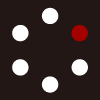
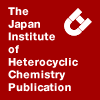
HETEROCYCLES
An International Journal for Reviews and Communications in Heterocyclic ChemistryWeb Edition ISSN: 1881-0942
Published online by The Japan Institute of Heterocyclic Chemistry
e-Journal
Full Text HTML
Received, 16th June, 2009, Accepted, 10th August, 2009, Published online, 11th August, 2009.
DOI: 10.3987/COM-09-S(S)37
■ Selective Triisopropylsilylation of α-Amino Acids: Protection without Racemization
Buddy Soto-Cairoli, Iveliz Kock, Jorge Justo de Pomar, Guang Yang, José M. Guzmán, Javier R. González, Augie Antomattei, and John A. Soderquist*
Department of Chemistry, University of Puerto Rico, San Juan, PR 00931-3346, U.S.A.
Abstract
The mono-, di- and trisilylation of α-amino acids with TIPSOTf is described. Treatment of the α-amino acids, proline and tryptophan with TIPSOTf in MeCN provides a quantitative yield of the corresponding ammonium salts of the O-TIPS esters (4). Employing two (2) equivalents of TIPSOTf/EDIPA produces N,O-bis-TIPS amino acid derivatives (2) without racemization as stable compounds (73–87%, 13 examples). Three (3) equivalents of this silylation mixture produces the corresponding tris-TIPS derivatives 6 (62–88%, 6 examples) from amino acids which contain protic functionality in their side chain. While this trisilylation procedure is ineffective for tryptophan, the N,O-bis-TIPS derivative 2m is easily prepared through this method, and the third silylation is achieved without racemization employing t-BuLi/R3SiX producing N1-SiR3-N,O-bis-TIPS tryptophans (51–97%, 4 examples).INTRODUCTION
Essential building blocks for the proteins of life, α-amino acids are of great interest in biochemistry, food science and medicinal chemistry.1 For applications to synthetic chemistry, their use can be limited by low solubility in organic solvents, a consequence of their zwitterionic nature.2 This issue can be resolved by derivatization of either the amine or carboxylate group. The amine functionality is usually converted to an N-alkoxycarbonyl derivative3 and the carboxylate moiety to an alkyl ester.4 In principle, silylation of these groups would be an ideal way to both solubilize amino acids and protect these functionalities by eliminating both the zwitterionic structure as well as intermolecular hydrogen bonding at the same time. Racemization should also be dramatically reduced compared to their carbamate counterparts. Trimethylsilyl (TMS) esters have been used as temporary protection for the carboxyl group because they are easily cleaved but their hydrolytic instability make them unsuitable for storage, and they are only prepared in situ.4,5 Persilylation of α-amino acids has been similarly used to enhance their volatility for their analysis by GC,6 to study their fragmentation behavior in the mass spectrometer,7 and in inverse solid-phase peptide synthesis.8
We became interested in the use of the triisopropylsilyl (TIPS) group as a protecting group in α-amino acid chemistry because it was known to not only retard reactions at the silicon center, but also, at adjacent centers.9 We developed an efficient protocol for the synthesis of representatives examples of stable and optically pure N,O-bis-TIPS-α-amino acid esters (2) from their amino acid precursors. Their borane-mediated reduction to enantiomerically pure N-TIPS- amino aldehydes (4) was accomplished through the hydrolysis of the intermediate oxazaborolidines 3.10 These silylated derivatives proved to be remarkably stable and easy to handle making them particularly well-suited for further conversions. Thus, through Wittig chemistry, we demonstrated their conversion to α,β-unsaturated-γ-amino esters 6 (Scheme 1). The derived Mosher amides were rigorously analyzed by NMR comparing these products to the diastereomeric mixtures obtained from racemic amino acids determining that ≤1% racemization had occurred in this overall process for the eight (8) representative examples chosen.10 Therefore, during the silylation, reduction, hydrolysis, olefination, desilylation and acylation procedures, no detectable racemization occurs! Herein, we present an extension of the TIPS silylation chemistry to more functionalized amino acids as well as to mono- and tri-TIPS derivatives.
RESULTS AND DISCUSSION
In this and our previous studies, our first challenge was to develop a workable procedure for solubilizing the amino acids (1) sufficiently to permit silylation to occur. We investigated known TIPS silylation procedures for carboxylic acids finding them to be ineffective for 1.9a Requiring the extremely reactive TIPSOTf to observe any silylation, we found non-polar solvents and most polar solvents to be unsatisfactory. We were encouraged by our initial findings with THF at reflux temperature, but faced the challenge of avoiding its polymerization.11 Fortunately, this could be achieved through the dropwise addition of TIPSOTf to the mixture of 1 and amine base. Our initial studies utilized DBU which was quite effective for the preparation of 2, but this procedure resulted in extensive racemization in some cases (e.g., L-serine and L-phenylglycine). As noted above, these problems were eliminated employing the highly hindered ethyldiisopropylamine (EDIPA, Hünig’s base) which produced the desired N,O-bis-TIPS amino acids (2) in high yields as pure, stable compounds without significant racemization (≤1%) (eqn. 1). This procedure has now been applied to thirteen (13) examples which are given in Table 1. In the case of proline, we also demonstrated that the corresponding N,O-bis-TBDMS derivative (2k’) was readily prepared in 76% yield employing TBDMSOTf instead of TIPSOTf in this protocol.
The silylation procedure was particularly effective; producing 2 as liquid compounds except in the case of the indolyl derivative 2m which was obtained as a crystalline solid from dry hexane through a slow
evaporation technique. We were curious as to the reason this compound was crystalline. Fortunately, the crystals proved to be of sufficient quality for X-ray analysis and the solid state structure of 2m was determined and is depicted in Figure 1. The molecule forms a dimer through H-bonding between the indole’s NH and the TIPS ester carbonyl oxygen. These interactions are possible due to the planarity of the indolyl groups which fit between the TIPS groups on each monomeric component. Large Si-N-C (~129°) and Si-O-C (126, 129°) are also observed due to the influence of the steric bulk of the TIPS groups.
