223.1362 [m-C7H10O]+ (100%).
10e and 11e: 46 mg (84%) as an inseparable mixture of diastereomers (2:1): brown oil; IR (film) 2930, 2858, 1741, 1640, 1561, 1448, 1386, 1361, 1293, 1271, 1224, 1158, 1030, 949, 810 cm-1; 1H NMR (500MHz, CDCl3) δ 5.90 (s, 1H), 5.84 (s, 0.5H), 2.72 (ddd, 1H, J = 8.9, 4.8, 4.8 Hz), 2.59 (ddd, 1H, J = 6.1, 6.1, 6.1 Hz), 2.54 (dd, 0.5H, J = 10.2, 6.4 Hz), 2.17 (m, 1.9H), 2.15 (s, 3.3H), 2.13 (2, 1.7H), 2.09 (m, 1.3H), 1.97 (m, 2.5H), 1.88 (s, 3.6H), 1.86 (s, 2.0H), 1.72 (m, 6.4H), 1.54 (m, 4H), 1.39 (m, 1H), 1.20 (m, 5.7H); 13C NMR (125MHz, CDCl3) δ 218.6, 215.2, 153.7, 153.3, 146.6, 146.3, 114.4, 114.2, 108.6, 108.0, 60.9, 60.0, 54.1, 52.1, 50.5, 47.7, 44.0, 39.8, 38.7, 33.6, 33.3, 31.8, 30.1, 28.8, 26.9, 26.3, 25.4, 25.3, 25.2, 23.6, 23.3, 22.9, 11.4, 11.4, 9.9, 9.8; HRMS calc for C18H24O2 (M+) 272.1776; found 272.1777 (40%), 162.1043 [M-C7H10O]+ (100%).
10f and 11f: 47 mg (86%) as an inseparable mixture of diastereomers (2:1): brown oil; IR (film) 2930, 2857, 1739, 1551, 1448, 1330, 1293, 1227, 1661, 1029, 796 cm-1; 1H NMR (500MHz, CDCl3) δ 6.75 (d, 1H, J = 3.5 Hz), 6.65 (d, 0.5H, J = 3.5 Hz), 6.57 (m, 1.4H), 2.82 (ddd, 1H, J = 9.0, 4.6, 4.6 Hz), 2.61 (m, 1.6H), 2.45 (d, 3H, J = 1.1 Hz), 2.43 (d, 1.5H, J = 1.1 Hz), 2.37 (ddd, 0.6H, J = 12.2, 7.8, 4.0 Hz), 2.18 (m, 2.2H), 2.04 (m, 3.3H), 1.87 (m, 3.4H), 1.71 (m, 4.6H), 1.52 (m, 1.6H), 1.39 (m, 1H), 1.24 (m, 4.6H); 13C NMR (125MHz, CDCl3) δ 219.0, 215.1, 146.1(appears to be two overlapping signals), 138.1, 138.0, 124.9, 124.5, 123.0, 122.7, 62.3, 61.3, 54.5, 53.3, 52.8, 47.9, 44.5, 43.5, 40.0, 38.0, 33.1, 31.8, 30.4, 28.9, 26.8, 26.3, 25.7, 25.3, 25.2, 23.4, 23.3, 23.2, 15.2, 15.2; HRMS calc for C17H22OS (M+) 274.1391; found 274.1390 (38%), 164.0657[M-C7H10O]+ (100%); Anal. Calc. for C17H22OS: C, 74.40; H, 8.08, S, 11.68. Found: C, 74.48; H, 8.42; S, 11.81.
10h and 11h: 63 mg (82%) isolated as a 2:1 mixture of diastereomers. Small quantities of the major diastereomer were obtained pure using radial chromatography while 11h was enriched to a 3:5 mixture.
