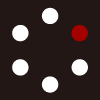
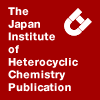
HETEROCYCLES
An International Journal for Reviews and Communications in Heterocyclic ChemistryWeb Edition ISSN: 1881-0942
Published online by The Japan Institute of Heterocyclic Chemistry
e-Journal
Full Text HTML
Received, 28th August, 2009, Accepted, 25th September, 2009, Published online, 28th September, 2009.
DOI: 10.3987/COM-09-S(S)120
■ Application of Sugar Allyltin Derivatives for the Preparation of Heterocyclic Compounds
Marta Magdycz, Piotr Cmoch, and Sławomir Jarosz*
Institute of Organic Chemistry, Polish Academy of Sciences, ul. Kasprzaka 44/52, P.O.Box 58, PL-01-224 Warszawa 42, Poland
Abstract
Controlled fragmentation of sugar allyltin, derivative of D-glucose, provided the eight carbon atom synthon: (2R,3S,4R)-tris(benzyloxy)oct-5,7-diene, which was used for the preparation of complex heterocyclic compounds. First step involved formation of the corresponding oxime, which underwent – under high pressure – the oxime/olefin cyclization (the alternative Diels-Alder product was not formed). Proper functionalization of the latter led to the tricyclic product.1. INTRODUCTION
Application of sugars as starting materials for the preparation of complex optically pure products (chiron approach1) is a well established method in synthetic organic chemistry. This approach is particularly useful in the synthesis of polyhydroxylated carbocyclic compounds which are regarded as sugar mimics.2 Such compounds (which can be prepared by a number of methods including e.g.: Ferrier-II rearrangement,3 Sinaÿ ‘molecular scissors’,4 RCM cyclization of sugar olefins,5 and many others1) possess interesting biological properties; because of their similarity to ‘normal’ sugars they efficiently block specific enzymes.6 Even more interesting are sugar analogs in which the ring oxygen atom is replaced by a nitrogen functionality (iminosugars).7
During the past years we have elaborated a convenient methodology for the preparation of enantiomerically pure carbo-bicyclic systems from sugar allyltins. The key-step consisted of a controlled fragmentation of these organometallics into isomerically pure (only E configuration across the internal double bond) dienoaldehydes, which – by proper functionalization – were converted either into bicyclo[4.4.0]decenes or bicyclo[4.3.0]nonenes (Figure 1).8
Such highly functionalized dienoaldehydes might also serve as precursors of heterocyclic derivatives. In the pioneering work by Herczegh, compound 1 was converted into the protected oxime 1a, which then was used in the intramolecular Diels-Alder (IMDA) reaction leading to the corresponding bicycle (Figure 2).9 However, the methyl group protecting the oxygen atom made this derivative useless in the preparation of sugar mimetics. The drawback laid also in non-homogeneity of the starting diene 1, which was prepared as a mixture of geometrical isomers across the internal double bond.9
2. Results and discussion
Because of the synthetic potential of such bicyclic system we decided to investigate this reaction and prepare derivatives which might be conveniently transformed into sugar mimetics, either bicyclic or monocyclic.
2.1. Synthesis of heterocyclic derivatives from dienoaldehyde
The synthesis was initiated from the readily available in our laboratory dienoaldehyde 3 (with the D-xylo-configuration), originated from the D-gluco-configurated allyltin 2.8
Reaction of the aldehyde 3 with hydroxylamine under the standard conditions afforded the corresponding oxime 4 (obtained as a mixture of syn-anti stereoisomers; see Exprerimental), which was subjected to the IMDA reaction under high (10 kbar) pressure. This process, however, did not afford the desired product 5 (which could serve as a precursor of a wide variety of highly functionalized derivatives, by reactions shown in Scheme 1), but another bicycle identified as 6, arising from the olefin/oxime cyclization10 (Scheme 1; for determination of the structure see chapter 2.2; Table 1 and Figure 4).1 High selectivity observed in such process might be rather expected, since the substituents located at both stereogenic centers (at the C4 and C6) in the vicinity of the reaction centers are at the same side of the molecule. Similar trend was observed also for more simple derivatives.10
Compound 6 has a big potential as starting material in the preparation of complex molecules. The additional functionality, the vinyl group located at the C8 position (for numbering see Scheme 1), allows for easy structural manipulations, which consequently should lead to a wide variety of mono- and bicyclic derivatives.
