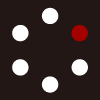
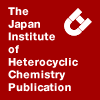
HETEROCYCLES
An International Journal for Reviews and Communications in Heterocyclic ChemistryWeb Edition ISSN: 1881-0942
Published online by The Japan Institute of Heterocyclic Chemistry
e-Journal
Full Text HTML
Received, 3rd September, 2009, Accepted, 15th October, 2009, Published online, 16th October, 2009.
DOI: 10.3987/COM-09-S(S)123
■ Synthesis of Chiral 1,3-Disubstituted Tetrahydroisoquinolines and Their Use in the Asymmetric Addition of Diethylzinc to Aldehydes
Patricia D. MacLeod, Amy M. Reckling, and Chao-Jun Li*
Department of Chemistry, McGill University, 801 Sherbrooke St. West, Montreal, Quebec H3A 2K6, Canada
Abstract
The effects of modification on three sites of 1-(1,2,3,4-tetrahydro-isoquinolin-1-yl)-naphthalen-2-ol (THIQNOL) chiral ligands on the asymmetric diethylzinc additions were examined. The studies showed that modifications at the nitrogen only reduces the efficiency of these types of ligands, whereas modification at the 3-position of the 1,2,3,4-tetrahydroisoquinoline ring and the 3-position of the naphthol ring can lead to chiral ligands which provide better enantioselectivities. In general, the use of a simple phenyl group on the 1,2,3,4-tetrahydroisoquinoline ring and either a phenyl or methoxyphenyl on the naphthol ring generates more effective chiral ligands for the asymmetric addition of diethylzinc to aldehydes.INTRODUCTION
The discovery of novel enantioselective reactions to generate optically active compounds plays a fundamental role in pharmaceutical research and represents one of the most important developments in modern organic chemistry.1 Many organic compounds of interest for pharmaceuticals and pesticides are chiral and very often only one of the enantiomers is effective or desirable for biological purposes. Because of this, the FDA now requires chiral drugs to be marketed in enantiomerically pure form. Asymmetric catalysis, which uses chiral catalysts to generate chiral compounds, is at the forefront of methodologies for achieving this challenge.2 Among the many chiral catalysts developed, BINAP-,3 QUINAP-4 and BINOL-based5 chiral complexes have been shown to be especially effective in catalyzing asymmetric reactions.
Of these types of reactions, the asymmetric addition of alkylzinc reagents to aldehydes in the presence of catalytic amounts of chiral catalysts has garnered much attention in recent years.6 Different types of ligands, including β-amino alcohols, amino thiols, amines and diols, have been employed to achieve asymmetric inductions; however Betti-base type derivatives, aminoalkylnapthols first synthesized by Betti in 1900,7 have also been shown to be effective chiral ligands.8 The Betti base and its derivatives generally acquire their chirality from chiral centres on the molecule, whereas the more popular QUINAP-, BINAP- and BINOL-based ligands possess a single chiral axis. Recently, we have synthesized a new class of chiral compounds9 which have both a chiral centre and a chiral axis that exist in close proximity. Such compounds can provide chiral match or mismatch effects, which can potentially enhance the asymmetric inductions in catalysis. We use the asymmetric diethylzinc addition as a proof-of-concept evaluation of such new chiral ligands. These compounds also have the potential to provide N,O; N,P and N,S ligands and are easily synthesized by the direct aza-Friedel-Crafts addition. The subsequent methylation and resolution of our lead-compound generated a representative chiral ligand 1-(1,2,3,4-tetrahydro-isoquinolin-1-yl)-naphthalen-2-ol (THIQNOL), (S)-(-)-1a, which was used to achieve 69% ee in the addition of diethylzinc to benzaldehyde (Scheme 1).9c In this report, we wish to communicate the preparation of new chiral ligands based on our initial design and their activity towards the asymmetric addition of diethylzinc to aldehydes.
RESULTS AND DISCUSSION
While seeking out modifications to improve on the enantioselectivities we achieved using (S)-1a, we had initially focused our modifications on the N-substituent. The methyl group was thus replaced by ethyl, propyl, allyl or benzyl groups (1b-e). Using dibenzoyl L-tartaric acid (L-DBTA), we were then able to resolve the ligands 1b-1d (Table 1). Unfortunately, we were unable to resolve ligand 1e; therefore, we separated the enantiomers by chiral HPLC. With the optically pure ligands 1b-1e in hand, we then examined their effectiveness in the asymmetric diethylzinc addition under the standard conditions. These modifications however, only resulted in decreasing rather than increasing the ee for the addition of diethylzinc to benzaldehyde (Table 2).
With N-substitutions not giving us the increase in enantioselectivity that we desired, we decided to look at alternative places to make modifications. We initially modified the 3-position of the tetrahydroisoquinoline ring; firstly, because literature has shown10 that (R)-3-phenyl-3,4-dihydroisoquinoline 3 could be formed with high enantiopurity from (±)-1,2-diphenylethylamine and secondly, because it is known that fixing that chiral centre will fix the chiral centre at position 1.11
Compound (S)-(R)-1f was isolated in moderate yield by direct addition of 2-naphthol to (R)-3-phenyl-3,4-dihydroisoquinoline 3 using our methodology developed previously (Table 4, Entry 1). To our delight, the application of this structure to the asymmetric addition of diethylzinc to benzaldehyde yielded the product in 79% ee (Table 5, Entry 1). With this encouraging result in hand, we decided to further modify the structure by installing a substituent at the 3-position of the naphthol ring. To this end, we prepared various 3-substituted 2-naphthols according to the rhodium-catalyzed procedure of Oi and co-workers (Table 3).12 We were then able to isolate the new chiral ligands in moderate yields through the addition of the 3-substituted 2-naphthols to 3 (Table 4). To confirm the relative configurations of C-1 and C-3, we performed nOe measurements on (S)-(R)-1g.
These new chiral ligands were then used to catalyze the diethylzinc addition to aldehydes (Table 5).
As mentioned previously, ligand (S)-(R)-1f yielded the diethylzinc addition product with better ee (79%) than we had seen with our previous modifications at the N position (Table 5, Entry 1). The addition of a moiety on the 3-position of the naphthol ring initially led to a further increase in ee, up to 92% for a simple phenyl group (Table 5, Entry 2). This encouraging result led us to use ligand (S)-(R)-1g as the chiral catalyst for the diethylzinc addition to a variety of aldehydes. In general, the ee’s for these substrates were high, up to 97% for the 4-methylbenzaldehyde (Table 5, Entry 3). On the other hand, it seems that there is a threshold for the amount of bulk on the added moiety that the system can sustain.