We also examined the solution (CCl4) and solid state (KBr pellet) IR spectra of 2m to note differences which may be attributable to the presence of this H-bonding. Historically, this technique has played a major role in related phenomena in amino acid chemistry.13–15 Thus, the solution N–H stretching bands (3488, 3390 cm-1) coalesce to a single broadened absorbance at 3370 cm-1 in the solid state. Also, the solution υC=O (1716 cm-1) is lowered to1701 cm-1 in the solid state. These changes are in agreement with the dimeric structure of 2m in the solid state compared to its being monomeric in solution.
It was of interest to better understand the nature of the silylation process which ultimately produces 2. Toward this end, we returned to an examination of the TIPSOTf/DBU system in CDCl3 by 13C NMR. We observed an exothermic reaction between TIPSOTf and DBU forming the corresponding N-TIPS amidinium triflate (7) as evidenced by a downfield shift of C-7 from δ 160.5 in DBU itself, to δ 171.8 in the salt (δ 166.0 in DBU-HOTf).12 Moreover, the signals observed for the TIPS group in this DBU salt (δ 13.7, 20.9) are significantly shifted downfield from those in TIPSOTf (δ 11.9, 18.0). We isolated 3 in quantitative yield from a similar reaction in THF (3 h, 25 °C) as an air-sensitive solid. However, no comparable reaction is observed between EDIPA and TIPSOTf in refluxing THF even after 24 h. This suggested that with the EDIPA process, the amino acids were reacting directly with TIPSOTf at the solid-liquid interface. Thus, we added one equivalent of TIPSOTf to a suspension of phenylalanine in hot THF and found that the triflic acid salt of its O-TIPS ester is produced along with a large amount of poly-THF. This process appeared promising if the use of THF could be avoided. Fortunately, the reaction proceeds cleanly in dry acetonitrile at reflux temperature and two of these monotriisopropylsilyl esters 8 were prepared as their triflic acid salts in quantitative yields in 4 h (Scheme 2). While very sensitive to atmospheric moisture, these salts are stable for several weeks when stored under N2 at –20 oC. It is significant that the extent of silylation with TIPSOTf apparently could be so conveniently controlled through the proper choice of stoichiometry and reaction conditions.
We chose to extend the triisopropylsilylation methodology to α-amino acids which contain additional protic functionality in the side chain. Trisilylation was accomplished to provide 10 in excellent yields for a variety of amino acids (eqn. 2, Table 2). These silylated derivatives 10 proved to be very stable, further illustrating the versatility and value of this new TIPS silylation methodology.
The trisilylation of tryptophan proved to be more challenging. Fortunately, this was achieved through the further silylation of 2m with its deprotonation employing t-BuLi (THF, -78 oC) followed by treatment with R3SiX. This operation produces an intermediate reddish solution of the indolyllithium amide which is smoothly silylated (eqn. 3). Thus, by varying the silylating agent, N1-SiR3 derivatives 11 can be prepared including the tris-TIPS derivative 7c (Table 3). As expected, the trisilylated derivatives 11 are liquids, a consequence of the removal of the hydrogen-bonding which led to the dimeric form of 2m. It should also be noted that the TIPS-protected ester and amine functionalities did not undergo a discernable reaction with t-BuLi suggesting that the TIPS group offers wide-ranging protection for amino acids. Thus, when 2m is deprotonated with t-BuLi as above and MeOH (1 equiv) added followed by TMSCl (1 equiv), re-isolation of 2m (94%) reveals that the optical rotation of 2m is unchanged, demonstrating that neither the alkyllithium nor the intermediate indolyllithium racemizes the TIPS-protected amino ester 2m!
We chose to examine this reactivity further. Thus, isoleucine 2j was treated with n-BuLi at -78 °C, and the mixture was allowed to slowly warm to -32 °C to lithiate 2j (Scheme 3). Silylation with TMSCl at -78 °C results in 92% conversion (GC) of 2j to a new compound 12 whose MS and IR are consistent with N-trimethylsilylation (MS m/z (relative intensity) 517 (M+, 15), 502 (M–Me+,15), 474 (M–(i-Pr)+, 100); IR (KCl) 1734 cm-1). Treatment of the reaction mixture with aqueous NH4Cl (3 h, 25 °C) gave after workup, an 80% isolated yield of recovered 2j (99% GC purity) which was found to have identical spectroscopic properties to the original 2j as well as the same optical rotation! Again, the TIPS groups in 2 evidently prevent addition to the ester or deprotonation of the α-carbon to form the corresponding enolates.
We also chose to examine the reaction of an O-TIPS ester which lacked the NHTIPS substitution with alkyllithium reagents to see whether or not they would add to the ester. Toward this end, we prepared triisopropylsilyl β-methylcrotonate (13) and treated it at –78 °C in THF with n-BuLi. After three hours, silylation of the dienoate with TIPSCl gave the corresponding disilylketene acetal 14 in 73% yield (eqn. 4).
This experiment clearly demonstrates that, if any ester enolates were formed from 2, they would be silylated to produce observable side products. Moreover, one TIPS group is enough to prevent the addition of unhindered organolithiums (i.e., n-BuLi) from adding to the carboxy group to ultimately produce the 3°-carbinols which are observed from standard esters. The two TIPS groups in 2 prevent their addition to the carboxy group as well as enolate formation which would lead to racemization. These organolithiums can be used with impunity to deprotonate other positions for additional silylations.
This unusual reactivity of 2 with organolithiums led us to generate MM structures for both 2m and 2j (Spartan 06) to better understand the role of the TIPS groups in limiting access to the α–proton in these derivatives. These structures are illustrated in Figure 2. In 2m, the indolic H is clearly accessible (C) with both A and B hydrogens being far less available for reaction with t-BuLi. From a pKa standpoint, B is far more acidic than either A or C, but it is deeply buried in an ionophobic region which sterically blocks access to the base. With the less hindered N-H site (C) found in 2m not present in 2j, the base has no choice but to react with the NHTIPS moiety (proton A) which has both a carboxy oxygen and amino nitrogen to help coordinate the base during deprotonation. Once again, access to the B proton is denied by the TIPS groups and the optical purity of 2 is preserved.
