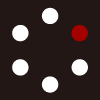
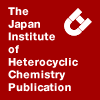
HETEROCYCLES
An International Journal for Reviews and Communications in Heterocyclic ChemistryWeb Edition ISSN: 1881-0942
Published online by The Japan Institute of Heterocyclic Chemistry
e-Journal
Full Text HTML
Received, 17th July, 2009, Accepted, 18th August, 2009, Published online, 20th August, 2009.
DOI: 10.3987/COM-09-S(S)59
■ Synthesis of Fluorinated 2,3-Disubstituted Benzofurans Potential β-Amyloid Aggregation Inhibitors
Marek Zaidlewicz,* Tomasz Kosmalski, Tathagata Sengupta, Kochupurackal P. Mohanakumar, and Krzysztof Staszak
Department of Chemistry, Nicolaus Copernicus University, 7 Gagarin Street, 87-100 Torun, Poland
Abstract
A convenient synthesis of 2-[4’-(3-diethylaminopropoxy)phenyl]-3-(2-fluoro¬benzoyl)benzofuran, 3-(4-fluorobenzoyl)- and 3-(4-trifluoromethylbenzoyl) derivatives, via the Suzuki cross-coupling reaction as the key transformation, is described.Aggregated β-amyloid peptides are components of the intracellular neurofibrillary tangles of senile plaques in Alzheimer’s disease brain.1-4 For symptomatic treatment acetylcholine esterase inhibitors, e.g. donepezil, rivastigmine, or galantamine, are used,5,6 and new compounds are under investigation,7 to increase acetylcholine levels in the brain thereby improving cholinergic neurotransmission.5,6 Among other approaches to Alzheimer’s disease therapy, inhibitors of β-amyloid aggregation are studied.8
Recently, benzofuran derivatives 1, 2, their 3-benzoyl and 3-tolyl derivatives were shown to be inhibitors of β-amyloid fibrils formation.9-11 The observed structure activity relationships prompted us to undertake the synthesis of 2-[4’-(3-diethylaminopropoxy)phenyl]-3-(2-fluorobenzoyl)benzofuran (3), 3-(4-fluorobenzoyl), and 3-(4-trifluoromethylbenzoyl) derivatives 4, 5 (Scheme 1), since the introduction of fluorine often exerts an effect on the physiological activity of compounds, exemplified by many fluorinated drugs.5
Although the compounds 3–5 could be prepared via earlier approaches to the synthesis of non-fluorinated compounds, involving classical non-catalytic transformations,9,12,13 we decided to introduce the aryl substituent in the 2-position of benzofuran by the Suzuki cross-coupling reaction. Thus, 2-benzofuranylboronic acid (6),14 was treated with 4-(3-diethylaminopropoxy)bromobenzene (7) in the presence of palladium acetate, potassium carbonate, in tetrahydrofuran–water, however, under these conditions the coupling product was not obtained. Attempted coupling of 7 with more reactive cyclic triolborates, prepared from 6 following the reported general procedure,15 was also unsuccessful.
Consequently, the synthesis was modified to prepare 2-(4-methoxyphenyl)benzofuran 8 by the cross-coupling reaction of 6 with 4-iodoanisole. Two reported reaction conditions,16,17 developed for the reaction of various aryl iodides and bromides with arylboronic acids, have been tested. The catalytic system, palladium acetate/DABCO, in the presence of potassium carbonate in acetone at 110 °C, produced the coupling product in low yield. Fortunately, under the second reaction conditions, palladium acetate, potassium carbonate in polyethylene glycol 4000–methanol,17 the reaction produced 8 in 94% yield (Scheme 2). Isolation of the product is simple and does not require chromatographic separation or purification. The reaction works also when water is used instead of methanol, although it is less convenient. Earlier, 8 was prepared by the Suzuki reaction of 6 with 4-bromoanisole in a microwave-assisted reaction, 90% yield,18 and also with tetrafluoroborate diazonium salt, generated from 4-bromoaniline, 61% yield.19
It should be noted that under conditions used for the synthesis of 8 no cross-coupling of 6 with 4-(3-diethylaminopropoxy)bromobenzene (7) was observed. Apparently, either lower reactivity of the aryl bromide moiety, or deactivation of the catalyst by the amino group prevents the reaction.
In the next step, the Friedel-Crafts acylation of 8 with 4-fluorobenzoyl chloride in the presence of tin(IV) chloride in dichlomethane produced the corresponding fluoroketone 9 in 85% yield. The methoxy group of 9 was cleaved with boron tribromide at room temperature, and the Williamson reaction of the deprotected phenol 10 with 3-chloro-N,N-diethylpropylamine produced 3, a viscous liquid, in 85% yield (Scheme 2). The 4-fluoro isomer 4 was prepared in the same way.