This is exemplified by comparing the use of ligand (S)-(R)-1h and -1i. The use of the methoxy group in the 3- or 4-position (Table 5, Entries 11 and 12) with these types of ligands keeps the enantioselectivity in the same vicinity as the results given by (S)-(R)-1g (Table 5, Entry 2), though the 4-methoxy substituted compound (S)-(R)-1h is slightly better. Once there is too much bulk added to the ligand, in this case, in the form of the 3-naphthyl substituted naphthol (S)-(R)-1k and the 3-(3,5-di-tert-butyl phenyl) 3-substituted naphthol (S)-(R)-1j, the reaction is less effective and gives much lower enantioselectivities (Table 5, Entries 13 and 14). Interestingly, when a third coordination site is added by replacing that same aromatic ring by an aliphatic alcohol, (S)-(R)-1l, the enantioselectivity is once again reduced (Table 5, Entry 15), showing that an extra coordination site is not beneficial to the reaction. This can be rationalized by visualizing the molecule. As seen in Figure 1, the addition of the second hydroxyl group only gives the zinc an opportunity to coordinate further away from the chiral atmosphere generated by the ligand.
In summary, we have studied the effects of modifications on three sites of our reported THIQNOL chiral ligands on the asymmetric diethylzinc additions. The studies show that modifications at the nitrogen only reduce the efficiency of these types of ligands, whereas modifications at the 3-position of the 1,2,3,4-tetrahydroisoquinoline ring and the 3-position of the naphthol ring can lead to chiral ligands which provide better enantioselectivities. However, it must be noted that there is a threshold for the amount of bulk that can be added on the naphthol ring before the chiral ligand becomes less effective. In general, the use of a simple phenyl group on the 1,2,3,4-tetrahydroisoquinoline ring and either a phenyl or methoxyphenyl on the naphthol ring generates more effective chiral ligands for the asymmetric addition of diethylzinc to aldehydes. With the knowledge gained from this study, we are provided the opportunity to further investigate the synthesis of structurally similar molecules in an effort to generate even more effective ligands. The application of these chiral ligands in other asymmetric reactions is currently under investigation.
EXPERIMENTAL
General
1H NMR spectra were recorded on Varian 300, 400 and 500 MHz spectrometers and the chemical shifts were reported in parts per million (δ) relative to internal standard TMS (0 ppm) for CDCl3. The peak patterns are indicated as follows: s, singlet; brs, broad singlet; d, doublet; t, triplet; dt, doublet of triplet; dq, doublet of quartet; ddd, doublet of doublet of doublet; dtd, doublet of triplet of doublet; m, multiplet; q, quartet; qn, quintet. The coupling constants, J, are reported in Hertz (Hz). 13C NMR spectra were obtained at 75, 100, and 125 MHz spectrometers and referenced to the internal solvent signals (central peak is 77.0 ppm in CDCl3). All reagents were purchased from Aldrich. All reagents were used without further purification. 1-(1,2,3,4-Tetrahydro-isoquinolin-1-yl)-naphthalen-2-ol was prepared according to our previous work. 9b,c 3-Phenyl-3,4-dihydroisoquinoline and 3-(hydroxymethyl)naphthalen-2-ol were prepared according to literature methodology.10,13
1-(2-Ethyl-1,2,3,4-tetrahydroisoquinolin-1-yl)naphthalen-2-ol (1b): To a solution of 1-(1,2,3,4-tetrahydro-isoquinolin-1-yl)naphthalen-2-ol (0.550 g, 2.0 mmol) in DMF (17 mL), powdered KHCO3 (0.204 g, 2.1 mmol) was added. The resulting slurry was stirred at room temperature for 10 minutes, then iodoethane (0.9 mL, 5.0 mmol) in DMF (12 mL) was added dropwise to the mixture. After stirring for 22 h, CH2Cl2 was added to the reaction mixture and the solution was washed with water to remove DMF. The organic layer was dried over MgSO4 and the solvent removed under reduced pressure to yield the crude product. Flash chromatography (hexanes/EtOAc = 10:1, 5:1) yielded a white powder (0.503 g, 1.7 mmol, 83 %). mp 125-128 oC; IR (solid): νmax 3022, 2952, 2869, 1620, 1597, 1462, 1412, 1268, 1234, 818, 742, 702; 1H NMR (CDCl3, 500MHz, ppm) δ 12.08 (s, 1H), 8.09 (d, J = 8.4 Hz, 1H), 7.82 (d, J = 8.4 Hz, 1H), 7.71 (d, J = 9.2 Hz, 1H), 7.53 (dt, J = 8.4, 1.2 Hz, 1H), 7.35 (t, J = 7.6 Hz, 1H), 7.13-7.05 (m, 3H), 6.86 (t, J = 7.6 Hz, 1H), 6.65 (d, J = 8.0 Hz, 1H), 5.57 (s, 1H), 3.0 (ddd, J = 11.2, 5.6, 1.6 Hz, 1H), 3.39-3.31 (m, 1H), 2.74-2.84 (m, 2H), 2.71 (dt, J = 12.0, 3.6 Hz, 1H), 2.45 (m,1H), 1.11 (t, J = 7.2 Hz, 3H); 13C NMR (CDCl3, 125 MHz, ppm) δ 155.6, 136.3, 134.1, 133.7, 129.4, 128.9, 128.4, 128.1, 127.5, 126.9, 126.4, 126.2, 122.4, 121.4, 119.9, 118.0, 61.8, 48.6, 48.2, 29.5, 11.4; HRMS (EI): calculated for C21H21NO: [M+•] = 303.1623 m/z; found: [M+•] 303.1614 m/z.
Resolution: To a solution of 1 (1.847g, 6.1 mmol) in CH2Cl2 (8 mL), a solution of L-dibenzoyltartaric acid (2.186 g, 6.1 mmol) in 100% EtOH (34 mL) was added dropwise. The resulting mixture was stirred for 22 hours which led to the formation of a colorless salt. The solid was filtered, suspended in water and treated with aqueous Na2CO3. After extraction with CH2Cl2, the organic phase was dried over MgSO4 and the solvent was removed under reduced pressure to yield a light purple powder (0.813 g, 2.7 mmol, 44 %). The enantiomeric purity was determined by chiral HPLC (Daicel Chiralcel OD-H, hexane/isopropanol = 97.5 2.5, flow rate 0.5 mL/min) to be 90 %. The mother liquor was evaporated under reduced pressure and subjected to the same measures as above. A pale orange solid (0.100 g, 3.3 mmol, 54 %) was isolated and its enantiomeric purity was determined to be 89 %. After one recrystallization from Et2O, a white solid (0.138 g, 0.46 mmol, 7%) was collected and the enantiomeric purity was found to be 98 %, [α]D20 +315.0 (c 0.168, CH2Cl2). A second recrystallization was performed on the remaining solution and a colorless, crystalline material (0.380 g, 1.3 mmol, 21 %) was isolated. The enantiomeric purity was determined to be >99.5 %.
