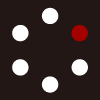
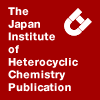
HETEROCYCLES
An International Journal for Reviews and Communications in Heterocyclic ChemistryWeb Edition ISSN: 1881-0942
Published online by The Japan Institute of Heterocyclic Chemistry
e-Journal
Full Text HTML
Received, 28th July, 2009, Accepted, 4th September, 2009, Published online, 8th September, 2009.
DOI: 10.3987/COM-09-S(S)80
■ Boron Trichloride Mediated Alkyne-Aldehyde Coupling Reactions
Min-Liang Yao, Michael P. Quinn, and George W. Kabalka*
Departments of Chemistry and Radiology, The University of Tennessee, Knoxville, Tennessee 37996-1600, U.S.A.
Abstract
The boron trichloride mediated reaction of aryl aldehydes and alkynes can be used to selectively generate either (Z,Z)- or (Z,E)-1,5-dichloro-1,4-dienes as major products, depending on the reaction conditions employed. To investigate the mechanism of this important carbon-carbon bond forming reaction, the boron trichloride mediated reaction of phenylacetylene with p-bromobenzaldehyde was chosen as the model system. Interestingly, when the coupling reactions are carried out below –60 °C, (E,E)-1,5-dichloro-1,4-dienes are also formed. The ratios of (Z,Z)-, (Z,E)-, and (E,E)-1,5-dichloro-1,4-dienes were correlated to the reaction conditions using gas chromatography-mass spectrometry. The results of the investigation can be used to explain why the ratio of (Z,E)-, (Z,Z)-, and (E,E)-1,5-dichloro-1,4-pentadiene products change very dramatically when changes are made in either the sequence of addition of the reagents or the reaction temperature.INTRODUCTION
Our research group has focused on the chemistry of organoboron halide derivatives for many years and resulted in the first report of a Grignard-like alkylation of aryl aldehydes using either dialkylboron chlorides1 or alkylboron dichlorides.2 In a continuation of these studies, we investigated the feasibility of expanding the reaction to the addition of vinylboron dichloride reagents to aryl aldehydes.3 Surprisingly, the reaction afforded (Z,Z)-1,5-dichloro-1,4-pentadienes as major products instead of the expected Grignard-like addition products, if preformed (Z)-vinylphenylboron dichloride reagents3a were used. Since it has been reported that boron trichloride readily adds to terminal alkynes to generate (Z)-vinylboron dichlorides,4 we decided to explore a one-pot reaction employing boron trichloride, phenylacetylene and benzaldehyde at 0 oC. We found that a diene product formed but, unexpectedly, (Z,E)-1,5-dichloro-1,4-pentadiene was isolated as the major product instead of the expected (Z,Z)-1,5-dichloro-1,4-pentadiene. (None of the (E,E)-1,5-dichloro-1,4-pentadiene isomer was detected.)
Several novel reactions were then designed to probe the reaction mechanism.5 During these studies, we discovered new reactions involving an unprecedented C-O bond cleavage in the R1R2CH-O-BXnR3-n intermediates; these include a transition-metal-free, formal Suzuki-coupling of alkoxides with vinylboron dichlorides,5b the boron trichloride mediated coupling of alkoxides with allylsilane,5e and the boron trihalide mediated haloallylation of aryl aldehydes using allylmetal compounds.5g In addition, we found that, at low temperature, the reaction of boron trichloride with two equivalents of terminal alkyne yielded only the vinylboron dichloride product and not the reported divinylboron chloride.3b
Although the methodology required for the stereoselective, boron trihalide mediated alkyne-aldehyde coupling reactions has been reported,3 the dramatic influence on the stereochemistry of the products due to the order of reactant addition and reaction temperature had not been investigated in details. To fully explore these parameters, we chose to study the reaction of phenylacetylene with p-bromobenzaldehyde as a model system.
