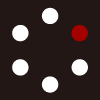
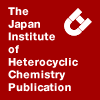
HETEROCYCLES
An International Journal for Reviews and Communications in Heterocyclic ChemistryWeb Edition ISSN: 1881-0942
Published online by The Japan Institute of Heterocyclic Chemistry
e-Journal
Full Text HTML
Received, 25th June, 2009, Accepted, 18th August, 2009, Published online, 18th August, 2009.
DOI: 10.3987/COM-09-S(S)40
■ Highly Active Rhodium Catalyst with Electron-Poor Diphosphine Enables Efficient Synthesis of Chiral 4-Aryl-δ-lactones
Toshinobu Korenaga,* Ryota Maenishi, Kazutaka Osaki, and Takashi Sakai*
Department of Applied Chemistry, Graduate School of Natural Science and Technology, Okayama University, 1-1-1 Tsushima-naka, Okayama 700-8530, Japan
Abstract
Chiral 4-aryl-δ-lactones could be synthesized efficiently with high enantioselectivity through asymmetric 1,4-addition of arylboronic acid to α,β-unsaturated lactones using Rh catalyst including electron-poor diphosphine (MeO-F12-BIPHEP) at room temperature for 1 h. In particular, our catalytic system proved to be applicable to relatively large coumarin analogues, giving optically pure 4-phenylchroman-2-one analogues in a short time.Since Akira Suzuki and Norio Miyaura reported on the Suzuki-Miyaura coupling reaction in 1979,1 researchers have utilized arylboronic acids for metal catalyzed cross-coupling reactions.2 Furthermore, the utility of arylboronic acids has been further enhanced by the development of the rhodium catalyzed asymmetric 1,4-addition of arylboronic acid to an α,β-unsaturated ketone.3 Using these reactions, chiral 4-substituted-δ-lactones such as 4-phenyltetrahydro-2H-pyran-2-one (2) can also be synthesized from the α,β-unsaturated lactone, 5,6-dihydro-2H-pyran-2-one (1). The reactivity of 1 was equal to or less than that of 2-cyclohexenone (Scheme 1).4 The reaction usually requires 3 – 5 mol% Rh catalyst and a relatively higher reaction temperature (30 – 100 °C). In addition, the reaction at room temperature or with less catalyst loading required a longer reaction time. In contrast, highly active rhodium catalysts for the reaction of 1 even at room temperature were developed in 2008.5 Here, 1 or 2 mol% Rh catalysts with chiral diene ligand obtained (R)-2a in a high yield with high enantioselectivity at room temperature for 3 or 1 h, respectively. Recently, we have developed a new type of electron-poor MeO-F12-BIPHEP ligand, which highly accelerates the rhodium-catalyzed 1,4-addition (Scheme 1).6 The catalytic system enabled highly efficient reaction of 1 even at room temperature. Thus, the reaction of 1 with 4-CF3-C6H4-B(OH)2 at 20 °C for 1 h gave 90% optically pure (R)-4-(4-trifluorophenyl)-tetrahydro- 2H-pyran-2-one (2b), using only 0.5 mol% Rh catalyst. This paper reports that our previously developed catalytic system with electron-poor diphosphine ligand is successfully applied to the asymmetric 1,4-addition of arylboronic acid to yield α,β-unsaturated lactone under mild conditions, yielding highly optically active 4-aryl-δ-lactones.
First, the reactions of 1 with arylboronic acids were performed using 0.5 mol% Rh catalyst with (R)-MeO-F12-BIPHEP, based on the procedure reported in our previous paper6 (Table 1). Although the reaction of 1 with phenylboronic acid in the presence of the catalyst with KOH in toluene/H2O (10/1) at 20 °C for 1 h gave (R)-2a in an excellent yield, the enantioselectivity was less than 90% ee (85% ee, Entry 1). However, the use of substituted arylboronic acid gave excellent enantioselectivity with a high yield (Entry 2 – 7). Irrespective of the position of the substituent on the phenyl group and the electronic nature of the substituent, the enantioselectivity of (R)-2 reached at least 97% ee. In contrast to the reaction of 2-cyclohexenone,6 the enantioselectivity was susceptible to the steric effect of arylboronic acid.