1-(2-Propyl-1,2,3,4-tetrahydroisoquinolin-1-yl)naphthalen-2-ol (1c): To a solution of 1-(1,2,3,4-tetrahydroisoquinolin-1-yl)naphthalen-2-ol (1.651 g, 6.0 mmol) in DMF (30 mL), powdered KHCO3 (0.661 g, 6.6 mmol) was added. The resulting slurry was stirred at room temperature for 10 minutes, then 1-iodopropane (2.4 mL, 30 mmol) in DMF (10 mL) was added dropwise to the mixture. After stirring for 24 h, CH2Cl2 was added to the reaction mixture and the solution was washed with water to remove DMF. The organic layer was dried over MgSO4 and the solvent removed under reduced pressure to yield the crude product. Flash chromatography (hexanes/EtOAc = 10:1, 5:1) yielded a white powder (1.252 g, 4.0 mmol, 66 %). mp 117-118 oC; IR (solid): νmax 2952, 2923, 2876, 1620, 1598, 1462, 1268, 814, 742, 702; 1H NMR (CDCl3, 400MHz, ppm) δ 12.01 (s, 1H), 8.07 (d, J = 8.8 Hz, 1H), 7.77 (d, J = 8.0 Hz, 1H), 7.67 (d, J = 9.2 Hz, 1H), 7.49 (t, J = 7.2 Hz, 1H), 7.30 (t, J = 8.0 Hz, 1H), 7.08-7.00 (m, 3H), 6.80 (t, J = 7.2 Hz, 1H), 6.63 (d, J = 8.0 Hz, 1H), 5.50 (s, 1H), 3.44 (dd, J = 11.2, 3.6 Hz, 1H), 3.34-3.27 (m, 1H), 2.85 (d, J = 16.4 Hz, 1H), 2.73-2.66 (m, 1H), 2.59 (t, J = 12.0 Hz, 1H), 2.33-2.26 (m, 1H), 1.55-1.51 (m, 2H), 0.74 (t, J = 7.2 Hz, 3H); 13C NMR (CDCl3, 125 MHz, ppm) δ 155.4, 136.3, 134.1, 133.6, 129.3, 128.9, 128.4, 128.0, 127.4, 126.8, 126.3, 126.1, 122.4, 121.3, 119.8, 118.2, 62.4, 56.6, 48.7, 29.4, 19.5, 11.5; HRMS (EI): calculated for C22H23NO: [M+•] = 317.1780 m/z; found: [M+•] 317.1774 m/z.
Resolution: To a solution of 2 (0.317g, 1.0 mmol) in CH2Cl2 (1.3 mL), a solution of L-dibenzoyltartaric acid (0.358 g, 1.0 mmol) in 100% EtOH (6.0 mL) was added dropwise. The resulting mixture was stirred for 20 h which led to the formation of a colorless salt. The solid was filtered, suspended in water and treated with aqueous Na2CO3. After extraction with CH2Cl2, the organic phase was dried over MgSO4 and the solvent was removed under reduced pressure to yield light purple powder (0.117 g, 0.4 mmol, 40 %). The enantiomeric purity was determined by chiral HPLC (Daicel Chiralcel OD-H, hexane/isopropanol = 97.5 2.5, flow rate 0.5 mL/min) to be 95 %, with optical rotation [α]D20 -283.2 (c 0.175, CH2Cl2). The mother liquor was evaporated under reduced pressure and subjected to the same measures as above. A pale orange solid (0.197 g, 0.6 mmol, 60 %) was isolated and its enantiomeric purity was determined to be 48 %.
1-(2-Allyl-1,2,3,4-tetrahydroisoquinolin-1-yl)naphthalen-2-ol (1d): To a solution of 1-(1,2,3,4-tetrahydro-isoquinolin-1-yl)naphthalen-2-ol (1.376g, 5.0 mmol) in DMF (16 mL), powdered KHCO3 (0.510 g, 5.1 mmol) was added. The resulting slurry was stirred at room temperature for 10 min, then allyl bromide (0.43 mL, 5.0 mmol) was added dropwise to the mixture. After stirring for 23 h, CH2Cl2 was added to the reaction mixture and water was used to wash off the DMF. The organic layer was dried over MgSO4, filtered and the solvent removed under reduced pressure. Flash chromatography (hexanes/EtOAc = 3:1) yielded a fluffy white powder (1.334 g, 4.2 mmol, 85 %). mp 104-108 oC; IR (solid): νmax 2926, 2843, 1620, 1597, 1468, 1409, 1273, 1237, 935, 821, 746; 1H NMR (CDCl3, 500MHz, ppm) δ 11.84 (s, 1H), 8.08 (d, J = 8.5 Hz, 1H), 7.82 (d, J = 8.0 Hz, 1H), 7.55-7.52 (m, 1H), 7.37-7.34 (m, 1H), 7.13-7.05 (m, 3H), 6.86 (t, J = 7.5 Hz, 1H), 6.64 (d, J = 8.0 Hz, 1H), 5.92-5.84 (m, 1H), 5.58 (s, 1H), 5.21 (dd, J = 10.0, 1.0 Hz, 1H), 5.12 (d, J = 17.0 Hz, 1H), 3.53-3.49 (m, 2H), 3.37-3.30 (m, 1H), 2.95-2.90 (m, 2H), 2.72-2.66 (m, 1H); 13C NMR (CDCl3, 125 MHz, ppm) δ 155.4, 136.1, 134.1, 133.7, 133.3, 129.6, 128.9, 128.5, 128.2, 127.6, 126.9, 126.5, 126.3, 122.5, 121.5, 119.8, 119.6, 117.7, 61.8, 57.6, 48.7, 29.4; HRMS (EI): calculated for C22H21NO: [M+•] = 315.1623 m/z; found: [M+•] 315.1616 m/z.
Resolution: To a solution of 13 (1.318 g, 4.2 mmol) in CH2Cl2 (5mL), a solution of di-benzoyl tartaric acid (1.497 g, 4.2 mmol) in 100% EtOH (10 mL) was added dropwise. The resulting mixture was stirred for 25 h with the formation of a colorless salt happening immediately after the addition of DBTA. The solid was filtered, suspended in water and treated with aqueous Na2CO3. After extraction with CH2Cl2, the organic phase was dried over MgSO4 and the solvent was removed under reduced pressure to yield a white powder (0.568 g, 1.8 mmol, 43 %). The enantiomeric purity was determined by chiral HPLC (Daicel Chiralcel AD hexane/isopropanol = 99:1, flow rate 1.0 mL/min) to be 92 %, with optical rotation [α]D20 -260.3 (c 0.180, CH2Cl2). The mother liquor was evaporated under reduced pressure and subjected to the same measures as above. A yellow solid was isolated and its enantiomeric purity was determined to be 90%.