CONCLUSIONS
In this study, a procedure for the mono-, di-, and trisilylation of α-amino acids was reported. Because of the large steric bulk of the triisopropylsilyl (TIPS) group, these silyl derivatives are stable and can be handled under a dry atmosphere. The monosilylation of proline and tryptophan is achieved with 1 equiv of TIPSOTf in MeCN to produce the corresponding O-TIPS esters (8) quantitatively. The N,O-bis-TIPS amino acids 2 are produced (72–87%, 13 examples) using the TIPSOTf/EDIPA combination in refluxing THF. With protic side-chain functionality, this methodology can be used to produce the tri-TIPS derivatives 10 (62–88%, 6 examples). In the case of tryptophan, disilylation occurs under the normal TIPSOTf/EDIPA conditions to provide 2m which forms a crystalline dimer whose X-ray structure was determined. Further silylation occurs through t-BuLi deprotonation of 2m followed by silylation with TMSCl, TESCl, TIPSOTf or TBDMSOTf to provide the corresponding N1-SiR3-N,O-bis-TIPS-tryptophans 11 (51–97%) without significant racemization. The TIPS groups in 2 offer protection from organolithiums against either their addition to the carboxy group or α-deprotonation leading to enolate formation.
EXPERIMENTAL
General information.
All experiments were carried out in pre-dried glassware (12 h, 150 oC) under nitrogen atmosphere. Standard handling techniques for air-sensitive compounds were also employed for all the operations. Nuclear magnetic resonance (NMR) spectra were obtained using General Electric DPX-300 and DRX-500 spectrometers. 1H (300 MHz), 13C (75 MHz) and 11B (96.5 MHz) NMR were recorded in CDCl3 (δ 7.26 and 77.0 respectively) and of C6D6 (δ 7.15 AND 128.0) as the internal standard. Infrared spectra were recorded on a Nicolet 740 GC FT-IR, a Perkin-Elmer 282 or a Nicolet series 6000 FT-IR spectrophotometer. Mass spectral data were obtained with a Hewlett-Packard 5995 A GC/MS spectrometer (70 eV), Fisons VG Autospect. High-resolution mass spectral data were obtained with a Micromass VG AutoSpec magnetic sector mass spectrometer (70 eV). Optical rotations were measured employing a Perkin-Elmer 243B polarimeter.
General procedure for the silylation of α-amino acids 1.
To a refluxing suspension of the amino acid (20.0 mmol) in dry THF (80 mL) under N2 atmosphere, EDIPA (40.0 mmol, 6.97 mL) was added. TIPSOTf (40.0 mmol, 10.75 mL) was added dropwise by syringe pump (3.00 mL/h, d = 20 mm), avoiding overheating. The solid amino acid gradually entered into solution. After the addition was complete, heating was maintained for 4 h. The reaction was cooled to room temperature under positive N2 pressure and THF was removed under vacuum. The remaining oil was diluted in dry hexane (100 mL) and stirred for a few minutes. The phases were allowed to partition and the upper phase was filtered via cannula through neutral alumina (Brockmann, activity [I]) into another flask. The ethyldiisopropylammonium salt phase was washed with hexane (2 x 50 mL) and filtered. The organic extracts were combined and concentrated. TIPSOH was slowly distilled (bp 40-42 °C, 0.4 torr) leaving the pure 2 which are air and moisture sensitive oils. However, they can be stored in dry hexane at -20 ºC under an inert atmosphere for several weeks. Compounds 2a-h were already published.10
(+)-N,O-bis-(Triisopropylsilyl)-(L)-valine (2i). 85% (12.8 g, 29.75 mmol) from (S)-valine (35.0 mmol, 4.1 g). 1H NMR (300 MHz, CDCl3) δ 0.93 (d, J = 7.0 Hz, 3H), 0.97 (d, J = 6.8 Hz, 3H), 1.03 (bs, 21H), 1.08 (bs, 18H), 1.22-1.35 (m, 4H), 1.90-1.97 (m, 1H), 3.40 (dd, J = 4.2, 12.7 Hz, 1H). 13C NMR (75 MHz, CDCl3) δ 12.0, 12.1, 12.5, 17.8, 18.0, 18.3, 18.4, 18.6, 34.3, 61.7, 175.3. IR (neat, NaCl) 3380, 2960, 2868, 1721, 886 cm-1. HRMS (EI) Exact mass calc. for C23H51NO2Si2 429.3458; Found (M-H) 428.3454. Anal. Calcd for C23H51NO2Si2: C 64.27%; H 11.96%. Found C 64.24%; H 12.04%. [α]25D + 6.8 (c 25.5, CCl4).
(+)-N, O-bis-(Triisopropylsilyl)-(L)-isoleucine (2j). 87% (12.9 g, 29.2 mmol) from (L)-isoleucine (33.5 mmol, 4.4 g). 1H NMR (300 MHz, CDCl3) δ 0.93 (t, J = 6.9 Hz, 3H), 1.05 (d, J = 6.7 Hz, 18H), 1.25 (d, J = 7.6 Hz, 3H), 1.29 (septet, J = 7.5, 3H), 1.48 (broad m, 3H), 1.57 (broad m, 2H), 1.60 (broad m, 1H), 3.39 (d, J = 4.2 Hz, 1H), 3.52 (dd, J = 12.3, 3.9 Hz, 1H). 13C NMR (75 MHz, CDCl3) δ 12.0, 12.3, 14.9, 17.7, 17.8, 17.9, 25.7, 42.2, 60.2, 175.1. IR (neat, NaCl) 3390, 2960, 2868, 1710, 1465 cm-1. HRMS (EI) Exact mass calc. for C21H46NO2Si2 400.3067; Found (M-C3H7) 400.3055. Anal. Calcd for C23H51NO2Si2: C 64.92%; H 12.05%. Found C 65.14%; H 12.10%. [α]25D + 18.1 (c 23.3, CCl4).
