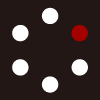
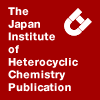
HETEROCYCLES
An International Journal for Reviews and Communications in Heterocyclic ChemistryWeb Edition ISSN: 1881-0942
Published online by The Japan Institute of Heterocyclic Chemistry
e-Journal
Full Text HTML
Received, 27th August, 2009, Accepted, 13th October, 2009, Published online, 13th October, 2009.
DOI: 10.3987/COM-09-S(S)117
■ Observation of 2,7-Disubstitution in Palladium Catalysed Directed C-H Activation of Indoles
Guilia Fanton, Nicola M. Coles, Andrew R. Cowley, Jonathan P. Flemming, and John M. Brown*
Chemistry Research Laboratory, University of Oxford, Mansfield Road, Oxford OX1 3TA, U.K.
Abstract
In previous work, controlled C-H activation and catalytic Heck reaction at the 2-position of indole was demonstrated. This was achieved by means of a N-(2-pyridylmethyl) directing group. In the course of extending the initial observations it was discovered that the corresponding N-(1-isoquinolylmethyl)indole derivative was prone to a further oxidative Heck reaction giving rise to a 2,7-disubstituted product, which was characterised by NMR and X-ray analysis. The parent pyridine showed no tendency for a second substitution reaction at the indole 7-position, but the related N-(2-quinolylmethyl) derivative did. In the case of the corresponding 6-methylphenanthridinyl derivative, a novel oxidative C-C cleavage reaction was observed.Catalytic C-H activation/coupling reactions afford the means of introducing a range of substituents into aromatic and heterocyclic compounds under milder conditions than traditional substitution protocols.1 For this reason the field has been extensively studied in recent years, and palladium catalysts have played a paramount role.2 In the case of indole, the 3-position is normally far more reactive than the 2-position to electrophilic activation, which is in turn far more reactive than sites in the 6-membered ring.3 The special position of 2-substituted indoles as “privileged” structures in medicinal chemistry,4 has provided a focus for C-H activation chemistry. For preformed indoles, the reactions employed include arylation,5 borylation,6 and alkenylation.7 In the last-named area directed7a and non-directed7b oxidative Heck reactions have been demonstrated, following early work by Fujiwara and Moritani.8 In a very recent publication, the utilisation of an N-(2-pyridinesulfonyl) directing group provides an entry for a wide range of 2-substituted indoles, and the directing group is readily removed.7c A carboxyl group at the 3-position of indole may direct reaction to the 2-position.7d The procedures available for catalytic activation of indole alkenylation at the 2-position are summarised in Scheme 1 below.
Variation of the heterocyclic directing group was examined in the course of further exploration of the catalytic reaction. In our earlier work shown in Scheme 1, it was noted that species capable of forming a stable palladacycle were inactive towards alkenylation.9 For this reason, initial efforts were concentrated on direct analogues of N-(2-pyridylmethyl)indole 1; the 1-isoquinolyl derivative 3 was examined first. 10 The reported conditions were applied to the reaction, and the extent of product formation followed by NMR. As expected, a new peak appears at 6.6 ppm (JHH 15.5 Hz), assigned to C2’ of the new alkene 4, and the reaction occurs with comparable facility to that of compound 1. As the reaction proceeded however, a further peak was observed in the alkene region of the 1H NMR spectrum that grew over time (d 6.15 ppm, JHH 15.5 Hz) ultimately at the expense of the initial product 4. It was also seen that formation of the second product 5 was facilitated under more forcing conditions, and its proportion was higher as the catalyst:substrate ratio was increased. Both NMR and MS evidence intimated that the second product was derived by double alkenylation.