10h: pale yellow oil; IR (film) 3062, 3031, 2931, 2857, 1730, 1611, 1496, 1480, 1466, 1453, 1356, 1335, 1179, 909, 737, 696 cm-1; 1H NMR (500MHz, CDCl3) δ 7.81 (d, 1H, J = 8.0 Hz), 7.26 (m, 4H), 7.16 (ddd, 1H, J = 7.0, 7.0, 1.2 Hz), 7.11 (ddd, 1H, J = 7.0, 7.0, 1.2 Hz), 7.07 (m, 2H), 7.00 (s, 1H), 5.29 (d, 1H, J = 16.2 Hz), 5.24 (d, 1H, J = 16.2Hz), 3.03, (ddd, 1H, J = 9.3, 3.6, 3.6 Hz), 2.56 (ddd, 1H, J = 6.3, 6.3, 6.3 Hz), 2.39 (dddd, 1H, J = 13.0, 9.1, 9.1, 7.8 Hz), 2.32 (ddd, 1H, J = 13.1, 9.4, 7.1 Hz), 2.21 (ddd, 1H, J = 11.6, 6.8, 4.6 Hz), 2.10, (m, 1H), 2.04 (dddd, 1H, J = 13.8, 4.6, 4.6, 4.6 Hz), 1.86 (m, 1H), 1.76 (ddd, 1H, J = 11.6, 7.7, 4.2 Hz), 1.63 (m, 2H), 1.50 (dddd, 1H, J = 17.4, 10.7, 6.1, 4.1 Hz), 1.42 (m, 2H), 1.17 (m, 1H), 1.09 (m, 2H); 13C NMR (125MHz, CDCl3) δ 222.9, 138.0, 137.9, 129.0, 127.8, 126.9, 126.8, 125.9, 122.0, 121.4, 119.2, 118.8, 110.3, 59.7, 50.4, 50.3, 49.4, 41.6, 41.4, 34.3, 29.9, 27.5, 24.3, 23.8, 23.8; HRMS calc for C27H29NO (M+) 383.2249; found 383.2247 (82%), 273.1525 [M-C7H10O]+ (100%).
11h: pale yellow oil; 1H NMR (500MHz, CDCl3) δ 7.91 (d, 1H, J = 8.0 Hz), 7.33-7.21 (m, 4H), 7.16 (m, 1H), 7.11 (m, 2H), 7.07 (m, 1H), 6.92 (s, 1H), 5.29 (d, 1H, J = 16.2), 5.24 (d, 1H, J = 16.2), 2.78 (dd, 1H, J = 9.7, 7.6), 2.56 (m, 1H), 2.23 (m, 1H), 2.11 (m, 1H), 2.06-1.95 (m, 1H), 1.89-1.72 (m, 4H), 1.54-1.37 (m, 2H), 1.29 (m, 1H), 1.25-1.03 (m, 5H); 13C NMR (125MHz, CDCl3), minor product signals only, δ 216.9, 137.9, 137.8, 128.0, 127.9, 127.0, 126.7, 125.0, 122.3, 121.7, 119.6, 119.4, 110.2, 60.3, 52.9, 51.6, 50.3, 45.2, 36.6, 32.4, 31.2, 26.7, 26.1, 25.6, 25.5.