Next, the reaction of coumarin analogues 3, which are bulkier substrates than 1, was focused upon because the resulting products are useful for biologically active compounds7 or their synthetic intermediates.8 The reaction of coumarin (3a) using the rhodium catalyzed asymmetric 1,4-addition has been reported only twice in the past. In the first report, the reaction in the presence of 3 mol% Rh catalyst with a chiral diene ligand at 50 °C gave (R)-4-phenylchroman-2-one (4a) in a 43% yield with 98% ee.9 In the second, researchers obtained almost optically pure (R)-4a (99.6% ee) in an 88% yield using 3 mol% Rh catalyst with (R)-Segphos and 10 equivalents of Ph-B(OH)2 in dioxane/H2O (10/1) at 60 °C for 8 h.8 In contrast to these reports, our catalytic system achieved the reaction smoothly under significantly milder conditions (Table 2). Thus, the reaction of 3a with 10 equivalents of Ph-B(OH)2 in the presence of 1 mol% Rh catalyst in toluene / sat. NaHCO3 aq. (1/1) at 30 °C for 1 h gave (R)-4a in a 94% yield with >99% ee (Entry 1),10 and HPLC analysis detected no (S)-enantiomer. In our catalytic system, a higher yield with sufficient enantioselectivity was obtained in a shorter reaction time (1 h) despite using a reduced amount of the catalyst under a lower temperature (30 °C). The result is attributed to the electronic effect of MeO-F12-BIPHEP, because the reaction using MeO-BIPHEP did not proceed at all (Entry 2).6 The catalyst loading could be reduced to 0.5 mol% to give (R)-4a still in a 90% yield without any loss of enantioselectivity (Entry 3). When 1.5 mol% Rh catalyst was used at 20 °C, (R)-4a was obtained in a 94% yield (Entry 4). Our highly reactive catalyst system enabled the reduction in the amount of Ph-B(OH)2, which is hydrolyzed at high temperatures. The use of 3 equivalents of Ph-B(OH)2 gave optically pure (R)-4a in a 90% yield, but 3 mol% Rh catalyst was necessary in this case (Entry 5). The optimized conditions in Entry 4 was then successfully applied to 6-methylcoumarin (3b) to obtain (R)-6-methyl-4-phenylchroman-2-one (4b),11 which can be converted into (R)-tolterodine used as a urological drug,8 in a 94% yield (>99% ee, Entry 6). Interestingly, 7-methoxycoumarin (3c) also smoothly reacted with Ph-B(OH)2 to obtain (R)-7-methoxy-4-phenylchroman-2-one (4c)12,13 in an 85% yield with >99% ee (Entry 7), even though 3c was inactive to the Rh-Segphos catalyst.8 These results show that the Rh / MeO-F12-BIPHEP complex acts as an extremely highly active catalyst for the asymmetric 1,4-addition to synthesize α,β-unsaturated lactones even when using less reactive substrates.
The optically pure 4-aryl-δ-lactones were synthesized efficiently using our catalytic system under mild conditions. In particular, the facts that both high reactivity and complete enantioselectivity were attained with relatively large substrates such as coumarin are promising indicators that our catalytic system could be widely utilized for the synthesis of natural and/or biologically active compounds in the future.
ACKNOWLEDGEMENTS
This work was supported by Mitsui Chemicals Award in Synthetic Organic Chemistry, Japan and a Grant-in-Aid for Young Scientists (B) from the Ministry of Education, Culture, Sports, Science and Technology, Japan, and JGC-S SCHOLARSHIP FOUNDATION. We thank the SC-NMR Laboratory of Okayama University for 1H, 13C, and 19F NMR measurements.