By isolating the initial product 4 (44% yield) and subjecting it to a second cycle of the oxidative Heck procedure, it proved possible to obtain a pure sample of compound 5, isolated in 26% yield after flash chromatography.11 The comparative 1H nOe experiments indicated that both of the alkenyl groups in compound 5 were in proximity to the N-methylene group (Figure 1a), indicating 2- and 7-disubstitution. This was confirmed by recrystallisation of a sample of the new product by slow diffusion of pentane into a solution of the compound in chloroform, which gave X-ray quality yellow crystals. The structure is shown in Figure 1b.12
With the disubstituted compound 5 fully characterised it was of interest to see whether this reaction pathway was a more general one. Compound 2 from the original experiments was re-reacted under moderately forcing conditions (4 eq. methyl acrylate, 4 eq. Cu(OAc)2, 10 mol% Pd(OAc)2, AcOH, dioxane, 70 ˚C) but no new product was observed after three days. The related reactant 2-((1H-indol-1-yl)methyl)quinoline 6 was prepared, and subjected to the standard conditions for an oxidative Heck reaction with methyl acrylate. Reaction occurred smoothly and the monosubstitution product 7 was isolated in 53% yield. At that stage disubstitution was not evident, and hence the product was subjected again to the reaction conditions with fresh reagents for several days. A new product was indeed isolable but in just 6% yield. Structure 8 was confirmed by standard means, augmented by a NOESY experiment that showed the expected characteristic features associated with 2,7-disubstitution (Scheme 3).
Since the main drawback in the existing methodology is the lack of a method for removal of the directing group, use of a phenanthridine was considered. This might be expected to be more prone to oxidative reactions that regenerated the indole product with a free N-H group.13 The reactant 9 was prepared as before and subjected to the standard alkenylation conditions (Scheme 4). Tlc analysis of the reaction mixture indicated several products, two in particular being isolated in low yield. The only Heck product obtained was the parent compound 10, together with small quantities of an oxidation product 11 derived from reactant 9. Subjecting the reactant 9 to the same conditions in the absence of methyl acrylate did not lead to cleavage of the indole N-substituent.
Finally, direct access to a 7-substituted indole was attempted by blocking the 2-position through silylation, since both catalytic and non-catalytic approaches to functionalise the 7-position are precedented.14 This first involved synthesis of the known compound 12.15 The silyl compound was subjected to the conditions of the oxidative Heck reaction, and the product of 2–alkenylation 13 isolated in 70% yield. This was compared directly with the literature data for compound 13 and its 3-substituted isomer 14,16 demonstrating that the reaction is regiospecific. Several examples of catalytic replacement of an arylsilyl group by an alkene have recently been reported.17 In those cases, a directing group was required to facilitate the reaction, and a palladacyclic intermediate is involved. It seems that the highly nucleophilic indole ring can undergo a direct electrophilc aromatic substitution by a charged Pd reagent to form a 2-palladated intermediate - the cation also being β-silyl stabilised - that then follows a conventional path to the alkenylated product.
Why should efficient access to the disubstitution pathway be confined to the isoquinolyl derivative 3? The possibility exists that the isoquinoline can adopt a conformation favourable to 7-activation that is less accessible to the corresponding pyridine and other directing groups. In order to test this, the monosubstitution products 2 and 4 were subjected to a conformational search using the Merck MMFF Force Field.18 The results are shown in Table 2, and Figure 2. They indicate that both compounds 2 and 4 have access to low-lying conformations in which the nitrogen lone-pair is in syn-relation to C7. In the isoquinoline the distance from the lone-pair to H7 at the reaction site is shorter in the conformations favouring its participation. This difference could account for their distinct behaviour in oxidative Heck reactions.
In conclusion, the C7- position in indole becomes accessible to Pd-catalysed coupling chemistry, by an N-methylene-1-isoquinolyl directing group. This opens up the challenge of more specific positional activation through further improvements in the design of directing groups.
ACKNOWLEDGEMENTS
We thank Johnson-Matthey for the loan of palladium salts, and EPSRC and the European Community for support of JPF and GF respectively. JMB is grateful to the Leverhulme Trust for a Fellowship.