The most obvious choice of such functionalization, conversion of this compound into the cyclopentane 7 will not be discussed here despite its high synthetic potential (functionalization of the ‘allylic alcohol end’, i.e. the vinyl group located at the C8 position should provide a wide variety of mono-carbocyclic compounds). This transformation would involve cleavage of the N-O bond either by hydrogenation in the presence of metal catalysts (this method should be however avoided because of sensibility of the protecting groups) or by reduction with lithium aluminum hydride,11 and will rather follow the route described for more simple sugar-derived bicyclic oxazolines.10
Taking, however, advantage from the presence of the vinyl unit in the molecule of 6, we planned the synthesis of the tricyclic derivative 9 (Scheme 2).
First step involved allylation of 6 at the nitrogen atom, which was performed under the standard conditions. The resulting diolefin 8 was then subjected to the ring closing metathesis reaction (RCM)12 which afforded the target compound 9 in 97% yield. The first generation Grubbs’ catalyst was highly effective in this cyclization. The advanced NMR studies fully confirmed the structure of the product (see chapter 2.2)
The desired IMDA product (of type 5) might be, eventually, prepared from the O-protected oxime (analogously to the Herczegh procedure), so we decided to protect this oxime with benzyl group. It is known that alkylation of oximes under neutral or weakly basic conditions provides the N-alkylated products, while alkylation under strong basic conditions (involving the oxime anions) affords the O-protected derivatives.13
We have treated the oxime 4 with benzyl chloride in basic media (EtOH, EtONa) in order to protect the hydroxyl group; the results are shown in Scheme 3. After the reaction two products were isolated. First one, cyclic (formed in low yield), was identified as N-benzylated oxazoline 10, with the same, as in 6, configuration at the three newly created stereogenic centers (see chapter 2.2 and Experimental). Second product, acyclic, was identified as O-protected oxime 4a (mixture of two geometrical isomers in the 4:1 ratio); the alternative structure, nitrone 11 (arising from alkylation of the nitrogen atom in 4) should be excluded on the basis of the NMR data (for determination of the structure see chapter 2.2, Table 1 and Figure 3). Formation of the O-protected oxime 4a might be expected based on the literature data, but formation of cyclic product 10 (especially, that it was formed in low yield) raised a question: if this compound was a product of simple alkylation of 6 (formed via oxime/olefin cyclization) or (more likely) was obtained via 1,3-dipolar cycloaddition of nitrone 11.
To distinguish between these two pathways, the nitrone 11 was prepared on independent route from dienoaldehyde 3 under the standard conditions (Scheme 4). However, it could not be isolated, because underwent spontaneous cyclization providing the benzylated oxazoline 10; the intramolecular 1,3-dipolar cycloaddition was therefore very fast.
This observation allowed us to rationalize the results of the reaction of oxime 4 with benzyl chloride under strongly basic conditions. Alkylation of oxime occurred at both atoms: the oxygen – providing the protected oxime 4a and nitrogen, which furnished the nitrone spontaneously undergoing cyclization. As expected13 the O-alkylation was the major pathway in this reaction.
To obtain the desired IMDA product we have prepared the O-benzylated oxime 4a on independent route by reaction of 3 with O-benzylated hydroxylamine. However, this compound did not undergo [4+2] cyclization even under high (10 kbar) pressure (Scheme 4). This result was very surprising, since under thermal conditions reported by Herczegh, the O-methyl derivative proceeded smoothly (see Figure 2). We do not have any explanation for this phenomenon, because the cyclization reactions are usually highly accelerated by high pressure.14
2.2. Determination of the structure of oxazoline 6 and benzylated oxime 4a and tricycle 9
The structures of the products: oxime 4a (isolated from the experiment shown in Scheme 4) and oxazoline 6 were established by advanced NMR experiments (Table 1; Figures 3 and 4). Assignment of the 1H and 13C NMR chemical shift was made based on the 1H NOESY and 1H TOCSY selective experiments, as well as on results of 1H-13C HSQC and HMBC correlations. Additionally, 1H-15N HMBC sequence was employed to distinguish different types of nitrogen atoms (oxime/nitrone and amine). Results of the assignment are collected in Table 1. For oxazoline structure the NMR experiments were performed in C6D6 solution at 328K in order to receive a good 1H NMR signals separation.