1-(2-Benzyl-1,2,3,4-tetrahydroisoquinolin-1-yl)naphthalen-2-ol (1e): To a solution of 1-(1,2,3,4-tetrahydro-isoquinolin-1-yl)naphthalen-2-ol (1.158 g, 4.2 mmol) in MeCN (40 mL), powdered KHCO3 (0.0.429 g, 4.3 mmol) was added. The resulting slurry was stirred at room temperature for 10 min, then benzyl bromide (0.55 mL, 4.6 mmol) in MeCN (12 mL) was added dropwise to the mixture. After stirring for 3 days, the solvent was removed under reduced pressure and flash chromatography was done with hexanes/EtOAc (20:1, 10:1) to yield a pale yellow powder (1.313 g, 3.6 mmol, 86 %). mp 145-149 oC; IR (solid): νmax 2834, 1621, 1453, 1265, 814, 742, 699; 1H NMR (CDCl3, 400MHz, ppm) δ 11.86 (s, 1H), 8.10 (d, J = 8.4 Hz, 1H), 7.79 (d, J = 7.6 Hz, 1H), 7.71 (d, J = 9.2 Hz, 1H), 7.52 (t, J = 6.8 Hz, 1H), 7.33 (t, J = 7.2 Hz, 1H), 7.28-7.21 (m, 5H), 7.14 (d, J = 8.8 Hz, 1H), 7.02 (m, 2H), 6.80-6.78 (m, 1H), 6.67 (d, J = 8.0 Hz, 1H), 5.57 (s, 1H), 4.07 (d, J = 12.8 Hz, 1H), 3.30 (dd, J = 10.8, 4.8 Hz, 1H), 3.23-3.11 (m, 2H), 2.74 (d, J = 16.8 Hz, 1H), 2.53 (dt, J = 11.6, 2.8 Hz, 1H); 13C NMR (CDCl3, 125 MHz, ppm) δ 155.1, 136.5, 135.9, 133.7, 129.64, 129.60 (2C), 129.0, 128.5, 128.4 (2C), 128.1, 127.5, 127.4, 127.0, 126.6, 126.2, 122.6, 121.5, 119.9, 118.1, 62.5, 59.5, 48.4, 29.2.
Resolution: Separation by chiral semi-prep HPLC (Daicel Chiralcel OD column, hexanes/isopropanol = 97.5:2.5, flow rate 3.0 mL/min) [α]D20+ 174.4 (c 0.219, CH2Cl2) (second peak).
General Procedure for Synthesis of 3-Substituted 2-Naphthols12:
Unless otherwise noted, [Rh(cod)Cl)2 (12.5 mg, 0.025 mmol), K2CO3 (276 mg, 2 mmol), Cs2CO3 (652 mg, 2 mmol) and 2-naphthol (144 mg, 1 mmol) were place in a sealable tube and placed under nitrogen atmosphere. Dry toluene (2 mL) was then added, followed by HMPT (36 µL, 0.2 mmol) and aryl bromide (2.4 mmol). The mixture was stirred vigorously at 100oC for 20 h. Upon cooling to room temperature, 10 mL conc HCl and 10 mL Et2O were added and the mixture stirred vigorously for 1 h. The mixture was then extracted with Et2O three times and the organic extracts washed with brine. After drying over MgSO4, the solvent was removed to yield the crude reaction mixture. Flash chromatography on silica gel yielded the corresponding products.
3-Phenylnaphthalen-2-ol (4b): Isolated by flash chromatography (hexanes/EtOAc = 9:1) to give a beige powder (119 mg, 0.54 mmol, 54 %). 1H NMR (CDCl3, 400 MHz, ppm) δ 7.79-7.72 (m, 3H), 7.56-7.42 (m, 6H), 7.36-7.33 (m, 2H), 5.26 (brs, 1H). This is a known compound and the spectral data is consistent with those reported in literature.14
3-(4-Methoxyphenyl)naphthalen-2-ol (4c): Isolated by flash chromatography (hexanes/EtOAc = 10:1) to give a white powder (91 mg, 0.36 mmol, 36 %). 1H NMR (CDCl3, 500MHz, ppm) δ 7.76 (d, J = 8.0 Hz, 1H), 7.72-7.70 (m, 2H), 7.49 (d, J = 8.5 Hz, 2H), 7.42 (t, J = 7.5 Hz, 1H), 7.34-7.31 (m, 2H), 7.05 (d, J = 9.0 Hz, 2H), 5.25 (s, 1H), 3.88 (s, 3H). This is a known compound and the spectral data is consistent with those reported in literature.15
3-(3-Methoxyphenyl)naphthalen-2-ol (4d): Isolated by flash chromatography (hexanes/EtOAc = 20:1) to give an orange oil (108 mg, 0.43 mmol, 43 %). IR (solid): νmax 3511, 3052, 3005, 2900, 2833, 1628, 1598, 1505, 1386, 1243, 1163, 1032, 811, 741; 1H NMR (CDCl3, 500MHz, ppm) δ 7.75 (d, J = 8.5 Hz, 1H), 7.72 (s, 1H), 7.69 (d, J = 8.0 Hz, 1H), 7.43-7.39 (m, 2H), 7.33-7.30 (m, 2H), 7.12 (d, J = 8.0 Hz, 1H), 7.08 (s, 1H), 6.96 (dd, J = 8.5, 2.0 Hz, 1H), 5.49 (s, 1H), 3.83 (s, 3H); 13C NMR (CDCl3, 125 MHz, ppm) δ 160.2, 150.8, 138.2, 134.3, 130.3, 130.3, 129.3, 128.8, 127.7, 126.5, 126.2, 123.8, 121.4, 114.8, 113.8, 110.2, 55.3.
3-(3,5-Di-tert-butylphenyl)naphthalen-2-ol (4e): Isolated by flash chromatography (hexanes/EtOAc = 9:1) to yield an orange oil (33 mg, 0.1 mmol, 20 %). 1H NMR (CDCl3, 500MHz, ppm) δ 7.78 (d, J = 8.0 Hz, 1H), 7.74-7.72 (m, 2H), 7.52-7.52 (m, 1H), 7.44-7.41 (m, 1H), 7.37-7.31 (m, 4H), 5.40 (s, 1H), 1.38 (s, 16H); 13C NMR (CDCl3, 125 MHz, ppm) δ 152.1, 150.9, 135.8, 134.3, 131.4, 129.2, 128.9, 127.7, 126.3, 126.3, 123.8, 123.4, 122.4, 110.0, 35.1, 31.5.