(-)-N,O-bis-(Triisopropylsilyl)-(L)-proline (2k). 86% (4.1 g, 9.6 mmol) from (S)-proline (11.2 mmol, 1.3 g). 1H NMR (300 MHz, C6D6) δ 1.05 (m, 39H), 1.29 (m, 3H), 1.42 – 1.99 (m, 4H), 3.07 (ddd, J = 9.4, 8.1, 4.9 Hz, 1H), 3.31 (ddd, J = 9.4, 8.1, 6.3 Hz, 1H), 4.11 (dd, J = 8.2, 2.5 Hz, 1H). 13C NMR (75 MHz, C6D6) δ 12.4, 12.9, 18.1, 18.8, 25.1, 32.1, 48.2, 62.5, 175.9. IR (neat, NaCl) 2960, 2868, 1731, 1460, 881 cm-1. Anal. Calcd for C23H49NO2Si2: C 64.56%; H 11.57%. Found C 64.63%; H 11.58%. [α]25D -13.9 (c 11.1, CHCl3).
N,O-bis-(Triisopropylsilyl)-glycine (2l). 81% (6.61 g, 17.0 mmol) from glycine (21.0 mmol, 1.58 g). 1H NMR (300 MHz, C6D6) δ 1.07-1.12 (m, 4H), 1.16 (d, J = 6.0 Hz, 18H), 1.21 (d, J = 6.0 Hz, 18H), 1.34-1.46 (m, 3H), 3.75 (d, J = 6.7 Hz, 2H). 13C NMR (75 MHz, C6D6) δ 11.9, 12.3, 17.9, 18.4, 46.1, 173.7. IR (neat, NaCl) 3396, 2945, 2868, 1721, 1547, 1460, 1204, 1148, 881 cm-1. GC-MS (70 eV) m/z 344 (M+-(i-Pr)).
(+)-N,O-bis-(Triisopropylsilyl)-(L)-tryptophan (2m). 85% (10.98 g, 21.3 mmol) from 5.11 g of (L)-tryptophan (25.0 mmol). 1H NMR (500 MHz, C6D6) δ 1.05 (m, 39H), 1.31 (septet, J=7.5 Hz, 3H), 3.14 (dd, J = 14.3, 5.9 Hz, 1H), 3.34 (dd, J = 14.3, 7.0 Hz, 1H), 4.09 (ddd, J = 12.4, 7.0, 5.9 Hz, 1H), 6.72 (s, 2H), 7.03 (m, 1H), 7.17 (m, 2H), 7.83 (m, 1H). 13C NMR (125 MHz, C6D6) δ 12.3, 12.3, 18.0, 18.0, 18.4, 18.6, 34.6, 57.9, 111.3, 112.0, 119.2, 119.6, 122.2, 122.7, 128.4, 136.6, 175.6. 29Si NMR (99 MHz, C6D6) δ 4.9, 20.9. IR (neat, NaCl) 3487, 3396, 2853, 2919, 1706, 1624, 1470, 892 cm-1. HRMS (EI) Exact mass calc. for C29H53N2O2Si2 517.3346; found 517.3647 (M++H). Anal. Calcd for C29H52N2O2Si2: C 67.38%; H 10.14%. Found C 67.35%; H 10.13%. Melting point, 47–48 oC. [α]25D + 19.6 (c 12.1, CHCl3).
(-)-N,O-bis-(tert-Butyldimethylsilyl)-(S)-proline (2k’). 76% (2.71 g, 7.89 mmol) from (S)-proline(10.34 mmol, 1.19 g). 1H NMR (300 MHz, CDCl3) δ 0.00 (s, 3H), 0.09 (s, 6H), 0.86 (s, s, 9H), 1.24-1.34 (m, 1H), 1.68-1.73 (m, 1H), 1.9-1.83 (m, 1H), 2.11-1.98 (m, 1H), 3.1-3.22 (m, 2H), 3.94 (dd, J = 2.6, 8.6 Hz, 1H). 13C NMR (75 MHz, CDCl3) δ -5.67, -5.56, -5.02, -4.96, 17.6, 20.2, 25.5, 25.7, 26.7, 31.8, 48.3, 62.6, 177.6. IR (neat, NaCl) 3160, 2940, 2843, 1711, 1470, 1363, 1076, 999, 666 cm-1 . GC-MS (70 eV) m/z 286 (M+-tert-Bu). Anal. Calcd for C13H28N2O2Si2: C 59.44%; H 10.89%. Found C 59.43%; H 11.05%. [α]25D - 12.0 (c 9.0, hexanes).
N-(Triisopropylsilyl)-1,8-diazammoniumbicyclo[5.4.0]undec-7-ene triflate (7). To a solution of DBU (1.38 g, 9.06 mmol) in dry THF (36 mL) under N2 atmosphere, 1.15 equiv of TIPSOTf (10.42 mmol, 2.8 mL) was added dropwise by syringe pump (2.80 mL/h, d = 13 mm) at room temperature. After the addition was complete, stirring was maintained for 2 h. THF was removed under vacuum. The remaining solid was washed with dry hexane (100 mL). The phases were allowed to partition and the liquid phase was removed via cannula affording 7 in quantitative yield. This substance is an air and moisture sensitive solid and must be used immediately.. 1H NMR (300 MHz, CDCl3) δ 1.06 (bs, 9H), 1.08 (bs, 9H), 1.30 (sept. J = 9.0 Hz, 3H), 1.63-1.74 (bs, 6H), 2.71-2.75 (m, 2H), 3.38 (t, J = 3.0 Hz, 2H), 3.56 (d, J = 6.0 Hz, 2H), 3.64-3.67 (m, 2H). 13C NMR (75 MHz, CDCl3) δ 13.7, 16.6, 18.0, 20.9, 23.9, 24.9, 28.0, 33.0, 43.6, 49.6, 55.5, 171.8. HRMS (EI) Exact mass calc. for C18H37N2Si 309.2726; found 309.2727 (M+- CF3SO3).