The 15N gradient selected HMBC experiment performed for 4a revealed the nitrogen signal at δ+3.5 ppm (correlated with protons H4 and H3 as well as methylene protons of benzyl group). This 15N NMR chemical shift pointed unambiguously at the oxime structure (typical data are in range: +30 to -60 ppm) and excluded the nitrone one (typical δ: -75 to -110 ppm).15
The structure of 4a was evaluated also on the basis of the 1H NOESY selective experiments. NOE effects (presented in Figure 3) confirmed the (expected)8 trans geometry across the internal C=C double bond and indicated the s-trans conformation of the diene.
Determination of the structure of oxazoline 6 was also evident from the NMR spectra. Cyclization of oxime 4 led to the bicyclic system in which new single bonds: C3-C7 and C8-O1 were created, which was fully confirmed by significant changes in the 1H and especially in 13C chemical shifts (Table 1). The 13C NMR signals of the C3, C7, and C8 atoms (involved in the double bond) in oxime 4 are in typical (ca. 130-150 ppm) field, whereas – after cyclization – are shifted to much higher field (ca. 50-90 ppm). The oxime type nitrogen signal (N2) was converted into the amine type; the 15N NMR shielding strongly increased (+3.5 → -223 ppm). Analysis of the 1H NOESY selective experiments taken on H3, H6, H7, and H8 protons provided the NOE effects presented in Figure 4, which indicated that protons in pairs: H3-H7, as well as, H6-H8 are in cis-relationship. Weak NOE effects observed at H3 and H7 after irradiation of the H5 also pointed at the cis relationship between H3, H5, and H7 protons.
Configuration of the N-benzyl-bicycle 10 (formed in the reaction of oxime 4 with benzyl chloride; see Scheme 3) was identical with the oxazoline 6 which was proven by simple benzylation of the latter under the standard conditions (see Experimental).
Assignment of the structure of compound 9 was obvious from the spectral data. The mass of the molecular ion of 9 differed by 28D from the ion of the starting material (di-olefin 8), which strongly suggested elimination of ethylene molecule and pointed at the structure 9. This was fully confirmed by the NMR data in which only two olefinic signals were seen (see Experimental). Although the configuration at the ring junctions was already known (it was the same as in starting 6) it was additionally supported by the NOE values shown in Figure 5.
3. CONCLUSION
Sugar allyltin derivatives are convenient starting materials for the preparation of highly functionalized dienoaldehydes, which ‘normally’ are used for the preparation of carbobicyclic systems. However, besides this ‘traditional’ application, they might be also applied in the synthesis of heterocyclic derivatives. We have shown, that intramolecular cyclization of the oxime, obtained from the corresponding dienoaldehyde derived form sugar allyltin, proceeds efficiently and highly stereoselectively under high pressure to produce the bicyclic 1H-cyclopent[c]isoxazole. Even more effective is cyclization of the corresponding nitrone obtained from the dienoaldehyde. Additional functionality (the vinyl group), present in the bicyclic structure of he product of oxime/olefin cyclization, allowed us to prepare the tricyclic compound by simple allylation of the nitrogen atom in this isoxazole followed by ring closing metathesis. Such derivatives (bicyclic and tricyclic) have a big synthetic potential for the preparation of highly functionalized sugar mimics. The oxime/olefin cyclization is much faster than the intramolecular Diels-Alder process, which was not observed even under high pressure.
4. EXPERIMENTAL
4.1. General
The NMR spectra all compounds were measured in CDCl3 solutions (unless otherwise stated) with following spectrometers: Bruker DRX 500 (at temperature 303 K) equipped with a TBI 500SB H-C/BB-D-05 Z-G probehead, Varian-NMR-vnmrs500 (at temperature 298 K) equipped with a PFG Auto XDB (1H-19F/15N-31P 5 mm) probehead, and Varian-NMR-vnmrs600 (at temperature 299 K) equipped with a PFG Auto XID (1H/15N-31P 5 mm) indirect probehead. For diene/oxime structure benzene-D6 were chosen to receive a best 1H NMR signal separation needed in process of configuration determination. Standard experimental conditions and standard Bruker and Varian programs were used. To assign the structures under consideration following 1D and 2D experiments were employed: the 1H selective NOESY, 1H selective TOCSY, COSY, 1H-13C gradient selected HSQC and HMBC with adiabatic pulses optimized for 1J(C-H) = 146 Hz and nJ(C-H) = 8 Hz. Additionally, the 1H-15N gradient selected HMBC optimized for nJ(N-H) = 5 Hz were used to distinguish different types of nitrogen atom in molecules of oxazoline/oxime/nitrone.