3-(Naphthalen-2-yl)naphthalen-2-ol (4f): Isolated by flash chromatography (hexanes/EtOAc = 9:1) to give a white powder (129 mg, 0.48 mmol, 48 %). mp 116-118 oC; IR (solid): νmax 3518, 3050, 2922, 1628, 1595, 1515, 1357, 1160, 826, 741; 1H NMR (CDCl3, 500MHz, ppm) δ 8.04 (s, 1H), 8.00 (d, J = 8.0 Hz, 1H), 7.93-7.90 (m, 2H), 7.84 (s, 1H), 7.81 (d, J = 8.5 Hz, 1H), 7.75 (d, J = 8.5 Hz, 1H), 7.68 (dd, J = 8.0, 1.5 Hz, 1H), 7.47-7.54 (m, 2H), 7.47-7.44 (m, 1H), 7.37-7.34 (m, 1H), 7.25 (s, 1H), 5.36 (s, 1H); 13C NMR (CDCl3, 125 MHz, ppm) δ 150.9, 134.4, 134.3, 133.6, 132.9, 130.4, 129.8, 129.0, 129.0, 128.2, 128.1, 127.8, 127.8, 127.2, 126.7, 126.6, 126.3, 123.9, 110.3; HRMS (EI): calculated for C20H14O: [M+•] = 270.1044 m/z; found: [M+•] 270.1037 m/z.
General Procedure for Addition of 3-Substituted 2-Naphthols to 3-Phenyl-3,4-dihydroisoquinoline:
Unless otherwise noted, 3-phenyl-3,4-dihydroisoquinoline (0.2 mmol) and the corresponding naphthol (0.2 mmol) were placed under nitrogen atmosphere and degassed water (0.4 mL) was added to the capped vessel. The reaction mixture was heated to 80 oC and stirred overnight. After extraction with CH2Cl2, the solvent was removed and the crude mixture purified by flash chromatography on silica gel.
1-((1S,3R)-3-Phenyl-1,2,3,4-tetrahydroisoquinolin-1-yl)naphthalen-2-ol (1f): Addition of 2-naphthol (1 mmol) to 3-phenyl-3,4-dihydroisoquinoline (1 mmol). Filtering and washing with Et2O yields a beige powder (0.264 g, 0.75 mmol, 75 %). mp 150-152 oC; IR (solid): νmax 3280, 3022, 2926, 1622, 1598, 1453, 1343, 1266, 1235, 1010, 812, 739, 700; 1H NMR (CDCl3, 500MHz, ppm) δ 11.80 (s, 1H), 8.04 (d, J = 8.5 Hz, 1H), 7.79 (d, J = 8.0 Hz, 1H), 7.71 (d, J = 8.5 Hz, 1H), 7.51 (t, J = 7.5 Hz, 1H), 7.44 (d, J = 7.0 Hz, 2H), 7.38-7.29 (m, 4H), 7.11-7.09 (m, 3H), 6.91-6.89 (m, 1H), 6.67 (d, J = 8.0 Hz, 1H), 6.23 (s, 1H), 4.28 (dd, J = 11.0, 3.0 Hz, 1H), 3.31-3.25 (m, 2H), 3.09 (dd, J = 16.5, 3.0 Hz, 1H); 13C NMR (CDCl3, 125 MHz, ppm) δ 155.8, 142.7, 135.7, 134.1, 133.5, 123.0, 128.97 (2C), 128.64, 128.6, 128.5, 128.2, 127.1, 127.0, 126.9, 126.6 (2C), 122.7, 121.5, 120.2, 118.2, 59.4, 56.8, 38.5; HRMS (EI): calculated for C25H21NO: [M+•] = 351.1623 m/z; found: [M+•] 351.1616 m/z.
3-Phenyl-1-((1S,3R)-3-phenyl-1,2,3,4-tetrahydroisoquinolin-1-yl)naphthalen-2-ol (1g): Addition of 3-phenyl-2-naphthol (1.2 mmol) to 3-phenyl-3,4-dihydroisoquinoline (1.2 mmol). Filtering and washing with cold Et2O, then drying for 3 h in a 60 oC oven yields a white powder (0.242 g, 0.56 mmol, 48 %). mp 129-132 oC; IR (solid): νmax 3279, 3057, 3029, 2920, 1602, 1492, 1453, 1423, 1012, 745, 698; 1H NMR (CDCl3, 500MHz, ppm) δ 12.10 (s, 1H), 8.09 (d, J = 8.5 Hz, 1H), 7.85 (d, J = 8.0 Hz, 1H), 7.80 (s, 1H), 7.62 (d, J = 7.0 Hz, 2H), 7.53 (t, J = 7.0 Hz, 1H), 7.49 (d, J = 7.0 Hz, 2H), 7.43-7.31 (m, 7H), 7.17-7.13 (m, 2H), 6.98-6.95 (m, 1H), 6.76 (d, J = 8.0 Hz, 1H), 6.37 (s, 1H), 4.36 (dd, J = 11.5, 3.5 Hz, 1H), 3.37-3.32 (m, 2H), 3.13 (dd, J = 16.5, 3.5 Hz, 1H), 2.84 (s, 1H); 13C NMR (CDCl3, 125 MHz, ppm) δ 153.8, 142.5, 128.4, 135.7, 134.2, 133.0, 132.6, 130.2, 129.7 (2C), 129.0, 128.9 (2C), 128.6, 128.2, 128.1, 128.0 (2C), 127.2, 127.0, 126.94, 126.88, 126.6 (2C), 126.5, 123.0, 121.2, 118.4, 59.6, 57.1, 38.3.