General procedure for the monosilylation of 1. To a refluxing suspension of the amino acid 1 (20.0 mmol) in dry acetonitrile (80 mL) under N2 atmosphere, TIPSOTf (20.0 mmol, 5.4 mL) was fast added. After the addition was complete, heating was maintained for 4 h. The reaction was cooled to room temperature under positive N2 pressure and the solvent was removed under vacuum. The remaining solid was washed with dry hexane (2 x 50 mL) and filtered leaving the pure 4 in quantitative yield. These substances are air and moisture sensitive solids. However, they can be stored neat at -20 ºC under an inert atmosphere for several weeks.
(+)-(S)-Proline triisopropylsilyl ester hydrotrifluoromethanesulfonate (8a). 13C NMR (75 MHz, CDCl3) δ 11.3, 11.7, 12.2, 17.4, 23.3, 28.7, 46.8, 60.6, 113.7, 117.9, 122.1, 126.3, 168.5. IR (neat, NaCl) 3498, 2884, 2679, 1721, 1598, 1460, 1358, 1296, 1240, 1163, 902, 728, 641, 569 cm-1. HRMS (EI) Exact mass calc. for C14H30NO2Si 272.2046; found 272.2040 (M+- CF3SO3). [α]25D + 15.6 (c 12.2, CHCl3).
(+)-(S)-Tryptophan triisopropylsilyl ester hydrotrifluoromethanesulfonate (8b). 1H NMR (300 MHz, CDCl3) δ 1.10-1.15 (m, 18H), 1.32-1.40 (m, 3H), 3.1-3.3 (m, 1H), 3.5-3.6 (m, 1H), 4.0-4.2 (bs, 1H), 6.88 (bs, 3H), 7.0-7.1 (m, 2H), 7.29 (s, 1H), 7.32-7.37 (m, 1H), 7.43-7.45 (m, 1H), 9.0 (bs, 1H). 13C NMR (75 MHz, CDCl3) δ 11.8, 17.5, 26.3, 54.3, 105.6, 112, 117.7, 119.5, 122.0, 126.0, 136.6, 168.1. HRMS (EI) Exact mass calc. for C20H33N2O2Si 361.2311; found 361.2310 (M+- CF3SO3). [α]25D – 12.0 (c 5.0, THF).
General procedure for the trisilylation of α-amino acids 1 with reactive side chains.
To a refluxing suspension of the amino acid (20.0 mmol) in dry THF (120 mL) under N2 atmosphere, EDIPA (40.0 mmol, 6.97 mL) was added. TIPSOTf (60 mmol, 16.1 mL) was added dropwise by syringe pump (1.5 mL / h, d = 20 mm), avoiding overheating. The solid amino acid gradually entered into solution. After the addition was complete, heating was maintained for 6 h. The reaction was cooled to room temperature under positive N2 pressure and THF was removed under vacuum. The remaining oil was diluted in dry hexane (100 mL) and stirred for a few minutes. The phases were allowed to partition and the upper phase was filtered via cannula through neutral alumina (Brockmann, activity [I]) into another flask. The ethyldiisopropylammonium salt phase was washed with hexane (2 x 50 mL) and filtered. The organic extracts were combined and concentrated. TIPSOH was slowly distilled (bp 40-42 °C, 0.4 torr) leaving the pure material 10. These substances are air and moisture sensitive oils. However, they can be stored in dry hexane at -20 ºC under an inert atmosphere for several weeks.
(+)-N,O,O-tris-(Triisopropylsilyl)-(S)-serine (10a): 88% (7.57 g, 13.20 mmol) from 1.58 g of (S)-serine (15.0 mmol). 1H NMR (300 MHz, CDCl3) δ 1.06 (m, 58H), 1.30 (m, 6H), 3.52 (ddd, J = 12.9, 5.6, 3.4 Hz, 1H), 3.75 (dd, J = 9.1, 5.7, 3.8 Hz, 1H), 4.00 (dd, J = 9.1, 3.4 Hz, 1H). 13C NMR (75 MHz, CDCl3) δ 11.8, 12.0, 12.0, 17.9, 18.0, 18.3, 58.7, 68.6, 174.2. IR (neat, NaCl) 3400, 2945, 2868, 1726, 1465, 881, 784 cm-1. HREIMS (DIP) Exact mass calc. for C30H67NO3Si3 530.3881; found 530.3870 (M+-C3H7). [α]20D +4.1 (c 6.5, hexanes).
(+)-N,O,S-tris-(Triisopropylsilyl)-(S)-cysteine (10b): 85% (10.03 g, 17.02 mmol) from 2.42 g of (S)-cysteine (19.97 mmol). 1H NMR (300 MHz, C6D6) δ 0.97 to 1.54 (m, 63H), 2.93 (dd, J=11.0, 7.1 Hz, 1H), 3.06 (dd, J=11.0, 4.3 Hz, 1H), 3.88 (ddd, J=12.8, 7.1, 4.3 Hz, 1H). 13C NMR (75 MHz, C6D6) δ 12.2, 12.4, 13.0, 18.2, 18.5, 18.7, 33.8, 58.6, 173.9. 29Si NMR (59.6 MHz, C6D6) 5.1, 22.2, 23.2. IR (neat, NaCl) 3395, 2950, 2863, 1721, 1460, 881 cm-1; HRMS (EI) Exact mass calc. for C30H67NO2SSi3 588.4122; found 588.4132 (M+-1); Anal. Calcd for C30H66NO2SSi3: C 61.05%; H 11.44%. Found C 61.11%; H 11.56%. [α]20D +6.8 (c 23.7, CCl4).
(+)-N,O,O-tris-(Triisopropylsilyl)-(S)-tyrosine (10c): 86% (11.18 g, 17.21 mmol) from 3.62 g of (S)-tyrosine (20.0 mmol). 1H NMR (300 MHz, CDCl3) δ 0.97 (m, 18H), 1.06 (m, 36H), 1.23 (m, 9H), 1.56 (broad s, 1H), 2.81 (dd, J = 13.5, 6.2 Hz, 1H), 2.91 (dd, J = 13.5, 6.7 Hz, 1H), 3.70 (broad m, 1H), 6.75 (dd, J = 6.5, 2.0 Hz, 2H), 7.04 (dd, J = 6.5, 2.0 Hz, 2H). 13C NMR (75 MHz, CDCl3) δ 11.9, 12.0, 12.7, 17.8, 17.9, 18.2, 18.3, 43.5, 58.4, 119.4, 130.0, 130.4, 154.7, 175.3. IR (neat, NaCl) 3380, 2934, 2852, 1721, 1608, 1501, 1460 cm-1. HRMS (EI) Exact mass calc. for C33H64NO3Si3 606.4194; found (M+-C3H7) 606.4203. Anal. Calcd for C36H71NO3Si3: C 66.50%; H 11.01%. Found C 66.72%; H 11.08%. [α]20D +7.58 (c 13.6, hexanes).