The 1H and 13C NMR spectral data are given relative to the TMS signal at 0.0 ppm. Nitromethane (δ = 0.0 ppm) was used as an external standard for the 15N NMR spectra. Concentration of all solutions used for measurements was about 20-30 mg of compounds in 0.6 cm3 of solvent.
The relative configurations of the protons were determined based on 1H selective NOESY experiments using standard Varian (ChemPack 4.1) sequence. The 1H and 13C NMR signals of benzyl groups occurring at the typical δ values were omitted for simplicity.
Mass spectra were recorded with an ESI/MS Mariner (PerSeptive Biosystem) mass spectrometer. Optical rotations were measured with a Digital Jasco polarimeter DIP-360 (λ = 589 nm) for solutions in CHCl3 (c = 1) at room temperature.
Column chromatography was performed on silica gel (Merck, 70-230 or 230-400 mesh). Methylene chloride was distilled from CaH2 and THF from potassium prior to use. Organic solutions were dried over anhydrous magnesium sulfate.
2,3,4-Tri-O-benzyl-5,6,7,8-tetradeoxy-D-xylo-oct-5(E),7-dienose oxime 4
To a solution of the dienoaldehyde 38,16 (1.70 g, 3.8 mmol) in EtOH / pyridine mixture (70 mL; 6:1 v/v), hydroxylamine hydrochloride (300 mg, 4.5 mml, 1.2 equiv.) was added and the mixture was stirred for 3 h at room temperature. After that time TLC (hexane – EtOAc, 3:1) showed disappearance of the starting material and formation of two more polar products. The solution was concentrated and the residue partitioned between EtOAc and 5% aq. HCl (20 mL both). The organic phase was separated, washed with water, brine, dried and concentrated and the product was purified by column chromatography (hexane – EtOAc, 10:1) to afford a mixture of syn/anti oximes 4 (535mg, 1,15mmol; 30%) and oxazoline 6 (1.135 g; 2.48 mmol, 65%).
Data for 4: HRMS: m/z calcd for: C29H31NO4 [M+Na+]: 480.21453; found: 480.2155. 1H NMR (500 MHz) for the main isomer: δ: 3.57 (dd, J = 5.9, 4.5 Hz, 1H, H-5), 4.12 (dd, J = 7.7, 4.5 Hz, 1H, H-4), 4.18 (dd, J = 7.1, 6.3 Hz, 1H, H-6), 5.15 (m, 2H, H-10), 5.54 (dd, J = 15.2, 7.8 Hz, 1H, H-7), 6.23 (m, 2H, H-8 and H-9), 7.46 (d, J = 7.8 Hz, 1H, H-3); 13C NMR (125 MHz) δ: 76.52, 80.34, 82.74 (C-4, C-5, C-6), 118.05 (C-10), 130.32, 134.68, 136.18 (C-7, C-8, C-9), 150.05, (C-3). 1H NMR (500 MHz) for the minor isomer (selected signals): δ: 3.80 (dd, J = 7.3, 3.2 Hz, 1H, H-5), 4.3 (m, 1H, H-6), 4.40 (m, 1H, H-10), 6.92 (d, J = 6.1 Hz, 1H, H-3); 13C NMR (125 MHz) δ: 71.7, 81.2, 81.7 (C-4, C-5, C-6).
When the mixture of syn/anti oximes 4 was left for three days the NMR spectra showed only cyclic product 6.
2,3,4-Tri-O-benzyl-5,6,7,8-tetradeoxy-D-xylo-oct-5(E),7-dienose O-benzyl-oxime 4a
To a solution of the dienoaldehyde 3 (515 mg, 1.1 mmol) in MeOH (50 mL), O-benzyl-hydroxylamine (200 mg, 1.3 mmol, 1.2 equiv.) and sodium acetate (115 mg, 1.3 mmol, 1.2 equiv.) were added and the mixture was stirred till disappearance of the starting material (8 h; TLC monitoring in hexane – EtOAc, 3:1). Methanol was removed in vacuum, and the residue partitioned between EtOAc (50 mL) and water (50 mL). The organic solution was separated, washed with water, brine, dried and concentrated, and the crude product was purified by column chromatography to afford single stereoisomeric oxime 4a (442 mg, 0.81 mmol, 72%). [α] +51.1; HRMS: m/z calcd for: C36H37NO4 [M+Na+]: 570.26148; found: 570.26203.