3-(4-Methoxyphenyl)-1-((1S,3R)-3-phenyl-1,2,3,4-tetrahydroisoquinolin-1-yl)naphthalen-2-ol (1h): Flash chromatography (CH2Cl2/hexanes = 3:1) yields a white powder (72 mg, 0.16 mmol, 69 %). mp 146-150 oC; IR (solid): νmax 3279, 2853, 1600, 1509, 1451, 1418, 1241, 1166, 1153, 1011, 840, 756, 745, 701; 1H NMR (CDCl3, 500MHz, ppm) δ 12.13 (s, 1H), 8.03 (d, J = 8.5 Hz, 1H), 7.80 (d, J = 8.0 Hz, 1H), 7.74 (s, 1H), 7.54 (d, J = 9.0 Hz, 2H), 7.48 (t, J = 8.0 Hz, 1H), 7.43 (d, J = 7.0 Hz, 2H), 7.35-7.27 (m, 4H), 7.12-7.07 (m, 2H), 6.93-6.91 (m, 3H), 6.73 (d, J = 8.0 Hz, 1H), 6.29 (s, 1H), 4.28 (dd, J = 11.5, 3.5 Hz, 1H), 3.80 (s, 3H), 3.31-3.26 (m, 1H), 3.07 (dd, J = 16.5, 3.5 Hz, 1H), 2.73 (s, 1H); 13C NMR (CDCl3, 125 MHz, ppm) δ 158.8, 153.9, 142.5, 135.7, 134.1, 132.7, 132.1, 130.75 (2C), 130.71, 129.6, 128.9 (2C), 128.8, 128.5, 128.2, 128.1, 126.9, 126.8, 126.7, 126.6 (2C), 126.4, 122.9, 121.1, 118.4, 113.5 (2C), 59.4, 57.0, 55.2, 38.3; HRMS (EI): calculated for C32H27NO2: [M+•] = 457.2042 m/z; found: [M+•] 457.2047 m/z.
3-(3-Methoxyphenyl)-1-((1S,3R)-3-phenyl-1,2,3,4-tetrahydroisoquinolin-1-yl)naphthalen-2-ol (1i): Flash chromatography (CH2Cl2/hexanes = 3:1) yields a white powder (46 mg, 0.10 mmol, 48 %). Mp 134-139 oC; IR (solid): νmax 3282, 3027, 2924, 2832, 1599, 1489, 1453, 1417, 1262, 1043, 1012, 744, 726, 699; 1H NMR (CDCl3, 500MHz, ppm) δ 12.1 (s, 1H), 8.05 (d, J = 8.5 Hz, 1H), 7.82 (d, J = 8.0 Hz, 1H), 7.78 (s, 1H), 7.51 (t, J = 7.5 Hz, 1H), 7.45 (d, J = 7.5 Hz, 2H), 7.37-7.28 (m, 5H), 7.22-7.09 (m, 4H), 6.93 (t, J = 8.0 Hz, 1H), 6.87 (dd, J = 8.5, 2.0 Hz, 1H), 6.74 (d, J = 8.0 Hz, 1H), 6.32 (s, 1H), 4.31 (dd, J = 11.5, 3.5 Hz, 1H), 3.81 (s, 3H), 3.33-3.27 (m, 1H), 3.08 (dd, J = 16.5, 3.0 Hz, 1H), 2.77 (s, 1H); 13C NMR (CDCl3, 125 MHz, ppm) δ 159.2, 153.8, 142.4, 139.7, 135.7, 134.1, 133.0, 132.4, 130.1 (2C), 129.0, 128.9 (2C), 128.5, 128.11, 128.07, 127.0, 126.9, 126.8, 126.6 (2C), 126.5, 122.9, 122.2, 121.2, 118.4, 115.5, 112.6, 59.5, 57.1, 55.3, 38.3; HRMS (EI): calculated for C32H27NO2: [M+•] = 457.2042 m/z; found: [M+•] 457.2030 m/z.
3-(3,5-Di-tert-butylphenyl)-1-((1S,3R)-3-phenyl-1,2,3,4-tetrahydroisoquinolin-1-yl)naphthalen-2-ol (1j): Addition of 3-(3,5-di-tert-butylphenyl)naphthalen-2-ol (0.1 mmol) to 3-phenyl-3,4-dihydroisoquinoline (0.1 mmol). Flash chromatography (CH2Cl2/hexanes = 1:1) yields a white powder (30 mg, 0.56 mmol, 56 %). mp 149-152 oC; IR (solid): νmax 3284, 2960, 2923, 2861, 1592, 1454, 1414, 1362, 1247, 878, 741, 700; 1H NMR (CDCl3, 500MHz, ppm) δ 12.01 (s, 1H), 8.08 (d, J = 9.0 Hz, 1H), 7.86 (d, J = 8.0 Hz, 1H), 7.80 (s, 1H), 7.53-7.46 (m, 5H), 7.41-7.33 (m, 5H), 7.16-7.13 (m, 2H), 6.99-6.95 (m, 1H), 6.79 (d, J = 8.0 Hz, 1H), 6.38 (s, 1H), 4.37 (dd, J = 11.5, 3.5 Hz, 1H), 3.37-3.32 (m, 1H), 3.12 (dd, J = 16.5, 3.0 Hz, 1H), 2.84 (s, 1H), 1.36 (s, 16H); 13C NMR (CDCl3, 125 MHz, ppm) δ 154.1, 150.0, 142.6, 137.4, 135.8, 134.2, 133.7, 132.9, 130.2, 129.0, 128.9 (2C), 128.6, 128.2, 128.1, 127.1, 126.8, 126.7 (2C), 126.5, 124.2 (2C), 122.8, 121.4, 121.2, 118.1, 59.6, 57.2, 38.4, 34.9, 31.6.
3-(Naphthalen-2-yl)-1-((1S,3R)-3-phenyl-1,2,3,4-tetrahydroisoquinolin-1-yl)naphthalen-2-ol (1k): Flash chromatography (CH2Cl2/hexanes = 1:2) yields a white powder (52 mg, 0.11 mmol, 54 %). mp 151-154 oC; IR (solid): νmax 3280, 2922, 2880, 1621, 1600, 1450, 1415, 1260, 1014, 819, 746, 700; 1H NMR (CDCl3, 500MHz, ppm) δ 12.21 (s, 1H), 8.07 (d, J = 9.0 Hz, 1H), 8.04 (s, 1H), 7.88-7.80 (m, 5H), 7.76 (dd, J = 8.5, 2.0 Hz, 1H), 7.53 (td, J = 7.0, 1.0 Hz, 1H), 7.54-7.43 (m, 4H), 7.39-7.27 (m, 4H), 7.15-7.10 (m, 2H), 6.96 (t, J = 7.0 Hz, 1H), 6.78 (d, J = 8.5 Hz, 1H), 6.34 (s, 1H), 4.30 (dd, J = 11.5, 3.5 Hz, 1H), 3.34-3.28 (m, 1H), 3.09 (dd, J = 16.5, 3.0 Hz, 1H), 2.78 (s, 1H); 13C NMR (CDCl3, 125 MHz, ppm) δ 154.0, 142.4, 136.1, 135.7, 134.1, 133.4, 133.0, 132.6, 132.5, 130.4, 129.0, 128.9, 128.6, 128.3, 128.2, 128.1, 127.5, 127.2, 127.0, 126.9, 126.95, 126.87, 126.5, 125.8, 123.0, 121.2, 118.5, 59.5, 57.1, 38.3; HRMS (EI): calculated for C35H27NO: [M+•] = 477.2093 m/z; found: [M+•] 477.2101 m/z.