RESULTS AND DISCUSSIONS
(Z,Z)-1,5-Dichloro-1,4-pentadiene (1) was synthesized according to reported procedure.3b In the reaction of (Z,Z)-di(chlorovinyl)boron chloride with p-bromobenzaldehyde (Scheme 1), the halovinyl group migrates from boron to carbon with retention of stereochemistry.3b,5b,5c Racemization occurred in the coupling reaction of chiral benzyloxide and vinylboron dihalide suggesting that a cationic mechanism is involved in this migration.5c
Alternatively, compound 1 can also be prepared by the reaction of p-bromobenzaldehyde with two equivalents of preformed (Z)-chlorovinylboron dichloride (Scheme 2). The Grignard-like addition of the (Z)-vinylboron dichloride to the aldehyde followed by a carbon-oxygen bond cleavage affords a carbocation intermediate. Then the cation adds to a second equivalent of the (Z)-vinylboron dichloride to generate the observed Z,Z-diene product. It has been demonstrated that electrophiles can add to vinylboron reagents stereoselectively with retention of configuration of the vinyl group.6
(Z,E)-1,5-Dichloro-1,4-pentadiene (2) was prepared by adding boron trichloride to a mixture of the p-bromobenzaldehyde and phenylacetylene at 0 oC (Scheme 3). Reaction of boron trichloride with the alkyne first generates the (Z)-chlorovinylboron dichloride. Addition of (Z)-vinylboron dichloride to the aldehyde, followed by a carbon-oxygen bond cleavage affords a carbocation intermediate which then adds to the second alkyne to generate the more thermodynamically stable (Z,E)-diene 2 as the major product. In this case, boron trichloride actually functions as a reactant. The reaction outlined in Scheme 3 presumably competes with one shown in Scheme 2. This assumption is strongly supported by two observations. The quantity of (Z,Z)-diene 1 increases when the aldehyde is added to a mixture of phenylacetylene and boron trichloride, a sequence which favors the formation of the vinylboron dichloride (Scheme 2). However, adding boron trichloride to a mixture of aldehyde and excess phenylacetylene (3.0 equivalents) at 0 oC increases the chance of quenching carbocation by acetylene and results in a higher ratio of 2 to 1. Since (Z,Z)-di(chlorovinyl)boron chloride only forms at elevated temperature (refluxing dichloromethane),3b the reaction pathway shown in Scheme 1 should not be responsible for the formation of 1 in this investigation.
After establishing the analytical GC-MS conditions7 for separating stereoisomers 1 and 2, we examined the addition of boron trichloride to a mixture of two equivalents of phenylacetylene and one equivalent of p-bromobenzaldehyde at various temperature (Table 1). The reaction mixtures were subjected to GC-MS analyses after a simple workup.8 As illustrated in Table 1, (Z,E)-1,5-dichloro-1,4-pentadiene product 2 was always the major product, indicating that the reaction pathway shown in Scheme 3 played a predominant role. As the temperature was decreased from 0 oC to -42 oC, the regioselectivity [(Z,E)- /(Z,Z)- isomer] increased due to the thermodynamic stability of the (Z,E)-pentadiene, 2. The reaction pathway outlined in Scheme 2 presumably is also involved but it would be expected to be minimal at temperatures approaching –60 oC, because the haloboration reaction generating (Z)-chlorovinylboron dichloride would be quite slow at such a low temperature; as a consequence, the concentration of (Z)-chlorovinylboron dichloride should be much lower than that of phenylacetylene. Interestingly, reactions run at temperatures below –60 oC also produce (E,E)-1,5-dichloro-1,4-pentadiene, 3. At these low temperatures, boron trichloride doesn’t participate in haloboration very effectively but still acts as a Lewis acid to initiate the competing reaction that generates (E,E)-1,5-dichloro-1,4-pentadiene 3 (Scheme 4). Addition of the alkyne to the boron trichloride activated carbonyl group, followed by C-O bond cleavage, forms a carbocation bearing a thermodynamically stable (E)-vinyl moiety. Addition of the cation to another equivalent of phenylacetylene then generates the more thermodynamically stable (E,E)-diene product 3.
Compound 3 was isolated by column chromatography. The 1H spectrum of the compound exhibited only one set of vinyl resonances which supports the formation of the symmetrical (E,E)-1,5-dichloride-1,4-pentadiene isomer.9 The structure was further confirmed by X-ray crystallography (Figure 1).10
The specific proton chemical shifts and coupling constants for vinyl and benzylic protons in the diene products, as well as the carbon-13 chemical shifts of the benzylic carbons, for the characterization of (Z,Z)-, (Z,E)-, (E,E)-isomers are tabulated in Table 2.
As summarized in Table 2, both the vinyl and benzylic protons in the (E,E)-diene are upfield when compared to those in (Z,Z)-diene. Surprisingly, the chemical shift of the benzylic proton in (E,E)-diene and (Z,Z)-diene approach 1 ppm.
CONCLUSION
The reaction mechanism of phenylacetylene, p-bromobenzaldehyde, and boron trichloride have been investigated and analyzed. Utilizing GC-MS data, the ratios of (Z,Z)-, (Z,E)-, and (E,E)-1,5-dichloro-1,4-diene isomers in the crude reaction mixtures obtained under varying reaction conditions were determined. The investigation reveals that, at relatively higher temperature (> - 42 oC), the addition of boron trichloride to phenylacetylene occurs rapidly and boron trichloride functions as a reactant to generate the key intermediate (Z)-chlorovinylboron dichloride. While, at relative lower temperature, the addition of boron trichloride to phenylacetylene becomes sluggish and the unreacted boron trichloride acts as a Lewis acid and leads to formation of the (E,E)-diene.
ACKNOWLEDGMENT
Acknowledgment is made to the Donors of the Petroleum Research Fund for support of this research. We also wish to thank the U.S. Department of Energy and the Robert H. Cole Foundation for partial support of this research.