NMR (500 MHz; see also Table 1) δ: 3.59 (dd, J = 6.2, 4.3 Hz, 1H, H-5), 4.13 (dd, J = 7.9, 4.3 Hz, 1H, H-4), 4.21 (m, 1H, H-6), 5.09-5.15 (m, 2H, H-10), 5.53 (dd, J = 15.3, 7.8 Hz, 1H, H-7), 6.18 (m, 1H, H-8); 6.26 (m, 1H, H-9); 13C NMR (125 MHz): 76.4 (C-4), 80.7 (C-6), 118.0 (C-10), 82.8 (C-5), 130.3 (C-7), 134.6 (C-8), 136.3 (C-9), 149.6 (C-3).
(7R,6R,5S,4S,3R,8R)-8-C-Vinyl-4,5,6-tri-O-benzyl-hexahydro-1H-cyclopent[c]isoxazole 6
To a solution of the dienoaldehyde 3 (370 mg, 0.84 mmol) in EtOH / pyridine mixture (35 mL; 6:1 v/v), hydroxylamine hydrochloride (70 mg, 1.00 mml, 1.2 equiv.) was added and the mixture was stirred for 3 h at room temperature. Then it was worked-up as above, but the crude product was immediately without any purification dissolved in a mixture toluene/benzene (2 mL, 4:1 v/v), placed in a high pressure apparatus17 and kept for three days at 10 kbar. Then it was allowed to reach the normal pressure, the solvent was removed in vacuum, and the crude product was isolated by column chromatography (hexane – EtOAc, 9:1) to afford derivative 6 as an oil. Yield: 298 mg (78%). [α] -60.2; HRMS: m/z calcd for C29H31NO4 [M+Na+]: 480.21453; found: 480.21407. 1H NMR (500 MHz) δ: 2.84 (ddd, J = 9.6, 6.9, 2.0 Hz, 1H, H-7), 3.78 (m, 1H, H-6), 3.83 (m, 1H, H-3), 3.90 (m, 1H, H-4), 3.96 (m, 1H, H-5), 4.38 (m, 1H, H-8), 4.59-4.83 (3x2H, O-CH2Ph), 5.24 (m, 1H, H-10a), 5.26 (m, 1H, H-10b), 5.72 (ddd, J = 16.3, 10.7, 4.5 Hz, 1H, H-9); 13C NMR (125 MHz) δ: 53.6 (C-7), 66.7 (C-3), 72.2, 72.3, 72.7 (3 x O-CH2Ph), 84.4 (C-6), 85.3 (C-4), 85.8 (C-8), 86.5 (C-5), 117.6 (C-10), 134.7 (C-9). 1H NMR (500 MHz, C6D6) δ: 2.50 (ddd, J = 9.3, 6.9, 1.6 Hz, 1H, H-7), 3.35 (dd, J = 9.3, 6.1 Hz, 1H, H-3), 3.68 (m, 1H, H-6), 3.89 (m, 1H, H-4), 3.93 (m, 1H, H-5), 4.15 (d, J = 11.8 Hz, 1H, H-8), 4.86 (ddd, J = 10.6, 1.6, 1.2 Hz, 1H, H-10a), 4.95 (ddd, J = 17.5, 1.6, 1.2 Hz, 1H, H-10b), 5.36 (ddd, J = 17.5, 10.6, 4.5 Hz, 1H, H-9); 13C NMR (125 MHz, C6D6) δ: 53.7 (C-7), 66.6 (C-3), 72.2, 72.3, 72.7 (3x O-CH2Ph); 84.8 (C-6); 85.3 (C-8); 85.5 (C-4); 86.3 (C-5); 115.9 (C-10); 135.6 (C-9). Anal. Calcd for: C29H31NO4: C, 76.12; H, 6.83; N, 3.06. Found: C, 75.88; H, 6.87; N, 3.09.
