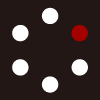
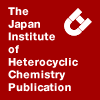
HETEROCYCLES
An International Journal for Reviews and Communications in Heterocyclic ChemistryWeb Edition ISSN: 1881-0942
Published online by The Japan Institute of Heterocyclic Chemistry
e-Journal
Full Text HTML
Received, 22nd July, 2009, Accepted, 4th September, 2009, Published online, 7th September, 2009.
DOI: 10.3987/COM-09-S(S)66
■ Enantioselective Synthesis of 2-Aryl-2,3-dihydro-4-quinolones by Chiral Brønsted Acid Catalyzed Intramolecular Aza-Michael Addition Reaction
Zhen Feng, Qing-Long Xu, Li-Xin Dai, and Shu-Li You*
State Key Laboratory of Organometallic Chemistry, Shanghai Institute of Organic Chemistry, 354 Finglin Road, Shanghai 200032, China
Abstract
Asymmetric intramolecular aza-Michael addition of activated α,β-unsaturated ketones catalyzed by chiral N-triflyl phosphoramide was realized. Enantioenriched 2-aryl-2,3-dihydroquinolin-4-ones can be obtained in excellent yields (77-98%) with up to 82% ee.2-Aryl-2,3-dihydroquinolin-4-ones are a class of heterocyclic compounds with significantly biological importance.1-3 Consequently, enormous synthetic efforts have been devoted to their efficient synthesis.4-9 Despite the recent progress towards the synthesis of 2-aryl-2,3-dihydroquinolin-4-ones, the catalytic enantioselective synthesis is rather limited. Notably, Hayashi and co-workers developed rhodium-catalyzed enantioselective 1,4-addition of arylzinc reagents to 4-quinolones, leading to optically pure 2-aryl-2,3-dihydro-4-quinolones.10 More interestingly, during the studies on testing 2-aryl-2,3-dihydro-4-quinolones as a new class of antitumor agents,1-2 their two enantiomers were found to display distinct activities. This difference in reactivity of two enantiomers together with only limited enantioselective synthesis has made that the development of efficient asymmetric catalytic synthesis of 2-aryl-2,3-dihydro-4-quinolones is urgent and highly desirable. As a straightforward synthesis, the route to 2-aryl-2,3-dihydro-4-quinolones by intramolecular aza-Michael addition of enone is very attractive. However, the catalytic asymmetric synthesis by this means is still unknown despite of the fact that the intermolecular aza-Michael addition of enone have been demonstrated efficiently by several groups.11-15 As part of our ongoing program in exploring chiral Brønsted acid catalysis,16-18 we recently realized the chiral Brønsted acid-catalyzed asymmetric intramolecular oxa-Michael addition of activated α,β-unsaturated ketones.19 The flavanone products can be synthesized with excellent yields (50-95%) and up to 74% ee by chiral N-triflyl phosphoramide.20-22 We then envisaged that chiral N-triflyl phosphoramide might be suitable for the aza-Michael addition of activated α,β-unsaturated ketones to afford the enantioenriched 2-aryl-2,3-dihydro-4-quinolones. In this paper, we report the preliminary study on this subject.
The initial testing of substrate 1 with chiral N-triflyl phosphoramide did not lead to the cyclization product. To increase the reactivity of enone, tert-butyl ester activated α,β-unsaturated ketone 2a is employed as substrate, and the tert-butyl ester moiety can be removed after the cyclization.23-24 To our delight, substrate 2a underwent the cyclization in the presence of chiral N-triflyl phosphoramide smoothly. Several chiral N-triflyl phosphoramide catalysts have been tested, and the results are summarized in Table 1. All the tested chiral N-triflyl phosphoramides were able to catalyze this reaction smoothly with excellent yields. The best enantioselectivity (72% ee) was obtained when (S)-4g, catalyst bearing 1-naphthyl substituent, was used (entry 7, Table 1).