References
1. K. I. Goldberg and A. S. Goldman, ACS Symp. Ser., 2004, 885, and articles therein; J. Q. Yu, R. Giri, and X. Chen, Org. Biomol. Chem., 2006, 4, 4041; CrossRef L. Ackermann, Top. Organomet. Chem., 2007, 24, 35. CrossRef
2. S. Cacchi and G. Fabrizi, Chem. Rev., 2005, 105, 2873; CrossRef L. Joucla and L. Djakovitch, Adv. Synth. Catal., 2009, 351, 673. CrossRef
3. J. A. Joule and K. Mills, Heterocyclic Chemistry, Fourth Edition; Blackwell Publishing, Oxford, 2000.
4. R. de Sa Alves Fernando, J. Barreiro Eliezer, and M. Fraga Carlos Alberto, Mini Rev Med Chem, 2009, 9, 782. CrossRef
5. E.g. H. Ohno, M. Iuchi, N. Fujii, and T. Tanaka, Org. Lett., 2007, 9, 4813; CrossRef N. Schwarz, A. Pews-Davtyan, K. Alex, A. Tillack, and M. Beller, Synthesis, 2007, 3722; CrossRef D. R. Stuart, E. Villemure, and K. Fagnou, J. Am. Chem. Soc., 2007, 129, 12072; CrossRef J. L. Zhao, Y. H. Zhang, and K. Cheng, J. Org. Chem., 2008, 73, 7428; CrossRef N. Lebrasseur and I. Larrosa, J. Am. Chem. Soc., 2008, 130, 2926. CrossRef
6. T. Ishiyama, J. Takagi, J. F. Hartwig, and N. Miyaura, Angew. Chem. Int. Ed., 2002, 41, 3056. CrossRef
7. a) E. Capito, J. M. Brown, and A. Ricci, Chem. Commun., 2005, 1854; CrossRef b) N. P. Grimster, C. Gauntlett, C. R. A. Godfrey, and M. J. Gaunt, Angew. Chem. Int. Ed., 2005, 44, 3125; CrossRef c) A. Garcia-Rubia, R. G. Arrayas, and J. C. Carretero, Angew. Chem. Int. Ed., 2009, 48, 6511; CrossRef d) A. Maehara, H. Tsurugi, T. Satoh, and M. Miura, Org. Lett., 2008, 10, 1159. CrossRef
8. Y. Fujiwara, I. Moritani, M. Matsuda, and S. Teranishi, Tetrahedron Lett., 1968, 9, 3863; CrossRef for more recent pertinent examples see, e.g.: C. G. Jia, W. J. Lu, T. Kitamura, and Y. Fujiwara, Org. Lett., 1999, 1, 2097; CrossRef M. D. K. Boele, G. P. F. van Strijdonck, A. H. M. de Vries, P. C. J. Kamer, J. G. de Vries, and P. van Leeuwen, J. Am. Chem. Soc., 2002, 124, 1586. CrossRef
9. R. I. Cooper, A. R. Cowley, E. Capito, J. M. Brown, and A. Ricci, Acta Crystallogr., 2005, E61, m585; A. R. Cowley, R. I. Cooper, E. Capito, J. M. Brown, and A. Ricci, Acta Crystallogr., 2005, E61, m582.