10i and 11i: 68 mg (82%) as an inseparable 5:1 mixture of diastereomers): pale yellow oil; IR (film) 3063, 3030, 2933, 2857, 1731, 1621, 1575, 1486, 1450, 1356, 1343, 1290, 1217, 1180, 1030, 839, 794, 736, 704 cm-1; 1H NMR (500MHz, CDCl3) δ 7.45 (d, 0.2H, J = 2.4 Hz), 7.34 (d, 1H, J = 2.4 Hz), 7.28 (m, 3.3H), 7.13 (d, 1H, J = 9.0 Hz), 7.08 (m, 2.4 Hz), 7.00 (s, 1H), 6.93 (s, 2H), 6.85 (m, 1.2H), 5.26 (d, 1H, J = 16.2Hz) 5.21 (d, 1.4H, J = 16.2 Hz), 3.91 (s, 0.6H), 3.90 (s, 3H), 3.03 (ddd, 1H, J = 9.0, 3.6, 3.6 Hz), 2.78 (dd, 0.2H, J = 9.2, 7.8 Hz), 2.59 (dd, 1.2H, J = 11.7, 5.9 Hz), 2.36 (m, 2H), 2.23 (m, 1.2H), 2.16 (m, 0.2H), 2.11 (m, 1H), 2.05 (m, 1.2H), 1.98 (m, 0.6H), 1.86 (m, 1.5H), 1.78 (m, 1.5H), 1.66 (m, 2.6H), 1.48 (m, 3.3), 1.19 (m, 3.9H); 13C NMR (125MHz, CDCl3) δ 222.4, 216.9, 153.8, 153.4, 153.4, 137.8, 137.6, 133.0, 128.7, 128.7, 127.5, 127.5, 126.8, 126.6, 126.5, 126.3, 125.2, 118.4, 117.9, 112.1, 111.4, 110.6, 110.5, 103.7, 103.5, 59.9, 59.4, 56.0, 55.9, 52.6, 51.1, 50.2, 50.1, 49.9, 49.1, 44.9, 41.1, 40.7, 36.0, 33.9, 32.1, 31.6, 30.8, 29.5, 27.1, 26.4, 25.8, 25.3, 25.2, 23.9, 23.5, 23.5; HRMS calc for C28H31NO2 (M+) 413.2355; found 413.2358 (30%), 303.1628 [M-C7H10O]+ (100%).
13: 17 mg (64%): colorless oil; IR (microscope) 2955, 2870, 2837, 1734, 1612, 1584, 1505, 1464, 1208, 1164, 1134, 1032 cm-1; 1H NMR (500MHz, CDCl3) δ 7.06 (d, 1H, J = 8.2 Hz), 6.45 (dd, 1H, J = 8.2, 2.5 Hz), 6.44 (d, 1H, J = 2.5 Hz), 3.79 (s, 3H), 3.71 (s, 3H), 2.74 (m, 1H), 2.68 (ddq, 1H, J = 11.8, 9.3, 7.1 Hz), 2.38 (ddd, 1H, J = 12.7, 9.2, 8.1 Hz), 2.18 (m, 1H), 1.84 - 1.95 (m, 2H), 1.75 – 1.84 (m, 2H), 1.58 (m, 1H), 1.21 (d, 3H, J = 7.1 Hz), 1.18 (ddd, 1H, J = 12.8, 11.8, 10.4 Hz); 13C NMR (125MHz, CDCl3) δ 221.6, 159.8, 157.4, 127.7, 124.0, 104.0, 99.6, 62.7, 55.3, 55.1, 47.9, 43.8, 36.4, 31.5, 30.5, 24.0, 15.8; HRMS calc for C17H22O3 (M+) 274.1569; found 274.1568 (23%), 204.1149 [M-C4H6O]+ (100%).
ACKNOWLEDGEMENTS
We thank the Natural Sciences and Engineering Research Council (NSERC) of Canada for generous support of this work.