With 10 mol% of (S)-4g, N-Ac substrate 2a was chosen to further optimize the reaction conditions. As summarized in Table 2, several commonly used solvents have been tested. Nonpolar solvents such as toluene, p-xylene and CCl4 were all well tolerated, giving almost identical yields and ees. The optimized reaction condition was chosen as the following: 10 mol% (S)-4g, 60 ˚C, and toluene as the solvent.
Under these optimized reaction condition, different substrates were explored to examine the generality of the reaction.25 The results are summarized in Table 3. A variety of aryl groups could be accommodated in the reaction, and the corresponding products were obtained in high yields with moderate enantioselectivities (77-98% yields, 4-82% ees). However, substrate 2d bearing NO2 led to the cyclization product in only 77% yield with a low ee (entry 4, Table 3). When the substrate 2g with electron-donating group (MeO) at ortho-position was used, excellent yield could be obtained, but with only 4% ee (entry 7, Table 3). The para-tolyl substituted substrate 2e gave the highest enantioselectivity (82% ee, entry 5, Table 3). The 1-naphthyl and 2-naphthyl substituted substrates gave the good enantioselectivity (76% ee, entries 8,9, Table 3).
In order to determine the absolute configuration of the products obtained here, the enantiopure 3b was obtained preparative chiral HPLC separation. The crystal structure of enantiopure 3b was obtained, and a single-crystal X-ray analysis determined its configuration as S (Figure 1).
In summary, we have developed the asymmetric intramolecular aza-Michael addition of activated α,β-unsaturated ketones by utilizing chiral N-triflyl phosphoramide. The enantioenriched 2-aryl-2,3-dihydroquinolin-4-ones can be synthesized in excellent yields (77-98%) with up to 82% ee. Further tuning the catalyst to broaden the substrate scope is ongoing in the lab.
ACKNOWLEDGEMENTS
We thank the National Natural Science Foundation of China (20732006, 20821002), National Basic Research Program of China (973 Program 2010CB833300), and the Chinese Academy of Sciences for generous financial support.
References
1. Y. Xia, Z. Y. Yang, P. Xia, K. F. Bastow, Y. Tachibana, S. C. Kuo, E. Hamel, T. Hackl, and K. H. Lee, J. Med. Chem., 1998, 41, 1155. CrossRef
2. S. X. Zhang, J. Feng, S. C. Kuo, A. Brossi, E. Hamel, A. Tropsha, and K. H. Lee, J. Med. Chem., 2000, 43, 167. CrossRef
3. N. Hoettecke, S. Rotzoll, U. Albrecht, M. Lalk, C. Fischer, and P. Langer, Bioorg. Med. Chem., 2008, 16, 10319. CrossRef
4. J. F. Wang, Y. X. Liao, P. Y. Kuo, Y. H. Gau, and D. Y. Yang, Synlett, 2006, 2791. CrossRef
5. N. Ahmed and J. E. van Lier, Tetrahedron Lett., 2006, 47, 2725; CrossRef N. Ahmed and J. E. van Lier, Tetrahedron Lett., 2007, 48, 13. CrossRef