(+)-N,O,O-tris-(Triisopropylsilyl)-(2S,3R)-threonine (10d): 78% (3.20 g, 5.44 mmol) from 0.83 g of (2S,3R)-threonine (6.98 mmol). 1H NMR (300 MHz, CDCl3) δ 4.08 (m, 1H), 3.63 (dd, J= 12.86, 4.15 1H), 1.40-1.25 (m, J= 6.3, 9H), 1.10-1.03 (m, 58H), 13C NMR (75 MHz, CDCl3) δ 173.6, 72.2, 61.7, 18.31, 18.30, 18.13, 18.11, 17. 93, 12.5, 12.06, 11.98. GC-MS (EI) m/z: 544 (M+-C3H7). Anal Cal. for C31H69NO3Si3: C 63.31%; H 11.82%. Found C 63.12%; H 11.92. [α]25D +18.97 (c 10.7, hexanes).
(+)-N,O,N’-tris-(Triisopropylsilyl)-(S)-lysine (10e): 62% (1.60 g, 2.60 mmol) from 0.61 g of (S)-lysine (4.17 mmol). 1H NMR (300 MHz, CDCl3) δ 0.20 (bs, 1H), 1.00-1.08 (m, 58H), 1.15-1.41 (m, 10H), 1.60-1.64 (m, 2H), 2.74 (bs, 2H), 3.46-3.54 (m, 1H). 13C NMR (75 MHz, CDCl3) δ 11.7, 11.8, 12.0, 17.8, 18.2, 18.3, 22.4, 35.1, 38.0, 42.7, 56.5, 175.9. IR (neat, NaCl) 3392, 2942, 2892, 2866, 1721, 1463, 1249, 1191, 995, 883, 671 cm-1. HRMS (EI) Exact mass calc. for C33H75N2O2Si3 615.5136; found (M++H) 615.5136. [α]25D +12.4 (c 2.1, hexanes).
Bis-Triisopropylsilyl (-)-(S)-(N)-triisopropylsilylaspartate (10f): 77% (1.88 g, 3.13 mmol) from 0.54 g of (S)-aspartic acid (4.06 mmol). 1H NMR (300 MHz, C6D6) δ 0.98-1.12 (m, 54H), 1.16-1.35 (m, 9H), 1.51 (d, J = 12.2 Hz, 1H), 2.74 (dd, J = 6.1, 17.0 Hz, 1H), 2.96 (dd, J = 3.3, 17.0 Hz, 1H), 3.69-3.74 (m, 1H). 13C NMR (75 MHz, C6D6) δ 12.17, 12.25, 12.4, 18.0, 18.1, 18.5, 43.6, 53.3, 171.1, 174.1. HRMS (EI) Exact mass calc. for C31H68NO4Si3 602.4456; found (M++H) 602.4453. [α]25D -2.1 (c 8.9, hexanes).
General Procedure to prepare trisilylated tryptophans (11). To a solution of 2m in THF (0.2 M) at -78 °C, 1 equiv of tert-butyllithium (1.7 M solution in hexanes) was added dropwise. The solution turned dark yellow to orange. After 15 min, 1 equiv of R3SiX was slowly added. The solution became colorless to pale yellow. The reaction was quenched with 1 mL of dry MeOH and allowed to reach room temperature. The reaction mixture was concentrated under vacuum. The remaining oil was diluted in dry hexanes (20 mL/equiv of substance) and filtered via canula through neutral alumina. To explore the possibility of the organolithium process resulting in partial racemization, after the above deprotonation was complete, 1 equiv. of MeOH was slowly added and the solution became colorless to pale yellow. The reaction mixture was neutralized with 1 equiv. of TMSCl and allowed to reach room temperature. Concentration under vacuum gave an oil which was diluted in pentane (10 mL/equiv. of substance) and filtered under nitrogen through neutral alumina. After vacuuming off the pentane, the concentrated organic layer was distilled under high vacuum to recover 2m (94%) whose specific rotation remained unchanged from its original value (see above).
(+)-N,O-bis-Triisopropylsilyl-N1-trimethylsilyl-(S)-tryptophan (11a). 97% (2.91 g , 4.95 mmol) from 2.64 g of 2m (5.11 mmol mmol). 1H NMR (300 MHz, CDCl3) δ 0.54 (s, 9H), 1.00-1.06 (m, 39H), 1.12-1.30 (m, 4H), 3.01 (dd, J= 6.4, 14.2 Hz, 1H), 3.22 (dd, J= 6.6, 14.2 Hz, 1H), 3.93 (ddd, J= 6.4, 6.6, 13.0 Hz, 1H), 7.02 (s, 1H), 7.12-7.21 (m, 2H), 7.43-7.46 (m, 1H), 7.64-7.67 (m, 1H). 13C NMR (75 MHz, C6D6) δ -0.60,-0.2, 0.18, 11.49, 11.87, 11.93, 12.25, 12.33, 17.72, 17.74, 18.14, 18.26, 34.4, 57.4, 112.8, 113.8, 119.1, 119.4, 121.3, 128.2, 131.5, 140.5, 175.9. IR (neat, NaCl) 3396, 2945, 2858, 1721, 1608, 1552, 1455, 1255, 1178 cm-1. HRMS (EI) Exact mass calc. for C29H53N2O2Si3 545.3415; found 545.3395 (M+-C3H7). [α]31D +17.2 (c 4.6, pentane).