References
1. N. Miyaura, K. Yamada, and A. Suzuki, Tetrahedron Lett., 1979, 3437. CrossRef
2. N. Miyaura, Bull. Chem. Soc. Jpn, 2008, 81, 1535. CrossRef
3. Y. Takaya, M. Ogasawara, T. Hayashi, M. Sakai, and N. Miyaura, J. Am. Chem. Soc., 1998, 120, 5579. CrossRef
4. Rh-catalyzed asymmetric 1,4-addition of Ar-B(OH)2 to 1a: J. L. Nôtre, J. C. Allen, and C. G. Frost, Chem. Commun., 2008, 3795; CrossRef R. Mariz, X. Luan, M. Gatti, A. Linden, and R. Dorta, J. Am. Chem. Soc., 2008, 130, 2172; CrossRef C. Monti, C. Gennari, and U. Piarulli, Chem. Euro. J., 2007, 13, 1547; CrossRef W.-L. Duan, H. Iwamura, R. Shintani, and T. Hayashi, J. Am. Chem. Soc., 2007, 129, 2130; CrossRef K. Kurihara, N. Sugishita, K. Oshita, D. Piao, Y. Yamamoto, and N. Miyaura, J. Organomet. Chem., 2007, 692, 428; CrossRef P. Kasak, V. B. Arion, and M. Widhalm, Tetrahedron: Asymmetry, 2006, 17, 3084; CrossRef S. L. X. Martina, A. J. Minnaard, B. Hessen, and B. L. Feringa, Tetrahedron Lett., 2005, 46, 7159; CrossRef R. Shintani, W.-L. Duan, T. Nagano, A. Okada, and T. Hayashi, Angew. Chem. Int. Ed., 2005, 44, 4611; CrossRef Y. Otomaru, K. Okamoto, R. Shintani, and T. Hayashi, J. Org. Chem., 2005, 70, 2503; CrossRef K. Yoshida and T. Hayashi, Heterocycles, 2003, 59, 605; CrossRef T. Hayashi, M. Takahashi, and Y. Takaya, J. Am. Chem. Soc., 2002, 124, 5052; CrossRef M. T. Reetz, D. Moulin, and A. Gosberg, Org. Lett., 2001, 3, 4083; CrossRef Y. Takaya, T. Senda, H. Kurushima, M. Ogasawara, and T. Hayashi, Tetrahedron: Asymmetry, 1999, 10, 4047. CrossRef
5. T. Gendrineau, O. Chuzel, H. Eijsberg, J.-P. Genet, and S. Darses, Angew. Chem. Int. Ed., 2008, 47, 7669; CrossRef C.-G. Feng, Z.-Q. Wang, C. Shao, M.-H. Xu, and G.-Q. Lin, Org. Lett., 2008, 10, 4101. CrossRef
6. T. Korenaga, K. Osaki, R. Maenishi, and T. Sakai, Org. Lett., 2009, 11, 2325. CrossRef
7. I. Singh, A. K. Prasad, A. K. Sharma, R. K. Saxena, C. E. Olsen, A. L. Cholli, L. A. Samuelson, J. Kumar, A. C. Watterson, and V. S. Parmar, Bioorg. Med. Chem., 2003, 11, 529. CrossRef
8. G. Chen, N. Tokunaga, and T. Hayashi, Org. Lett., 2005, 7, 2285. CrossRef
9. C. Defieber, J.-F. Paquin, S. Serna, and E. M. Carreira, Org. Lett., 2004, 6, 3873. CrossRef
10. General procedure for the reactions of coumarin analogues: A 20 mL Schlenk flask was charged with (R)-MeO-F12-BIPHEP (12.4 mg, 15.5 μmol), μ-dichlorotetraethylene dirhodium(I) (3.0 mg, 7.7 μmol) and 0.5 mL of deoxygenated toluene under argon atmosphere. The mixture was stirred at room temperature for 5 min for complexation. To the mixture was added 2.5 mL of deoxygenated toluene, phenylboronic acid (1.89 g, 15.5 mmol), 2.0 mL of deoxygenated sat. NaHCO3 aq, and coumarin (3a, 227 mg, 1.55 mmol). After being stirred at 30 °C for 1 h, to the solution was added saturated aqueous NaHCO3 solution. The resulting mixture was extracted with EtOAc (three times). The organic layer was dried over MgSO4, filtrated with suction, and then concentrated under reduced pressure. The residue was purified by silica gel column chromatography (Hexane / EtOAc = 3/1) to give (R)-4-phenylchroman-2-one (4a) as a white solid (327 mg, 94% yield, >99% ee).