6. S. Chandrasekhar, K. Vijeender, and Ch. Sridhar, Tetrahedron Lett., 2007, 48, 4935. CrossRef
7. N. O. Townsend and Y. A. Jackson, Heterocycles, 2007, 71, 669. CrossRef
8. D. Solé and O. Serrano, J. Org. Chem., 2008, 73, 9372. CrossRef
9. D. Kumar, G. Patel, B. G. Mishra, and R. S. Varma, Tetrahedron Lett., 2008, 49, 6974. CrossRef
10. R. Shintani, T. Yamagami, T. Kimura, and T. Hayashi, Org. Lett., 2005, 23, 5317. CrossRef
11. L. W. Xu and C. G. Xia, Eur. J. Org. Chem., 2005, 633. CrossRef
12. Y. K. Chen, M. Yoshida, and D. W. C. MacMillan, J. Am. Chem. Soc., 2006, 128, 9328. CrossRef
13. M. P. Sibi and K. Itoh, J. Am. Chem. Soc., 2007, 129, 8064. CrossRef
14. P. Diner, M. Nielsen, M. Marigo, and K. A. Jørgenson, Angew. Chem. Int. Ed., 2007, 46, 1983. CrossRef
15. X. J. Lu and L. Deng, Angew. Chem. Int. Ed., 2008, 47, 7710. CrossRef
16. For reviews on chiral Brønsted acid catalysis: T. Akiyama, Chem. Rev., 2007, 107, 5744; CrossRef M. Terada, Chem. Commun., 2008, 4097; CrossRef X. H. Yu and W. Wang, Chem. Asian J., 2008, 3, 516. CrossRef
17. Q. Kang, Z. A. Zhao, and S. L. You, J. Am. Chem. Soc., 2007, 129, 1484; CrossRef Q. Kang, Z. A. Zhao, and S. L. You, Adv. Synth. Catal., 2007, 349, 1657; CrossRef Q. Kang, X. J. Zheng, and S. L. You, Chem. Eur. J., 2008, 14, 3539; CrossRef M. Zeng, Q. Kang, Q. L. He, and S. L. You, Adv. Synth. Catal., 2008, 350, 2169; CrossRef Q. Kang, Z. A. Zhao, and S. L. You, Org. Lett., 2008, 10, 2031; CrossRef Y. F. Sheng, G. Q. Li, and S. L. You, Chem. Eur. J., 2009, 15, 3351; CrossRef Q. Kang, Z. A. Zhao, and S. L. You, Tetrahedron, 2009, 65, 1603; CrossRef Y. F. Sheng, Q. Gu, A. J. Zhang, and S. L. You, J. Org. Chem, 2009, 74, 6869. CrossRef
18. For a review: S. L.You, Q. Cai, and M. Zeng, Chem. Soc. Rev., 2009, 38, 2190. CrossRef
19. Z. Feng, M. Zeng, Q.-L. Xu, and S. L. You, Chin. Sci. Bull., 2009, in press.
20. D. Nakashima and H. Yamamoto, J. Am. Chem. Soc., 2006, 128, 9626; CrossRef P. Jiao, D. Nakashima, and H. Yamamoto, Angew. Chem. Int. Ed., 2008, 47, 2411; CrossRef C. H. Cheon and H. Yamamoto, J. Am. Chem. Soc., 2008, 130, 9246. CrossRef
21. M. Rueping, W. Ieawsuwan, A. P. Antonchick, and B. J. Nachtsheim, Angew. Chem. Int. Ed., 2007, 46, 2097; CrossRef M. Rueping, B. J. Nachtsheim, S. A. Moreth, and M. Bolte, Angew. Chem. Int. Ed., 2008, 47, 593; CrossRef M. Rueping, T. Theissmann, A. Kuenkel, and R. M. Koenigs, Angew. Chem. Int. Ed., 2008, 47, 6798. CrossRef
22. D. Enders, A. A. Narine, F. Toulgoat, and T. Bisschops, Angew. Chem. Int. Ed., 2008, 47, 5661. CrossRef
23. M. M. Biddle, M. Lin, and K. A. Scheidt, J. Am. Chem. Soc., 2007, 129, 3830. CrossRef