(+)-N,O-bis-Triisopropylsily-N1-triethylsilyl-(S)-tryptophan (11b). 93% (2.96 g, 4.69 mmol) from 2.60 g of 2m (5.03 mmol). 1H NMR (300 MHz, CDCl3) δ 0.28-1.25 (m, 58H), 3.14 (dd, J = 6.4, 14 Hz, 1H), 3.42 (dd, J = 6.1, 14 Hz, 1H), (ddd, J= 6.1, 6.4, 12.6 Hz, 1H), 7.19-7.29 (m, 3H), 7.42-7.52 (m, 1H), 7.80-7.95 (m, 1H). 13C NMR (75 MHz, C6D6) δ 4.6, 6.9, 12.3, 12.4, 17.7, 18.1, 18.5, 18.6, 34.3, 57.4, 113.1, 114.5, 119.4, 120.0, 121.9, 128.7, 132.2, 141.3, 175.6 IR (neat, NaCl) 3391, 2934, 2868, 1716, 1608, 1445, 1301, 1173, 1142, 1009 cm-1. HRMS (EI) Exact mass calc. for C32H59N2O2Si3 587.3884, found 587.3722. [α]25D +12.9 (c 26.7, CCl4).
(+)-N,O,N1-tris-Triisopropylsilyl-(S)-tryptophan (11c). 66% (2.15 g, 3.2 mmol) from 2.5 g of 2m (4.83 mmol). 1H NMR (300 MHz, CDCl3) δ 0.99 (m, 21H), 1.08 (d, J = 7.3 Hz, 18H), 1.18 (d, J = 7.5 Hz, 9H), 1.18 (d, J = 7.5 Hz, 9H), 1.31 (m, 3H), 1.72 (septet, J = 7.5 Hz, 3H), 3.05 (dd, J = 14.5, 6.3 Hz, 1H), 3.30 (dd, J = 14.5, 6.0 Hz, 1H), 3.99 (m, 1H), 7.14 (m, 4H), 7.48 (m, 1H), 7.64 (m, 1H). 13C NMR (75 MHz, C6D6) δ 12.3, 12.4, 13.2, 18.1, 18.3, 18.5, 18.6, 34.2, 57.5, 114.1, 114.4, 119.3, 120.0, 122.0, 129.4, 132.3, 141.7, 175.5. 29Si NMR (99 MHz, C6D6) 4.7, 12.2, 20.9. IR (neat, NaCl) 3396, 3078, 3042, 2945, 2889, 2863, 1721, 1460 cm-1. HRMS (EI) Exact mass calc. for C38H72N2O2Si3 672.4902; Found 672.4874 (M+). Anal. Calcd for C38H72N2O2Si3: C 67.79%; H 10.78%. Found C 67.94%; H 10.91%. [α]20D +10.2 (c 16.0, CCl4).
(+)-N,O-bis-Triisopropylsilyl-N1-tert-butyldimethylsilyl-(S)-tryptophan (11d). 51% (2.28 g, 3.62 mmol) from 3.67 g of 2m (7.10 mmol). 1H NMR (300 MHz, CDCl3) δ 0.52-0.60 (m, 6H), 0.92-0.99 (m, 27H), 1.00 (d, J = 1.3 Hz, 9H), (bs, 9H), 1.18-1.34 (m, 7H), 2.97 (dd, J = 6.4, 14.3 Hz, 1H), 3.18 (dd, J = 6.5, 14.3 Hz, 1H), 3.85-3.95 (m, 1H), 7.01 (s, 1H), 7.06-7.17 (m, 3H), 7.41-7.44 (m, 1H), 7.55-7.64 (m, 1H). 13C NMR (75 MHz, CDCl3) δ -4.0, 11.9, 12.0, 17.7, 18.1, 18.3, 19.4, 26.3, 34.4, 57.6, 114.1, 114.5, 119.4, 120.0, 121.9, 129.4, 132.2, 141.9, 175.5. IR (neat, NaCl) 3386, 3085, 3052, 2940, 2860, 1720, 1611, 1500, 1447, 1255, 1134 cm-1. HRMS (EI) Exact mass calc. for C32H59N2O2Si3 587.3884. Found 587.3873. [α]25D +14.6 (c 10.6, hexanes).
Deprotonation of 2j with n-BuLi, silylation and disilylation. To 2j (0.132 g, 1.00 mmol) and tetradecane (0.170 g, 1.00 mmol) in THF (10 mL) at –78 °C, n-BuLi (0.5 mL of 2.1 M in hexanes, 1.1 mmol) was added slowly with vigorous stirring. After 30 min, the solution was allowed to reach –32 °C, whereupon it was recooled to -78 °C. TMSCl (0.212 g, 1.1 mmol) was added dropwise via syringe. After 1 h, the mixture was allowed to warm to 25 °C. GC analysis revealed a 92% conversion to a new compound whose MS and IR were consistent with N-trimethylsilylation to give 12 (MS m/z (relative intensity) 517 (M+, 15), 502 (M–Me+,15), 474 (M–(i-Pr)+, 100); IR (KCl) 1734 cm-1). An aqueous solution of NH4Cl (5 mL, 10 w/v) was added to the reaction mixture which was stirred vigorously for 3 h. The mixture was extracted with hexane (25 mL), and this solution was washed with water (6 X 25 mL) dried (K2CO3) and concentrated (0.05 Torr, 2 h) to remove the solvents and tetradecane. The recovered 2j (80%, 99% GC purity) was found to have identical spectroscopic properties to the original material as well as the same optical rotation.
Triisopropylsilyl 3-methylcrotonate (13). To a well stirred solution of imidazole (4.09 g, 60 mmol), 3-methylcrotonic acid (3.02 g. 30 mmol) in dry DMF (15 mL) at 25 °C, TIPSCl (5.78 g. 30 mmol) was added dropwise. The resulting reaction mixture was allowed to stir for 5 h. Extraction with hexane (3 x 10 mL) and careful concentration using vacuum produced 6.11 g (80 %) of 13. Anal. Calcd for C14H28O2Si: C, 65.57; H, 11.00. Found: C, 65.83; H, 11.08. 1H NMR (CDC13) δ 1.09 (d, J = 7.3 Hz, 18H), 1.28 (sept, J = 7.4 Hz, 3H), 1.88 (d, J = 1.0 Hz, 3H), 2.14 (d, J = 1.0 Hz, 3H), 5.71 (m, 1H). 13C NMR (CDC13) δ 11.8, 17.7, 19.8, 27.1, 117.7, 155.9, 166.1. IR 1690, 1640,1220, 870 cm-1. MS (relative intensity) m/z 213 (100), 99 (6), 83 (56), 75 (78), 73 (8).