10. Synthesis of heterobenzyl bromide precursors: For isoquinoline, quinoline see: B. R. Brown, D. L. Hammick, and B. H. Thewlis, J. Chem. Soc, 1951, 1145; CrossRef for phenanthridine see I. M. Clarkson, A. Beeby, J. I. Bruce, L. J. Govenlock, M. P. Lowe, C. E. Mathieu, D. Parker, and K. Senanayake, New J. Chem., 2000, 24, 377. CrossRef
11. Characterisation details for compound 4: Oil. νmax/cm-1: 3106, 2917, 1730, 1601, 1540, 1503, 896, 817, 736. 1H NMR (400 MHz, CDCl3) δ: 8.34 (1H, d, J = 5.7 Hz, H-3’), 8.10 (1H, d, J = 8.4 Hz, H-5’), 7.84 (1H, d, J = 8.1 Hz, H-8’), 7.79 (1H, d, J = 15.7 Hz, H-1”), 7.70 (1H, ddd, J = 0.9, 7.0 and 8.1 Hz, H-7’), 7.61 (2H, m, H-4 and H-6’), 7.54 (1H, d, J = 5.7 Hz, H-4’), 7.21 (1H, d, J = 8.3 Hz, H-7), 7.15 (1H, ddd, J = 1.1, 7.0 and 8.3 Hz, H-6), 7.09 (1H, m, H-5), 7.08 (1H, s, H-3), 6.46 (1H, d, J = 15.7 Hz, H-2”), 6.00 (2H, s, H-3”), 3.72 (3H, s, Me). δC (100.6 MHz, CDCl3): 167.2, 154.4, 142.0, 139.0, 136.1, 135.4, 133.2, 130.1, 127.7, 127.6, 127.6, 125.7, 123.6, 123.2, 121.5, 120.6, 120.5, 117.8, 109.9, 104.0, 51.5, 46.7. m/z (ESI+) calc. for [M+Na+]: 365.1260, found 365.1259. Characterisation details for compound 5: Mp = 153-156 °C. λmax (MeOH)/nm 340, 322, 258, 227 (ε/dm3 mol-1 cm-1 13 700, 13 700, 17 200, 33 700); νmax/cm-1: 3097, 2943, 1711, 1622, 1578, 1505, 897, 820, 736. 1H NMR (400 MHz, CDCl3) δ: 8.39 (1H, d, J = 5.7 Hz, H-3’), 8.13 (1H, d, J = 8.4 Hz, H-5’), 7.95 (1H, d, J = 8.2 Hz, H-8’), 7.80 (1H, m, H-7’), 7.72 (1H, m, H-6’), 7.70 (1H, d, J = 15.8 Hz, H-1*), 7.70 (1H, dd, J = 0.7 and 8.0 Hz, H-4), 7.64 (1H, d, J = 15.6 Hz, H-1”), 7.62 (1H, d, J = 5.7 Hz, H-4’), 7.22 (1H, d, J = 6.8 Hz, H-6), 7.13 (1H, s, H-3), 7.11 (1H, m, H-5), 6.47 (1H, d, J = 15.7 Hz, H-2”), 6.17 (2H, s, H-3”), 6.09 (1H, d, J = 15.6 Hz, H-2*), 3.72 (3H, s, H-Me), 3.18 (3H, s, H-Me*). δC (100.6 MHz, CDCl3) 167.1, 166.2, 154.5, 142.4, 142.3, 137.1, 137.0, 136.2, 132.9, 130.4, 129.0, 127.8, 127.7, 125.2, 124.1, 123.6, 123.2, 120.7, 120.5, 120.2, 119.1, 109.5, 104.8, 51.7, 51.1, 48.8. m/z (ESI+) calc. for [M+Na+]: 449.1472, found 449.1460.
12. Deposited with CDS as CCDC 745057.
13. V. Rukachaisirikul and R. W. Hoffmann, Tetrahedron, 1992, 48, 10563. CrossRef
14. N. Charrier, E. Demont, R. Dunsdon, G. Maile, A. Naylor, A. O'Brien, S. Redshaw, P. Theobald, D. Vesey, and D. Walter, Synthesis, 2006, 3467; CrossRef C. G. Hartung, A. Fecher, B. Chapell, and V. Snieckus, Org. Lett., 2003, 5, 1899; CrossRef S. Paul, G. A. Chotana, D. Holmes, R. C. Reichle, R. E. Maleczka, and M. R. Smith, J. Am. Chem. Soc., 2006, 128, 15552; CrossRef A. K. Mohanakrishnan, R. Balamurugan, N. Ramesh, M. Mathiselvam, and S. Manavalan, Synth. Commun., 2007, 37, 4343. CrossRef
15. A. R. Katritzky and K. Akutagawa, Tetrahedron Lett., 1985, 26, 5935. CrossRef
16. S. Hibino, E. Sugino, T. Kuwada, N. Ogura, K. Sato, and T. Choshi, J. Org. Chem., 1992, 57, 5917. CrossRef
17. W. Rauf and J. M. Brown, Chem. Commun., 2009, 3874; CrossRef W. Rauf, A. P. Thompson, and J. M. Brown, Synlett, in press.
18. T. A. Halgren, J. Comput. Chem., 1996, 17, 490; computation carried out with Spartan08.