The synthesis can be modified, starting with phenol 11 and carrying the acylation reaction in the last step (Scheme 3). Thus, cleavage of the methoxy group of 8 gave phenol 11 in 90% overall yield from 2-benzofuranylboronic acid. An alternate synthesis from 2-(4-nitrophenyl)benzofuran by the reduction – diazotization – hydrolysis sequence afforded 11 in 62% yield.13
Both steps, the Williamson and Friedel-Crafts reactions, under the conditions indicated, gave lower yields as compared to the route shown on Scheme 2. Acylation of 12 in the presence of aluminum chloride (2.2 molar equivalents) requires a larger amount of Lewis acid, longer time, and higher temperature, as compared to the acylation of 8 in the presence of tin(IV) chloride. The final products 3 and 5, prepared according to Scheme 3, were purified by column chromatography on silica gel, eluent ethyl acetate-triethylamine 100:1.
In conclusion, the Suzuki cross-coupling reaction, providing a high yield access to the key intermediate 8 under mild conditions from readily available materials, makes the approach shown on Scheme 2 convenient and efficient. Hydrochlorides of aminoketones 3–5 were investigated for acetylcholinesterase inhibitory activity, using purified enzyme from bovine erythrocytes employing spectrophotometry,20 but found to be not effective in the range of 10-9–10-4 M. β-Amyloid aggregation inhibitory activity is currently under investigation.
EXPERIMENTAL
2-(4-Methoxyphenyl)benzofuran (8). 4-Iodoanisole (1.87 g, 8 mmol), potassium carbonate (1.84 g, 13.3 mmol), and 6 (1.63 g, 10 mmol) were added to a mixture of MeOH (20.00 g) and polyethylene glycol 4000 (20.00 g) at 50 °C under argon. After 10 min stirring, palladium acetate (10 mg) was added and the mixture was stirred for 1 h at this temperature. MeOH was removed on a rotary evaporator, water (100 mL) was added, precipitated solid was filtered off and dissolved in Et2O. The filtrate was extracted with Et2O (2x50 mL). The ethereal solutions were combined, washed with 3M aqueous NaOH (25 mL), saturated brine (20 mL), and dried with anhydrous magnesium sulfate. Solvent was removed and the product was crystallized from n-hexane, 1.69 g, 94%, mp 153–154 °C (Lit.,21 152–154 °C). 1H NMR (300 MHz, CDCl3), δ (ppm) 3.87 (s, 3H, CH3), 6.90 (d, J = 0.6 Hz, 1H, CH), 6.96–7.01 (AA’ spin system, 2H, CH), 7.21 (td, J = 7.2, 1.6 Hz, 1H, CH), 7.26 (td, J = 7.2, 1.8 Hz, 1H, CH), 7.51 (ddd, J = 7.2, 1.8, 0.6 Hz, 1H, CH), 7.56 (ddd, J = 7.2, 1.8, 0.6 Hz, 1H, CH), 7.78–7.83 (BB’ spin system, 2H, CH). 13C NMR (75 MHz, CDCl3), δ (ppm) 55.31 (CH3), 99.65 (CH), 110.96 (CH), 114.22 (2CH), 120.54 (CH), 122.80 (CH), 123.31 (C), 123.70 (CH), 126.39 (2CH), 129.47 (C), 154.64 (C), 156.03 (C), 159.95 (C).
2-[4’-(3-Diethylaminopropoxy)phenyl]-3-(2-fluorobenzoyl)benzofuran (3). 1H NMR (300 MHz, CDCl3) δ (ppm) 1.04 (t, J = 7.2 Hz, 6H, CH3), 1.91 (quintet, J = 6.3 Hz, 2H, CH2), 2.55 (q, J = 7.2, 4H, NCH2), 2.59 (t, J = 6.3 Hz, 2H, NCH2), 3.90 (t, J = 6.3 Hz, 2H, OCH2), 6.74–6.80 (AA’ spin system, 2H, CH), 6.88 (ddd, J = 10.2, 8.4, 0.9 Hz, 1H, CH), 7.09 (td, J = 7.5, 1.2 Hz, 1H, CH), 7.28 (td, J = 7.5, 1.2 Hz, 1H, CH), 7.31–7.40 (m, 2H, CH), 7.53–7.60 (m, 4H, CH), 7.74 (ddd, J = 7.2, 1.2, 0.9 Hz, 1H, CH). 13C NMR (75 MHz, CDCl3) δ (ppm) 11.81 (2CH3), 26.93 (CH2), 47.04 (2NCH2), 49.19 (NCH2), 66.38 (OCH2), 111.01 (CH), 114.09 (2CH), 116.10 (d, JCF = 21.8 Hz, 2CH), 116.28 (C), 121.46 (C), 121.53 (CH), 124.05 (CH), 124.07 (d, JCF = 4.5 Hz, CH), 125.05 (CH), 127.64 (C), 128.21 (d, JCF = 12.7 Hz, C), 130.63 (2CH), 130.82 (d, JCF = 2.20 Hz, CH), 133.35 (d, JCF = 8.6 Hz, CH), 153.65 (C), 160.36 (d, JCF = 254.4 Hz, C), 160.54 (C), 161.18 (d, JCF = 1.4 Hz, C), 188.31 (CO). IR: 1640 cm-1 (C=O); HRMS: C28H28FNO3 m/z [M+1]+ Calcd. 446.2126; Found 446.2124. Anal. Calcd for C28H28FNO3: C 75.48%; H 6.33%; N 3.14%. Found C 75.65%; H 6.38%; N 3.44%.