m.p. 114 – 115 °C; 1H NMR (300 MHz, CDCl3): δ 3.01 (dd, J = 14.7, 7.8 Hz, 1H), 3.08 (dd, J = 14.7, 7.8 Hz, 1H), 4.32 – 4.36 (m, 1H), 6.96 – 6.99 (m, 1H), 7.08 (dt, J = 7.2, 1.2 Hz, 1H), 7.12 – 7.17 (m, 3H), 7.25 – 7.38 (m, 4H); 13C NMR (75 MHz, CDCl3): δ 36.9, 40.6, 117.1, 124.6, 125.7, 127.5, 127.6, 128.3, 128.8, 129.1, 140.2, 151.7, 167.6; IR (KBr): 1767, 1497, 1454, 1418, 1335, 1277, 1202, 1188, 1134, 966, 895, 816, 760, and 708 cm-1; [α]D30.2 –41.1° (c 1.0, CHCl3); HPLC (Daicel Chiralcel OD-H, Hexane/i-PrOH = 95/5, flow rate = 1.0 mL/min) tR of (S)-4a: 13.8 min (0%); tR of (R)-4a: 15.2 min (>99%).
11. (R)-6-Methyl-4-phenylchroman-2-one (4b): m.p. 112 – 113 °C; 1H NMR (300 MHz, CDCl3): δ 2.25 (s, 3H), 2.98 (dd, J = 15.6, 6.3 Hz, 1H), 3.06 (dd, J = 15.6, 6.3 Hz, 1H), 4.27 – 4.31 (m, 1H), 6.78 (m, 1H), 7.02 (d, J = 8.4 Hz, 1H), 7.07 – 7.17 (m, 3H), 7.25 – 7.38 (m, 3H); 13C NMR (75 MHz, CDCl3): δ 20.7, 37.1, 40.7, 116.8, 125.3, 127.5, 127.6, 128.6, 129.1, 129.3, 134.3, 140.5, 149.6, 167.8; IR (KBr): 1760, 1610, 1587, 1487, 1450, 1339, 1337, 1221, 1205, 1136, 1111, 966, 908, 874, 767, 756, and 700 cm-1; [α]D30.7 = –3.6° (c 1.0, CHCl3); HPLC (Daicel Chiralcel OD-H, Hexane/i-PrOH = 90/10, flow rate = 1.0 mL/min) tR of (S)-4b: 12.2 min (0%); tR of (R)-4b: 14.0 min (>99%).
12. 7-Methoxy-4-phenylchroman-2-one (4c): m.p. 133 – 134 °C; 1H NMR (300 MHz, CDCl3): δ 2.98 (dd, J = 15.9, 7.8 Hz, 1H), 3.07 (dd, J = 15.9, 7.8 Hz, 1H), 3.80 (s, 3H), 4.26 – 4.31 (m, 1H), 6.63 (dd, J = 8.4, 2.7 Hz, 1H), 6.69 (d, J = 2.7 Hz, 1H), 6.85 – 6.88 (m, 1H), 7.13 – 7.17 (m, 2H), 7.25 – 7.37 (m, 3H); 13C NMR (75 MHz, CDCl3): δ 37.3, 40.1, 55.5, 102.5, 110.7, 117.6, 127.5, 127.6, 128.9, 129.1, 140.7, 152.4, 160.0, 167.6; IR (KBr): 1755, 1629, 1585, 1508, 1456, 1325, 1267, 1221, 1134, 1109, 1030, 972, 880, 833, 752, and 700 cm-1; [α]D31.3 –43.0° (c 1.0, CHCl3); HPLC (Daicel Chiralcel OD-H, Hexane/i-PrOH = 95/5, flow rate = 1.0 mL/min) tR of minor enantiomer of 4c: 17.3 min (0%); tR of major enantiomer of 4c: 21.5 min (>99%).
13. In ref. 8, plus sign of optical rotation of 4c had been assigned to be (R)-enantiomer. However, the determination had been performed by analogy with (R)-4b (in addition, the optical rotation of (R)-4b had shown minus sign in ref. 8 as well as our result). If absolute configuration of 4c is determined by analogy with (R)-4a or 4b, 4c in table 2 is (R)-enantiomer.