References
1. G. W. Kabalka, Z. Wu, S. Trotman, and X. Gao, Org. Lett., 2000, 2, 255. CrossRef
2. G. W. Kabalka, Z. Wu, and Y. Ju, Tetrahedron, 2001, 57, 1663. CrossRef
3. (a) G. W. Kabalka, Z. Wu, and Y. Ju, Org. Lett., 2002, 4, 1491; CrossRef (b) G. W. Kabalka, M.-L. Yao, S. Borella, Z.-Z. Wu, Y.-H. Ju, and T. Quick, J. Org. Chem., 2008, 73, 2668. CrossRef
4. M. F. Lappert and B. Prokai, J. Organometal. Chem., 1964, 1, 384. CrossRef
5. (a) G. W. Kabalka, Z. Wu, and Y. Ju, Org. Lett., 2004, 6, 3929; CrossRef (b) G. W. Kabalka, M.-L. Yao, S. Borella, and Z.-Z. Wu, Chem. Commun., 2005, 2492; CrossRef (c) G. W. Kabalka, M.-L. Yao, S. Borella, and Z.-Z. Wu, Org. Lett., 2005, 7, 2865; CrossRef (d) G. W. Kabalka, M.-L. Yao, and S. Borella, Org. Lett., 2006, 8, 879; CrossRef (e) G. W. Kabalka, M.-L. Yao, and S. Borella, J. Am. Chem. Soc., 2006, 128, 11320; CrossRef (f) G. W. Kabalka, M.-L. Yao, S. Borella, and L. K. Goins, Organometallics, 2007, 26, 4112; CrossRef (g) M.-L. Yao, S. Borella, T. Quick, and G. W. Kabalka, J. Chem. Soc. Dalton, 2008, 776; CrossRef (h) M.-L. Yao, M. S. Reddy, W.-B. Zeng, K. Hall, I. Walfish, and G. W. Kabalka, J. Org. Chem., 2009, 74, 1385; CrossRef (i) M.-L. Yao, T. Quick, Z.-Z. Wu, M. P. Quinn, and G. W. Kabalka, Org. Lett., 2009, 11, 2647. CrossRef
6. (a) S. Hara, H. Dojo, S. Takinami, and A. Suzuki, Tetrahedron Lett., 1983, 24, 731; CrossRef (b) S. K. Stewart and A. Whiting, Tetrahedron Lett., 1995, 36, 3925; CrossRef (c) A. P. Lightfoot, G. Maw, C. Thirsk, S. J. R. Twiddle, and A. Whiting, Tetrahedron Lett., 2003, 44, 7645; CrossRef (d) G. W. Kabalka and A. R. Mereddy, Organometallics, 2004, 23, 4519; CrossRef (e) A. S. Batsanov, J. P. Knowles, and A. Whiting, J. Org. Chem., 2007, 72, 2525. CrossRef
7. GC-MS studies were run on Hewlett packard: HP 6890 series GC System with 5973 Mass Selective Detector; Column: Agilent 19091S-433E, 30.0mm x 0.25mm x 0.25μm; Gas (He) flow rate: 0.8 mL/min; Initial temperature; 50 oC (hold 1 min); Ramp temperature rate: 7 °C/min to maximum 280 °C.
8. Phenylacetylene (204 mg, 2.0 mmol) and p-bromobenzaldehyde (185 mg, 1.0 mmol) and were placed in a dry argon-flushed, 50 mL round-bottomed flask equipped with a stirring bar and dissolved in 15 mL dry CH2Cl2. The solution was cooled to desired temperature, and boron trichloride (1.1 mmol, 1.1 mL of a 1.0 M CH2Cl2 solution) was added via a syringe. The solution was allowed to stir for 1 h at that temperature. The resulting mixture was hydrolyzed with water (20 mL) and extracted with hexanes (2 x 30 mL). The organic layer was separated and passed through a short silicon gel column to remove the trace of water and acidic by-product.
9. Spectral data for (E,E)-1,5-dichloro-1,4-diene (3): 1H NMR (250 MHz, CDCl3): σ 7.47-7.01 (m, 14 H), 6.05 (d, 2 H, J = 10.7 Hz), 4.34 (t, 1 H, J = 10.7 Hz). 13C NMR: σ 140.60, 136.19, 132.89, 131.98, 128.84, 128.64, 128.22, 128.16, 121.01, 44.78.
10. Crystal structure analysis for (E,E)-diene 3: (C23H17BrCl2, Mr=444.18), monoclinic, space group P2(1)/n, a=8.730(2) Å, b= 13.248(3) Å, c=16.912(4) Å, α=90.00, β=95.957(4), γ=90.00, V=1945.4(8) Å3, Z=4, T=173(2), 17368 collected, 3899 crystallographic independent and 3442 reflexes mit I>2s(I), MoKa radiation, λ=0.71073 Å, θmax=26.20, R[I>2s(I)]: R1=0.0375, wR2= 0.0963, wR2=0.1008 (all data).