2-[4’-(3-Diethylaminopropoxy)phenyl]-3-(4-fluorobenzoyl)benzofuran (4). Hydrochloride (4·HCl), mp 149–152 °C. 1H NMR (300 MHz, CDCl3) δ (ppm) 1.39 (t, J = 7.2 Hz, 6H, CH3), 2.29-2.40 (m, 2H, CH2), 3.13 (q, J = 7.2, 4H, NCH2), 3.18 (t, J = 8.1 Hz, 2H, CH2), 4.05 (t, J = 5.7 Hz, 2H, CH2), 6.73-6.81 (AA’ spin system, 2H, CH), 6.93-7.03 (AA’ spin system, C6H4F, 2H, CH), 7.22 (td, J = 7.5, 0.9 Hz, 1H, CH), 7.32 (td, J = 8.1, 1.2 Hz, 1H, CH), 7.47 (dd, J = 7.5, 0.6 Hz, 1H, CH), 7.53 (dd, J = 8.1, 0.4 Hz, 1H, CH), 7.55-7.61 (BB’ spin system, 2H, CH), 7.78-7.86 (BB’ spin system, C6H4F, 2H, CH). 13C NMR (75 MHz, CDCl3) δ (ppm) 8.49 (2CH3), 23.59 (CH2), 46.62 (2CH2), 48.97 (CH2), 64.79 (CH2), 111.05 (CH), 114.22 (2CH), 114.75 (C), 115.50 (d, JCF = 21.7 Hz, 2CH), 121.08 (CH), 122.36 (C), 123.75 (CH), 125.09 (CH), 128.26 (C), 130.03 (2CH), 132.33 (d, JCF = 9.36 Hz, 2CH), 134.15 (d, JCF = 2.87 Hz, C), 153.51 (C), 157.60 (C), 159.32 (C), 165.57 (d, JCF = 254.95 Hz, C), 190.59 (CO). 4: Anal. Calcd for C28H28FNO3: C 75.48%; H 6.33%; N 3.14%. Found C 75.40%; H 6.30%; N 3.09%. IR: 1643 cm-1; HRMS: C28H28FNO3 m/z [M+1]+ Calcd. 446.2126; Found 446.2123.
2-[4’-(3-Diethylaminopropoxy)phenyl]-3-(4-trifluoromethylbenzoyl)benzofuran (5). 1H NMR (300 MHz, CDCl3) δ (ppm) 1.03 (t, J = 7.2 Hz, 6H, CH3), 1.93 (quintet, J = 6.6 Hz, 2H, CH2), 2.53 (q, J = 7.2, 4H, 2NCH2), 2.58 (t, J = 6.6 Hz, 2H, NCH2), 3.99 (t, J = 6.3 Hz, 2H, OCH2), 6.76–6.81 (AA’ spin system, 2H, CH), 7.28 (ddd, J = 7.5, 7.2, 1.5 Hz, 1H, CH), 7.37 (ddd, J = 8.1, 7.5, 1.5 Hz, 1H, CH), 7.51-7.58 (BB’ spin system, 2H, CH), 7.55-7.59 (m, 4H, CH), 7.61 (ddd, J = 7.2, 0.9, 0.6 Hz, 1H, CH), 7.87 (ddd, J = 8.1, 0.8, 0.6 Hz, 1H, CH). 13C NMR (75 MHz, CDCl3) δ (ppm) 11.72 (2CH3), 26.88 (CH2), 46.99 (2NCH2), 49.19 (NCH2), 66.45 (OCH2), 111.14 (CH), 114.42 (2CH), 114.46 (C), 121.36 (CH), 123.57 (q, JCF = 271.2 Hz, CF), 124.01 (CH), 125.23 (CH), 125.28 (q, JCF = 3.5 Hz, 2CH), 128.13 (C), 129.93 (2CH), 130.36 (2CH), 133.88 (q, JCF = 32.4 Hz, C), 140.58 (C), 141.05 (C), 153.71 (C), 159.68 (C), 160.70 (C), 191.09 (CO). Anal. Calcd for C29H28F3NO3: C 70.29%; H 5.69%; N 2.83%. Found C 70.17%; H 5.50%; N 3.06%. IR: 1645 cm-1 (C=O); HRMS: C29H28F3NO3 m/z [M+1]+ Calcd. 496.20941; Found 496.20857.