3-(Hydroxymethyl)-1-((3R)-3-phenyl-1,2,3,4-tetrahydroisoquinolin-1-yl)naphthalen-2-ol (1l): Preparative TLC (CHCl3/ethyl acetate = 17:1) yields a white powder (36 mg, 0.8 mmol, 40 %). mp 142-146 oC; IR (solid): νmax 3595, 3292, 3057, 2922, 2891, 2853, 1627, 1452, 1406, 1261, 1198, 1055, 1016, 896, 745, 701; 1H NMR (CDCl3, 500MHz, ppm) δ 8.03 (d, J = 8.5 Hz, 1H), 7.79 (d, J = 8.5 Hz, 1H), 7.69 (s, 1H), 7.53-7.47 (m, 3H), 7.41 (t, J = 7.5 Hz, 2H), 7.37-7.33 (m, 2H), 7.14-7.13 (m, 2H), 6.94-6.91 (m, 1H), 6.68 (d, J = 8.0 Hz, 1H), 6.28 (s, 1H), 4.81 (d, J = 13.0 Hz, 1H), 4.75 (d, J = 13.0 Hz, 1H), 4.32 (dd, J = 11.5, 3.5 Hz, 1H), 3.36-3.31 (m,1 H), 3.13 (dd, J = 16.5, 3.5 Hz, 1H); 13C NMR (CDCl3, 125 MHz, ppm) δ 154.5, 142.3, 135.4, 133.9, 133.0, 130.8, 129.03, 128.98, 128.6, 128.3, 128.1, 127.9, 127.40, 127.00, 127.6.69, 126.63, 126.57, 123.1, 121.3, 118.1, 62.9, 59.4, 56.9, 38.2; HRMS (EI): calculated for C26H23NO2: [M+•] = 381.1729 m/z; found: [M+•] 381.1719 m/z.
General Procedure for Diethylzinc Additions to Aldehydes
Under nitrogen atmosphere, 0.5 mL of diethylzinc (1M in heptane) was introduced. To it, was added 0.5 mL of toluene, ligand (0.02 mmol in 0.5 mL toluene) and the corresponding aldehyde (0.25 mmol). The mixture was stirred at 0 oC for 72 h then quenched with 3 mL of 2M HCl. The mixture was washed three times with 5 mL of Et2O, once with brine, dried over MgSO4 and filtered. The solvent was removed and the product, an oil, was isolated by flash chromatography (hexanes/EtOAc = 9:1). All of the below compounds are known compounds and the spectral data are identical to those reported in the literature.10
(R)-1-Phenylpropan-1-ol (6a): Isolated by thin layer chromatography (hexane/EtOAc = 3:1, Rf = 0.4). HPLC (Daicel Chiralcel OD-H, hexane/isopropanol = 97.5:2.5, flow rate = 0.5 mL/min) tR = 22.7 min, tR = 26.4 min, ee = 69 %; 1H NMR (CDCl3, 300 MHz, ppm) δ 7.34-7.20 (m, 5H), 4.54 (t, J = 6.0 Hz, 1H), 2.54 (brs, 1H), 1.86-1.64 (m, 2H), 0.88 (t, J = 6.0 Hz, 3H).
(R)-1-p-Tolylpropan-1-ol (6b): Isolated by thin layer chromatography (hexane/ EtOAc = 3:1, Rf = 0.4). HPLC (Daicel Chiralcel AD-H, hexane/isopropanol = 97.5:2.5, flow rate = 1.0 mL/min) tR = 11.2 min, tR = 12.7 min, ee = 53 %; 1H NMR (CDCl3, 200 MHz, ppm) δ 7.29-7.14 (m, 4H), 4.57 (t, J = 6.6 Hz, 1H), 2.43 (s, 1H), 2.35 (s, 3H), 1.84-1.69 (m, 2H), 0.91 (t, J = 7.6 Hz, 3H).
(R)-1-(4-Methoxyphenyl)propan-1-ol (6c): Isolated by thin layer chromatography (hexane/ EtOAc = 3:1, Rf = 0.5). HPLC (Daicel Chiralcel OD-H, hexane/isopropanol = 95:5, flow rate = 1.0 mL/min) tR = 11.8 min, tR = 13.6 min, ee = 38 %; 1H NMR (CDCl3, 300 MHz, ppm) δ 7.24 (d, J = 11.4 Hz, 2H), 6.87 (d, J = 9Hz, 2H), 4.52 (t, J = 6.6 Hz, 1H), 3.791 (s, 3H), 2.11 (s, 1H), 1.86-1.65 (m, 2H), 0.87=8 (t, J = 7.5 Hz, 3H).
(R)-1-(3-Methoxyphenyl)propan-1-ol (6d): Isolated by thin layer chromatography (hexane/EtOAc = 3:1, Rf = 0.5). HPLC (Daicel Chiralcel OJ-H, hexane/isopropanol = 98:2, flow rate = 0.8 mL/min) tR = 31.5, tR = 33.7 min, ee = 62 %; 1H NMR (CDCl3, 200 MHz, ppm) δ 7.30-7.22 (m, 1H), 6.93-6.79 (m, 3H), 4.57 (t, J = 6.6 Hz, 1H), 3.81 (s, 3H), 1.94 (s, 1H), 1.89-1.69 (m, 2H), 0.92 (t, J = 7.2 Hz, 3H).
(R)-1-(4-Chlorophenyl)propan-1-ol (6e): Isolated by thin layer chromatography (hexane/EtOAc = 3:1, Rf = 0.6). HPLC (Daicel Chiralcel OD-H, hexane/isopropanol = 98:2, flow rate = 1 mL/min) tR = 13.3 min, tR = 14.2 min, ee = 70 %; 1H NMR (CDCl3, 400 MHz, ppm) δ 7.32-7.25 (m, 4H), 4.57 (t, J = 6.8 Hz, 1H), 1.96 (s, 1H), 1.82-1.67 (m, 2H), 0.90 (t, J = 7.2 Hz, 3H).
(R)-1-m-Tolylpropan-1-ol (6f): HPLC (Daicel Chiralcel OB-H, hexane/isopropanol = 95:5, flow rate = 0.5 mL/min) tR = 13.0 min, tR = 15.4 min, ee = 95 %; 1H NMR (CDCl3, 400 MHz, ppm) δ 7.24-7.21 (m, 1H), 7.15-7.07 (m, 3H), 4.53 (t, J = 7.2 Hz, 1H), 2.35 (s, 3H), 1.84-1.69 (m, 2H), 0.91 (t, J = 7.6 Hz, 3H).