24. L. Wang, X. Liu, Z. Dong, X. Fu, and X. Feng, Angew. Chem. Int. Ed., 2008, 47, 8670. CrossRef
25. Selected data for new compounds: 3g: white solid, 95% yield, 4% ee, [α]D20 +3.7o (c 0.30, acetone). 1H NMR (300 MHz, CDCl3) δ 2.41 (s, 3H), 3.19-3.35 (m, 2H), 3.84 (s, 3H), 6.30 (brs, 1H), 6.69 (t, J = 7.8 Hz, 1H), 6.84 (t, J = 8.1 Hz, 2H), 7.14-7.27 (m, 2H), 7.54 (t, J = 8.1 Hz, 1H), 7.68 (brs, 1H), 7.96 (d, J = 7.8 Hz, 1H); 13C NMR (75 MHz, CDCl3) δ 23.6, 42.8, 52.9, 55.2, 110.6, 120.2, 124.8, 124.9, 125.8, 126.1, 127.0, 127.5, 129.0, 134.4, 142.7, 156.9, 170.0, 193.4; IR (film) 3002, 1683, 1664, 1653, 1600, 1479, 1461, 1365, 1317, 1292, 1255, 1228, 1211, 1109, 1033, 775, 758, 418 cm-1; MS (EI): 295 (M+, 27), 253 (38), 252 (100), 146 (51), 119 (22), 91 (23), 77 (12), 43 (22); HRMS (EI): Exact mass calcd for C18H17NO3 [M]+: 295.1208. Found: 295.1211. mp 198 - 200 °C. Chiral HPLC analysis:Daicel Chiralpak AD-H (25 cm), Hexanes / IPA = 80 / 20, 0.6 mL/min, λ = 230 nm, t (major) = 26.89 min, t (minor) = 24.82 min. 3h: white solid, 81% yield, 76% ee, [α]D20 +8.9° (c 0.30, acetone). 1H NMR (300 MHz, CDCl3) δ 2.29 (s, 3H), 3.37-3.41 (m, 2H), 6.85 (brs, 1H), 7.02 (d, J = 7.8 Hz, 1H), 7.00-7.17 (m, 4H), 7.47-7.57 (m, 3H), 7.79 (d, J = 8.1 Hz, 1H), 8.00 (d, J = 7.5 Hz, 1H), 8.35 (d, J = 8.4 Hz, 1H); 13C NMR (75 MHz, CDCl3) δ 23.0, 43.3, 52.1, 123.7, 124.4, 125.4, 125.8, 126.0, 126.4, 126.9, 127.0, 127.4, 128.7, 129.0, 131.4, 133.6, 133.7, 134.2, 141.7, 169.5, 194.5; IR (film) 3049, 2966, 1687, 1663, 1598, 1481, 1363, 1293, 1276, 1228, 794, 774, 539 cm-1; MS (EI): 315 (M+, 56), 273 (77), 272 (100), 154 (24), 153 (39), 152 (26), 146 (70), 141 (17), 128 (19), 90 (10), 43 (28); HRMS (EI): Exact mass calcd for C21H17NO2 [M]+: 315.1259. Found: 315.1260. mp 196 – 198 °C. Chiral HPLC analysis: Daicel Chiralpak AD-H (25 cm), Hexanes / IPA = 80 / 20, 0.6 mL/min, λ = 230 nm, t (major) = 15.12 min, t (minor) = 16.92 min. 3i: white solid, 98% yield, 76% ee, [α]D20 -76.1o( c 0.30, acetone). 1H NMR (300 MHz, CDCl3) δ 2.47 (s, 3H), 3.38-3.56 (m, 2H), 6.68 (brs, 1H), 7.10-7.54 (m, 7H), 7.69-7.72 (m, 3H), 7.92 (d, J = 7.8 Hz, 1H); 13C NMR (75 MHz, CDCl3) δ 23.3, 42.3, 54.6, 124.9, 125.0, 125.6, 126.0, 126.2, 126.3, 127.2, 127.4, 127.9, 128.6, 132.4, 132.8, 134.4, 135.4, 141.6, 170.2, 193.2; IR (film) 1664, 1601, 1480, 1459, 1379, 1379, 1283, 1228, 1116, 1025, 862, 780, 477 cm-1; MS (EI): 315 (M+, 20), 273 (40), 272 (100), 154 (17), 146 (32), 43 (19); HRMS (EI): Exact mass calcd for C21H17NO2 [M]+: 315.1259. Found: 315.1262. mp: 154 – 156 °C. Chiral HPLC analysis:Daicel Chiralpak AD-H (25 cm), Hexanes / IPA = 80 / 20, 0.6 mL/min, λ = 230 nm, t (major) = 22.00 min, t (minor) = 25.48 min.