N-Allyl-(7R,6R,5S,4S,3R,8R)-8-C-Vinyl-4,5,6-tri-O-benzyl-hexahydro-1H-cyclopent[c]isoxazole 8
To a solution of oxazoline 6 (175 mg, 0.38 mmol) in MeCN (20 mL) containing potassium carbonate (150 mg), allyl bromide (0.3 mL, 3.8 mmol, 10 equiv.) was added and the mixture was boiled under reflux for 24 h. TLC analysis (hexane – EtOAc, 3:1) indicated disappearance of the starting material and formation of new less polar product. The mixture was partitioned between EtOAc (20 mL) and water (20 mL), the organic phase was separated, and the aqueous one extracted with EtOAc (20 mL). Combined organic solutions were washed with water, brine, dried, and concentrated, and the product was isolated by column chromatography (hexane – EtOAc, 3:1) to afford compound 8 (125 mg, 0.25 mmol, 65%). [α] +7.0; HRMS: m/z calcd for: C32H35NO4 [M+Na+]: 520.24583; found: 520.24612. 1H NMR (500 MHz)1 δ: 2.81 (m, 1H, H-7), 3.95 (m, 1H, H-3), 5.15-5.26 (m, 4H, H-10 and H-13), 5.84-5.98 (m, 2H, H-9 and H-12); 13C NMR (125 MHz) δ: 55.38 (C-7), 61.34 (C-11), 72.87 (C-3), 118.38, 118.57 (C-10, C-13), 133.75, 136.80 (C-9, C- 2). Anal. Calcd for: C32H35NO4: C, 77.24; H, 7.09; N, 2.81. Found: C, 77.29; H, 7.10; N, 2.82.
Synthesis of tricyclic derivative 9 by RCM reaction
To a solution of the diolefin 8 (22.5 mg, 0.045 mmol) in CH2Cl2 (15 mL) under an argon atmosphere, the first generation Grubbs’ catalyst (4 mg) was added and the mixture was boiled under reflux for 8 hours. After this time TLC (hexane – EtOAc, 3:1) indicated disappearance of the starting material and formation of new more polar product. The mixture was cooled to room temp., the solvent was removed in vacuum, and the residue was purified by column chromatography (hexane – EtOAc 10:1) to afford pure 9 as an oil (21 mg, 0.044 mmol, 97%). HRMS: m/z calcd for: C30H31NO4 [M+Na+]: 492.21453; found: 492.21539. NMR (600 MHz) δ: 2.90 (ddd, J = 18.6, 3.6, 1.9 Hz, 2H, H-11), 3.04 (~t, J = 8.4, 1H, H-7), 3.45 (m, 1H, H-3), 3.69 (~t, J = 8.0, 1H, H-6), 3.86 (m, 1H, H-4), 3.88 (m, 1H, H-5), 4.01 (d, J = 4.8 Hz, 1H, H-8), 5.44 (ddd, J = 10.0, 3.3, 1.9 Hz, 1H, H-10), 5.95 (m, 1H, H-9); 13C NMR (150 MHz): 56.23 (C-11), 60.90 (C-7), 82.69, 86.25, 87.55 (C-4, C-5, C-6), 120.91 (C-10), 130.32 (C-9).
N-Benzyl-(7R,6R,5S,4S,3R,8R)-8-C-vinyl-4,5,6-tri-O-benzyl-hexahydro-1H-cyclopent[c]isoxazole 10
The bicyclic derivative 6 (75 mg, 0.16 mmol) was dissolved in MeCN (25 mL) to which potassium carbonate (100 mg) and benzyl bromide (0.2 mL, 1.6 mmol) were added and the mixture was boiled under reflux for 6 h. After that time TLC (hexane – EtOAc, 3:1) indicated disappearance of the starting material and formation of less polar product. Solvent was removed in vacuum and the residue partitioned between water (5 mL) and EtOAc (10 mL). The organic phase was separated, washed with water, brine, dried and concentrated, and the product was purified by column chromatography (hexane – EtOAc, 10:1) to afford 10 (65 mg, 0.12 mmol, 75%). [α] -6.5; HRMS: m/z calcd for: C36H37NO4 [M+Na+]: 570.26148; found: 570.26203. NMR (500 MHz) δ: 2.81 (m, 1H, H-7), 3.60 (dd, J = 9.3, 5.6 Hz, 1H, H-3), 4.10 (m, 1H, H-6), 4.22 (m, 1H, H-4), 4.31 (t, J = 6.6 Hz, 1H, H-5), 4.46 (m, 1H, H-8), 5.20 (d, J = 10.3 Hz, 1H, H-10a), 5.30 (d, J = 17.1 Hz, 1H, H-10b), 5.90 (ddd, J = 17.1, 10.3, 7.3 Hz, 1H, H-9); 13C NMR (125 MHz) δ: 55.9 (C-7), 73.4 (C-3), 83.9 (C-4), 85.3 (C-8), 85.9 (C-6), 90.1 (C-5), 117.4 (C-10), 137.5 (C-9).