ACKNOWLEDGEMENTS
Financial support from the Ministry of Higher Education, Warsaw, grant PBZ-KBN 126/T09/2004 is acknowledged.
References
1. E. D. Robertson and L. Mucke, Science, 2006, 314, 781. CrossRef
2. K. Blennow, M. J. de Leon, and H. Zetterberg, Lancet, 2006, 368, 387. CrossRef
3. T. Luhrs, C. Ritter, M. Adrian, D. Riek-Loher, D. Bohrmann, H. Dobeli, D. Schubert, and R. Riek, Proc. Natl. Acad. Sci. U.S.A., 2005, 102, 17342. CrossRef
4. J. Hardy and D. J. Selcoe, Science, 2002, 297, 353. CrossRef
5. G. H. Cocolas and S. I. Cutler, in ‘Organic Medicinal and Pharmaceutical Chemistry,’ 11th ed., ed. by J. H. Block, J. M. Biele, Lippincott Williams & Wilkins, Philadelphia, 2004, 566; E. J. Corey, B. Czako, and L. Kürti, ‘Molecules and Medicine,’ J. Wiley, Hoboken, 2007, 225.
6. R. A. Hansen, G. Gartlehner, K. N. Lohr, and D. I. Kaufer, Drugs Aging, 2007, 24, 155; CrossRef E. I. Solntseva, J. V. Bukanova, E. V. Marchenko, A. V. Rossokhin, and N. G. Skrebitsky, Cell. Mol. Neurobiol., 2009, 29, 219; CrossRef H. Sugimoto, H. Ogura, Y. Arai, Y. Iimura, and Y. Yamanishi, Jpn. J. Pharmacol., 2002, 89, 7. CrossRef
7. F. Belluti, L. Piazzi, A. Bisi, S. Gobi, M. Bartolini, A. Cavalli, P. Valenti, and A. Rampa, Eur. J. Med. Chem., 2009, 44, 1341; CrossRef R. Sheng, Y. Xu, Ch. Hu, J. Zhang, X. Lin, J. Li, B. Yang, Q. He, and Y. Hu, Eur. J. Med. Chem., 2009, 44, 7. CrossRef
8. C. A. Ross and M. A. Poirier, Nat. Med., 2004, S10; CrossRef D. Shaw, J. Best, K. Dinnell, A. Nadin, M. Sherman, C. Pattison, J. Peachey, M. Reilly, B. Williams, J. Wrigley, and T. Harrison, Bioorg. Med. Chem. Lett., 2006, 16, 3073. CrossRef
9. L. J. Twyman and D. Allsop, Tetrahedron Lett., 1999, 40, 9383. CrossRef
10. D. Allsop, G. Gibson, I. K. Martin, S. Moore, S. Turnbull, and L. J. Twyman, Bioorg. Med. Chem. Lett., 2001, 11, 255. CrossRef
11. S. Rizzo, C. Riviere, L. Piazzi, A. Bisi, S. Gobi, M. Bartolini, V. Andrisano, F. Morroni, A. Tarazzi, J.-P. Monti, and A. Rampa, J. Med. Chem., 2008, 51, 2883. CrossRef
12. L. M. Brenner and Ch. K. Brush, US Pat. 4,001,426 (1977). Chem. Abstr., 1977, 87, 39262h.
13. O. Dann, J. Ruff, and H. Griessmeier, Liebigs Ann. Chem., 1984, 409.
14. J. Yates and R. S. Airs, GB Pat. 814647, 1959. Chem. Abstr., 1960, 54, 8851h.
15. Y. Yamamoto, M. Takizawa, X.-Q. Yu, and N. Miyaura, Angew. Chem. Int. Ed., 2008, 47, 928. CrossRef
16. J.-H. Li and W.-J. Liu, Org. Lett., 2004, 6, 2809. CrossRef
17. L. Liu, Y. Zhang, and Y. Wang, J. Org. Chem., 2005, 70, 6122. CrossRef
18. I. R. Baxendale, Ch. M. Gryffiths-Jones, S. V. Ley, and G. K. Tranmer, Chem. Eur. J., 2006, 12, 4407. CrossRef
19. R. H. Taylor and F.-X. Felpin, Org. Lett., 2007, 9, 2911. CrossRef
20. G. L. Ellman, D. Courtney, V. Andres, and R. M. Featherstone, Biochem. Pharmacol., 1961, 7, 88. CrossRef
21. M. Csekei, Z. Novak, and A. Kotschy, Tetrahedron, 2008, 64, 8992. CrossRef