1,1-bis-(Triisopropylsiloxy)-3-methyl-1,3-butadiene (14). To a well-stirred solution of n-BuLi (3.24 mL, 1.7 M, 5,5 mmol) in dry THF (25 mL) at –78 °C, 13 (1.28 g, 5.0 mmol) was added dropwise over 20 min. After 3 h, TIPSCl (0.97 g, 5.0 mmol) was added and the mixture was allowed to warm to 25 °C. Hexane (25 mL) was added and the resulting mixture was extracted with brine (3 X 15 mL). The organic layer was dried (Na2SO4), concentrated and distilled to give 1.5 g (73%) of 14 (bp 127 °C, 0.025 Torr). Upon standing, 14 solidifies (mp 44–45 °C). 1H NMR (500 MHz, CDCl3) δ 1.12 (d, J = 7.4 Hz, 18H), 1.13 (d, J = 7.4 Hz, 18H), 1.25 (m, 6H), 1.92 (d, J = 0.6 Hz, 3H), 4.27 (s, 1H), 4.38 (dd, J = 2.8, 1.4 Hz, 1H), 4.70 (dq, J = 2.8, 0.6 Hz, 1H). 13C NMR (CDCl3) δ 13.0, 13.6, 18.0, 23.7. 86.7, 106.6, 140.8, 152.2. IR (CCl4) 3090, 1640, 1190, 600 cm-1. MS (relative intensity) m/z 412 (20), 397 (35), 75 (23), 50 (100). HRMS (EI) Exact mass calc. for C23H48O2Si2 412.3192; found 412.3181.
ACKNOWLEDGEMENTS
The support of the NSF (CHE-0848192) and NIH SCORE (S06GM8102) Program is gratefully acknowledged. We also wish to thank Bristol-Myers Squibb for their support of I.K. and this study.
References
1. T. Baldwin and M. Lapointe, ‘The Chemistry of Amino Acids. The Biology Project [Online],’ (2003) Available: http://www.biology.arizona.edu/biochemistry/. Retrieved on 2009-6-11.
2. (a) D. L. Hughes, J. J. Bergan, and E. J. J. Grabowski, J. Org. Chem., 1986, 51, 2579; CrossRef (b) M. J. Locke and R. T. McIver, J. Am. Chem. Soc., 1983, 105, 4226. CrossRef
3. R. Geiger and W. Konig, ‘The Peptides,’ Vol. 3, ed. by E. Gross and J. Meienhofer, Academic Press, Inc., New York, 1981, pp. 1-99.
4. R. W. Roeske, ‘The Peptides,’ Vol. 3, ed. by E. Gross and J. Meienhofer, Academic Press, Inc., New York, 1981, pp. 101-135.
5. M. J. O. Anteunis, C. Becu, F. Becu, and C. Roland, Bull. Soc. Chim. Belg., 1990, 99, 361.
6. C. W. Gehrke and K. R. Leimer, J. Chromatogr., 1971, 57, 219. CrossRef
7. (a) K. R. Leimer, R. H. Rice, and C. W. Gehrke, J. Chromatogr., 1977, 141, 355; CrossRef (b) T. G. Sobolevsky, E. S. Chernetsova, I. A. Revelsky, A. B. Sterostin, B. Miller, and V. Oriedo, Eur. J. Mass Spectromet., 2003, 9, 487; CrossRef (c) M. K. Rao, M. Sharma, S. K. Raza, and D. K. Jaiswal, Phosphorus, Sulfur and Silicon, 2003, 178, 559. CrossRef
8. A. Johansson, E. Ǻkerblom, K. Ersmark, G. Lindeberg, and A. Hallberg, J. Comb. Chem., 2000, 2, 496 and refs. cited therein. CrossRef
9. (a) C. Rücker, Chem. Rev., 1995, 95, 1009; CrossRef (b) J. A. Soderquist, J. Vaquer, M. J. Díaz, F. G. Bordwell, and S. Zhang, Tetrahedron Lett., 1996, 37, 2561; CrossRef (c) J. C. Justo de Pomar and J. A. Soderquist, Tetrahedron Lett., 1998, 39, 4409; CrossRef (d) J. C. Justo de Pomar and J. A. Soderquist, Tetrahedron Lett., 2000, 41, 3285; CrossRef (e) J. A. Soderquist and J. C. Justo de Pomar, Tetrahedron Lett., 2000, 41, 3537. CrossRef
10. (a) B. Soto-Cairoli, J. Justo de Pomar, and J. A. Soderquist, Org. Lett., 2008, 10, 333; CrossRef For applications to the asymmetric synthesis of β-amino alcohols, see, (b) B. Soto-Cairoli and J. A. Soderquist, Org. Lett., 2009, 11, 401. CrossRef
11. K. Matyjaszewski, Macromolecules, 1988, 21, 519. CrossRef
12. For a related N-acylamidinium intermediate, see: W.-C. Shieh, S. Dell, and O. Repic, J. Org. Chem., 2002, 67, 2188. CrossRef
13. S. Y. Venyaminov and N. N. Kalnin, Biopolymers, 1990, 30, 1243. CrossRef
14. I. M. Klotz and D. M. Gruen, J. Phys. Chem., 1948, 52, 961. CrossRef
15. (a) C. J. Chapo, J. B. Paul, R. A. Provencal, K. Roth, and R. J. Saykally, J. Am. Chem. Soc., 1998, 120, 12956; CrossRef (b) M. F. Bush, J. T. O’Brien, J. S. Prell, R. J. Saykally, and R. C. Cohen, J. Am. Chem. Soc., 2007, 129, 1612. CrossRef