Reaction of the oxime 4 with benzyl chloride
To a solution of oxime 4 (48 mg, 0.11 mmol) in EtOH (10 mL) containing EtONa (100 mg) benzyl chloride (0.15 mL, 0.13 mmol) was added and the mixture was stirred at room temp. for 2 h. Then it was concentrated under reduced pressure and the residue was partitioned between water (15 mL) and EtOAc (15 mL). The organic phase was separated, washed with water, dried and concentrated, and the residue was purified by column chromatography (hexane – EtOAc, 4:1) to afford benzylated oxazoline 10 (10 mg, 0.018 mmol, 16%; identical in all respect with the compound prepared by benzylation of 6) and the mixture of geometrical isomers of oxime 4a (34 mg, 0.062 mmol, 56%) in the ratio 4:1 (detected by integration of signals H5 occurring at δ: 3.55 and 3.76 ppm).
In the NMR spectra of this mixture, the signals of the major stereoisomer were consistent with analogous data recorded for pure N-benzylated oxime obtained from reaction of 3 with BnO-NH2. Selected signals for the minor isomer: 1H NMR (500 MHz) δ: 3.75 (dd, J = 7.6, 2.9 Hz, 1H, H-7), 4.20 (m, 1H, H-8), 5.47 (m, 1H, H-1), 6.14 (m, 1H, H-2), 6.22 (m, 1H, H-9), 6.90 (d, J = 5.8, 1H, H-3); 13C NMR (125 MHz) δ: 72.2, 81.1, 81.6 (C-4, C-5, C-6), 118.0 (C-10), 130.3, 134.7, 136.3 (C-7, C-8, C-9), 152.3 (C-3).
Reaction of the dienoaldehyde 3 with N-benzyl-hydroxylamine
To a solution of the dienoaldehyde 3 (240 mg, 0.54 mmol) in CH2Cl2 (50 mL), N-benzyl-hydroxylamine (103 mg, 0.65 mmol, 1.2 equiv.) and magnesium sulfate (500 mg.) were added, and the mixture was stirred till disappearance of the starting material (10 h; TLC monitoring in hexane – EtOAc, 3:1). Solvent was removed in vacuum, and the residue partitioned between EtOAc (50 mL) and water (50 mL). The organic solution was separated, washed with water, brine, dried and concentrated, and the crude product was purified by column chromatography (hexane – ethyl acetate, 10:1) to afford only the bicyclic derivative 10 (identical in all respect with 10 prepared from 6 (193 mg, 0.42 mmol, 78%).
Attempts of cyclization of 4a under high pressure
The O-protected oxime 4a (one isomer obtained in the reaction of 3 was dissolved in a mixture toluene/benzene (2 mL, 4:1 v/v), placed in a high pressure apparatus, and kept for three days at 10 kbar. After this time no product was formed; only unreacted oxime was recovered (TLC and NMR analysis).
References
1. S. Hanessian, Total Synthesis of Natural Products: The Chiron Aproach, Pergamon Press, New York, 1983; B. Fraser-Reid, Acc. Chem. Res., 1996, 29, 57 and references therein.