References
1. J. M. Fox, X. Huang, A. Chieffi, and S. L. Buchwald, J. Am. Chem. Soc., 2000, 122, 1370; CrossRef P. Nilsson, M. Larhed, and A. Hallberg, J. Am. Chem. Soc., 2003, 125, 3430; CrossRef X. Liao, Z. Weng, and J. F. Hartwig, J. Am. Chem. Soc., 2007, 129, 195; CrossRef M. L. Hlavinka and J. R. Hagadorn, Organometallics, 2007, 26, 41052. CrossRef
2. R. J. Snow, T. Butz, A. Hammach, S. Kapadia, T. M. Morwick, A. S. Prokopowicz, H. Takahashi, J. Tan, M. A. Tschantz, and X.-J. Wang, Tetrahedron Lett., 2002, 43, 7553; CrossRef M. Bella, S. Kobbelgaard, and K. A. Jorgensen, J. Am. Chem. Soc., 2005, 127, 3670; CrossRef S. Kobbelgaard, M. Bella, and K. A. Jorgensen, J. Org. Chem., 2006, 71, 4980. CrossRef
3. S. Tripathy, R. Reddy, and T. Durst, Can. J. Chem., 2003, 81, 997; CrossRef U. K. Tambar and B. M. Stoltz, J. Am. Chem. Soc., 2005, 127, 5340. CrossRef
4. T. N. Grant, C. J. Rieder, and F. G. West, Chem. Commun., 2009, 5676. CrossRef
5. C. C. Browder, F. P. Marmsäter, and F. G. West, Org. Lett., 2001, 3, 3033; CrossRef C. C. Browder, F. P. Marmsäter, and F. G. West, Can. J. Chem., 2004, 82, 375; CrossRef T. N. Grant and F. G. West, J. Am. Chem. Soc., 2006, 128, 9348; CrossRef T. N. Grant and F. G. West, Org. Lett., 2007, 9, 3789. CrossRef
6. C. J. Rieder, R. J. Fradette, and F. G. West, Chem. Commun., 2008, 1572. CrossRef
7. A. K. Basak and M. A. Tius, Org. Lett., 2008, 10, 4073. CrossRef
8. B. Föhlisch and R. Joachimi, Chem. Ber., 1987, 120, 1951. CrossRef
9. S. Giese, L. Kastrup, D. Stiens, and F. G. West, Angew. Chem. Int. Ed., 2000, 39, 1970. CrossRef
10. Intermolecular [4+3] trapping of the Nazarov intermediate: Y. Wang, B. D. Schill, A. M. Arif, and F. G. West, Org. Lett., 2003, 5, 2747; CrossRef A. Yungai and F. G. West, Tetrahedron Lett., 2004, 45, 5629. CrossRef
11. R. B. Woodward and R. Hoffmann, Angew. Chem., Int. Ed. Engl., 1969, 8, 781. CrossRef
12. Similarly, the indole trapping described by Basak and Tius may be an especially effective process due to a longer lifetime conferred upon the cationic intermediate from additional conjugation.
13. For a discussion of steric effects on the regiochemistry of E1 eliminations, see: H. C. Brown and I. Moritani, J. Am. Chem. Soc., 1955, 77, 3623. CrossRef
14. Related alkylations of indoles via conjugate addition have been described: W. Chen, W. Du, L. Yue, R. Li, Y. Wu, L.-S. Ding, and Y.-C. Chen, Org. Biomol. Chem., 2007, 5, 816; CrossRef (b) L.-W. Xu, W. Zhou, L. Yang, and C.-G. Xia, Synth. Commun., 2007, 37, 3095. CrossRef
15. A structurally similar compound to 9 had been reported, prepared via arylcuprate 1,4-addition to a methylenecyclopentanone: A. B. Smith, III, B. A. Wexler, and J. S. Slade, Tetrahedron Lett., 1980, 21, 3237. CrossRef
16. Epimers 10j and 11j were distinguished by analysis of the coupling constants for the hydrindan bridgehead proton adjacent to the ketone. For the cis isomer 10j, two small couplings and one large trans-diaxial coupling were observed, while for trans isomer 11j, two large trans-diaxial couplings were observed.
17. See reference 10 for the preparation of 2g.
18. Major product 13 was assigned the indicated relative stereochemical configuration based upon vicinal coupling constants and the observation of several unambiguous rOe correlations in the 2D-TROESY spectrum.
19. Spectral data for 2f, 3a-c, 3j, 8, 10a-c, 11a-c, 10j and 11j are found in the electronic supplementary information accompanying reference 6.