(R)-1-(3-Chlorophenyl)propan-1-ol (6g): HPLC (Daicel Chiralcel OD-H, hexane/isopropanol = 96:4, flow rate = 0.5 mL/min) tR = 16.7 min, tR = 17.9 min, ee = 93 %; 1H NMR (CDCl3, 300 MHz, ppm) δ 7.34 (s, 1H), 7.30-7.19 (m, 3H), 4.58 (t, J = 6.8 Hz, 1H), 1.98 (brs, 1H), 1.83-1.71 (m, 2H), 0.91 (t, J = 7.6 Hz, 3H).
(R)-1-(2-Chlorophenyl)propan-1-ol (6h): HPLC (Daicel Chiralcel OB-H, hexane/isopropanol = 97.5:2.5, flow rate = 0.5 mL/min) tR = 15.1 min, tR = 18.4 min, ee = 93 %; 1H NMR (CDCl3, 300 MHz, ppm) δ 7.53 (d, J = 8.0 Hz, 1H), 7.33-7.26 (m, 2H), 7.21-7.17 (m, 1H), 5.06 (brs, 1H), 2.10 (s, 1H), 1.86-1.67 (m, 2H), 0.99 (t, J = 7.2 Hz, 3H).
(R,E)-1-Phenylpent-1-en-3-ol (6i): HPLC (Daicel Chiralcel OD-H, hexane/isopropanol = 95:5, flow rate = 1.0 mL/min) tR = 12.9 min, tR = 21.6 min, ee = 87 %; 1H NMR (CDCl3, 300 MHz, ppm) δ 7.38 (d, J = 7.2 Hz, 1H), 7.32 (t, J = 7.6 Hz, 2H), 7.25 (d, J = 6.8 Hz, 1H), 6.57 (d, J = 16.4 Hz, 1H), 6.21 (dd, J = 15.6, 6.4 Hz, 1H), 4.21 (td, J = 6.4, 6.4 Hz, 1H), 1.73-1.62 (m, 2H), 0.97 (t, J = 7.6 Hz, 3H).
ACKNOWLEDGEMENTS
We are grateful to the Canada Research Chair (Tier I) foundation (to CJL), the CFI, NSERC and ACS-GCI Pharmaceutical Roundtable for support of our research. AR thanks NSERC for a Summer Research Fellowship.
References
1. For an overview, please see: R. Noyori, Adv. Synth. Catal., 2003, 345, 15. CrossRef
2. (a) E. M. Carreira and L. Kvaerno, in Classics in Stereoselective Synthesis, Wiley-VCH Verlag GmbH & Co., Weinheim, 2009; (b) Comprehensive Asymmetric Catalysis; ed. by E. N. Jacobsen, A. Pfaltz, and H. Yamamoto, Springer: New York, 1999; (c) V. Caprio and J. M. J. Williams, Catalysis in Asymmetric Synthesis; Wiley: Ames, 2009; (d) New Frontiers in Asymmetric Catalysis; ed. by K. Mikami and M. Lautens, Wiley: Hoboken, 2007.
3. (a) P. Kocovsky, S. Vyskocil, and M. Smrcina, Chem. Rev., 2003, 103, 3213; CrossRef (b) R. Noyori and H. Takaya, Acc. Chem. Res., 1990, 23, 345. CrossRef
4. N. Gommermann, A. Gehrig, and P. Knochel, Synlett, 2005, 2799. CrossRef
5. J. M. Brunel,Chem. Rev., 2005, 105, 857. CrossRef
6. (a) N. Oguni and T. Omi, Tetrahedron Lett., 1984, 25, 2823; CrossRef (b) M. Kitamura, S. Suga, K. Kawai, and R. Noyori, J. Am. Chem. Soc., 1986, 108, 6071; CrossRef (c) L. Pu and H.-B. Yu, Chem. Rev., 2001, 101, 757; CrossRef (d) K. Soai and S. Niwa, Chem. Rev., 2002, 92, 833. CrossRef
7. (a) M. Betti, Gazz. Chim. Ital., 1900, 30 (II), 301; (b) M. Betti, Org. Synth., 1941, 1, 381.
8. (a) C. Cardellicchio, G. Ciccarella, F. Naso, E. Schingaro, and F. Scordari, Tetrahedron: Asymmetry, 1998, 9, 3667; CrossRef (b) C. Cardellicchio, G. Ciccarella, F. Naso, F. Perna, and P. Tortorella, Tetrahedron, 1999, 55, 14685; CrossRef (c) G. Palmieri, Tetrahedron: Asymmetry, 2000, 11, 3361; CrossRef (d) C. Cimarelli, G. Palmieri, and E. Volpini, Tetrahedron: Asymmetry, 2002, 13, 2417; CrossRef (e) D.-X. Liu, L.-C. Zhang, Q. Wang, C.-S. Da, Z.-Q. Xin, R. Wang, M. C. K. Choi, and A. S. C. Chan, Org. Lett., 2001, 3, 2733; CrossRef (f) I. Szatmári, R. Sillanpää, and F. Fülöp, Tetrahedron: Asymmetry, 2008, 19, 612. CrossRef
9. (a) Z. Li, D. S. Bohle, and C.-J. Li, Proc. Nat. Acad. Sci. (USA), 2006, 103, 8928; CrossRef (b) C.-J. Li, P. D. MacLeod, Z. Li, and J. Feng, U.S. Patent WO 2007098608, 2007; (c) P. D. MacLeod, Z. Li, J. Feng, and C.-J. Li, Tetrahedron Lett., 2006, 47, 6791. CrossRef
10. R. D. Larsen, R. A. Reamer, E. G. Corley, P. Davis, E. J. J. Grabowski, P. J. Reider, and I. Shinkai, J. Org. Chem., 1991, 56, 6034. CrossRef
11. (a) I. Szatmári, L. Lázár, and F. Fülöp, Tetrahedron Lett., 2006, 47, 3881; CrossRef (b) M. Heydenreich, A. Koch, S. Klod, I. Szatmári, F. Fülöp, and E. Kleinpeter, Tetrahedron, 2006, 62, 11081. CrossRef
12. S. Oi, S. Watanabe, S. Fukita, and Y. Inoue, Tetrahedron Lett., 2003, 44, 8665. CrossRef
13. L. E. Miller, W. W. Hanneman, W. L. St. John, and R. R. Smeby, J. Am. Chem. Soc., 1954, 76, 296. CrossRef
14. R. J. Madhushaw, M.-Y. Lin, S. M. A. Sohel, and R.-S. Liu, J. Am. Chem. Soc., 2004, 126, 6895. CrossRef
15. R. B. Bedford and M. E. Limmert, J. Org. Chem., 2003, 68, 8669. CrossRef