2. Selected recent reviews and papers: A. Arjona, A. M. Gomez, J. C. Lopez, and J. Plumet, Chem. Rev., 2007, 107, 1919; CrossRef M. Long and Th. Ziegler, Eur. J. Org. Chem., 2007, 768; CrossRef J. Zhou, G. Wang, L.-H. Zhang, and X.-S. Ye, Curr. Org. Chem., 2006, 10, 625; CrossRef M. A. L. Podeschwa, O. Plettenburg, and H. J. Altenbach, Eur. J. Org. Chem., 2005, 3101; CrossRef M. A. L. Podeschwa, O. Plettenburg, and H. J. Altenbach, Eur. J. Org. Chem., 2005, 3116; CrossRef S. Freeman and T. Hudlicky, Bioorg. Med. Chem. Lett., 2004, 14, 1209 and references therein. CrossRef
3. R. J. Ferrier and S. Middleton, Chem. Rev., 1993, 93, 2779. CrossRef
4. M. Sollogub, J.-M. Millet, and P. Sinaÿ, Angew. Chem. Int. Ed., 2000, 39, 362. CrossRef
5. M. Jorgensen, P. Hadwiger, R. Madsen, A. E. Stütz, and T. M. Wrodnigg, Curr. Org. Chem., 2000, 4, 565; CrossRef (microreview) R. Madsen, Eur. J. Org. Chem., 2007, 399. CrossRef
6. B. Ganem, Acc. Chem. Res., 1996, 29, 340; CrossRef M. Bols, Acc. Chem. Res., 1998, 31, 1; CrossRef A. T. Vasella, Angew. Chem. Int. Ed., 1999, 38, 750; CrossRef A. Berecibar, C. Grandjean, and A. Siriwardena, Chem. Rev., 1999, 99, 779; CrossRef I. Robina, A. J. Moreno-Vargas, A. T. Carmona, and P. Vogel, Current Drug Metabolism, 2004, 5, 329. CrossRef
7. A. Stütz, Ed. Iminosugars as Glycosidases Inhibitors: Nojirimycin and Beyond; Wiley-VCH, Weinheim, 1999; P. Compain and O. R. Martin, Iminosugars: from synthesis to therapeutic applications; John Wiley and Sons: Chichester, 2007.
8. Microreview: S. Jarosz and A. Gaweł, Eur. J. Org. Chem., 2005, 3415. CrossRef
9. H. Herczegh, M. Zsely, L. Szilagyi, G. Batta, I. Bajza, and R. Bognar, Tetrahedron, 1989, 45, 2793. CrossRef
10. Olefin / oxime cyclization in sugar chemistry: P. J. Dransfield, S. Moutel, M. Shipman, and V. Sik, J. Chem. Soc., Perkin Trans. 1, 1999, 3349. CrossRef
11. E. M. Beccalli, G. Broggini, A. Farina, L. Malpezzi, A. Terraneo, and G. Zecchi, Eur. J. Org. Chem., 2002, 2080. CrossRef
12. A. Deiters and S. F. Martin, Chem. Rev., 2004, 104, 2199; CrossRef R. R. Schrock and A. H. Hoveyda, Angew. Chem. Int. Ed., 2003, 42, 4592; CrossRef S. J. Connon and S. Blechert, Angew. Chem. Int. Ed., 2003, 42, 1900; CrossRef RCM in synthesis of sugar mimetics: R. Madsen, Eur. J. Org. Chem., 2007, 399. CrossRef
13. A. Melman, in: The chemistry pf hydroxylamines, oximes, and hydroxamic acids, chapter 5 (in Patai Series: The chemistry of functional groups, John Wiley & Sons Ltd., 2009).
14. B. Baranowski and J. Jurczak, High Pressure Chemical Synthesis; Elsevier: New York, NY, USA, 1989; F. B. Lopez, R. M. J. Egberink, D. N. Reinhoudt, and W. Verboom, Tetrahedron, 2008, 64, 10023; CrossRef E. Kozłowska, S. Jarosz, and A. Jeżewski, Tetrahedron, 1997, 53, 10775. CrossRef
15. M. Witanowski, L. Stefaniak, and G. A. Webb, Annual Report of NMR Spectroscopy, 1977, 7, 200; M. Witanowski, L. Stefaniak, and G. A. Webb, Annual Report of NMR Spectroscopy, 1981, 11b, 378 (ed. by G. A. Webb, Academic Press).
16. E. Kozłowska and S. Jarosz, J. Carbohydr. Chem., 1994, 13, 889. CrossRef
17. J. Jurczak, M. Chmielewski, and S. Filipek, Synthesis, 1979, 41; CrossRef J. Jurczak and T. D. Gryko, in “Chemistry under Extreme or Non-Classical Conditions”, 1997, chapter 4 (ed. by R. van Eldik and C. D. Hubbard, Wiley & Sons, Inc. and Spektrum Akademischer Verlag Co-Publication).