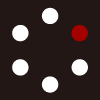
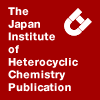
HETEROCYCLES
An International Journal for Reviews and Communications in Heterocyclic ChemistryWeb Edition ISSN: 1881-0942
Published online by The Japan Institute of Heterocyclic Chemistry
e-Journal
Full Text HTML
Received, 10th July, 2009, Accepted, 18th August, 2009, Published online, 19th August, 2009.
DOI: 10.3987/COM-09-S(S)51
■ Novel Formation of Dipyrrolo- and Diindolo[1,2-a:2’,1’-c]quinoxaline Derivatives and Their Optical Properties
Shoji Matsumoto,* Sheng Qu, Takamitsu Kobayashi, Masahiro Kanehiro, Motohiro Akazome, and Katsuyuki Ogura
Faculty of Engineering, Chiba University, 1-33 Yayoi-cho, Inage-ku, Chiba 263-8522, Japan
Abstract
Dipyrrolo- and diindolo[1,2-a:2’,1’-c]quinoxaline structures (A and B, respectively) were synthesized from 1,2-di(1-pyrrolyl)- and 1,2-di(1-indolyl)benzene derivatives with iodine using a novel intramolecular coupling reaction. Apparent differences between A and B were observed from UV-VIS absorption and fluorescence spectra. Sharp peaks with a fine structure were observed in the absorption and fluorescence spectra of diindolo[1,2-a:2’,1’-c]quinoxaline (2b). Introduction of a phenyl ring at the 6 and 6’ positions of the indole moieties of 2b is efficient for imparting a red shift. In addition, the electron-withdrawing group at those positions particularly affected the emission peaks.INTRODUCTION
The chemistry of pyrroles and their analogues extends into various fields such as synthetic methods,1 biological interests,2 and functional materials.3 Especially, optical and electrical applications using a pyrrole skeleton and its π-conjugated systems have attracted much attention to the development of electric conducting materials,4 sensors,5 nonlinear optics,6 fluorescent materials,7 electroluminescent materials,8 organic metal-lustrous compounds,9 and so on.10 Furthermore, several unique structures including the pyrrole skeleton have been investigated to develop new useful physical properties. For example, expanded porphyrins,11 dipyrromethenes,12 dipyrrolylquinoxalines,13 and calix[4]pyrroles14 were investigated thoroughly as functional materials. Recently, we reported the synthesis and optical properties of novel 2,2’-bipyrroles, 1,1’,5,5’-tetraaryl-2,2’-bipyrrole derivatives: their conformation in an excited state is closer to a planar one, but a twisted conformation is favorable in a ground state.15 If the conformation is forced in the ground state to be planar, they would resemble the pyrene system that seems to be applicable to various sensors using fluorescence ability.16 Hence, we were interested in 2,2’-bipyrrole structures bridging the nitrogen atom with the phenylene group that are dipyrrolo[1,2-a:2’,1’-c]quinoxalines (A) and their π-expanded structures, diindolo[1,2-a:2’,1’-c]quinoxalines (B). A few reports have described the synthesis of A as well as its application to conducting polymers,17,18 and no report has addressed the structure B. Therefore, we started our investigation of the synthesis and optical properties of A and B to develop the novel optical and electrical devices. Until then, two syntheses of the structure A had been reported: in the literature by Kaupp et al., the first included the [4+2] cycloaddition of 2,3-dimethylquinoxaline dioxide and phenylpropiolate.17b Pagani and his co-workers achieved the synthesis through the subsequent cyclization of 2,3-dimethylquinoxaline with ethyl 3-bromopyruvate.17a Those reported routes are impressive, but they might not be applied to the synthesis of the expanded structure B. Therefore, we planned the intramolecular C–C bond formation of the corresponding 1,2-di(1-pyrrolyl)- and 1,2-di(1-indolyl)benzene derivatives with iodine, which is similar to the cyclization-aromatization reaction of stilbene (Scheme 1).19,20 The starting 1,2-di(1-pyrrolyl)- and 1,2-di(1-indolyl)benzene derivatives can be obtained through CuI-catalyzed coupling reaction of 1,2-dihalobenzene with pyrrole and indole derivatives.21 Herein, we report the novel formation of dipyrrolo- and diindolo[1,2-a:2’,1’-c]quinoxaline derivatives (A and B) using the pyrrole–pyrrole coupling reaction with iodine together with the relationship between their structures and optical properties.
RESULTS AND DISCUSSION
We examined the photochemical reaction of 1,2-di(1-pyrrolyl)benzene (1a), which was prepared by the CuI-catalyzed coupling reaction of 1,2-dihalobenzene with pyrrole and indole derivatives,21 in the presence of iodine. When 1a was treated with 1 mol-equivalent of iodine in toluene for 5.5 h under irradiation with a high-pressure mercury lamp (100 W) through a Pyrex filter, the desired dipyrrolo[1,2-a:2’,1’-c]quinoxaline (2a) was obtained in 49% yield with the recovery of 1a (11%) (Entry 1 in Table 1). Increasing and decreasing the amount of iodine was not effective (Entries 1, 2, and 3). Surprisingly, a comparable result was obtained with a trace amount of starting 1a when the reaction was conducted under dark conditions (Entry 1 vs. 5). But every runs in toluene and acetonitrile gave the unidentified compounds. Furthermore, we found that 2a was obtained in high yield (85% yield) using chlorobenzene as a solvent (Entry 6). A similar pyrrole–pyrrole intramolecular coupling reactions using Fe(ClO4)3 or FeCl3 were reported as the oxidant.22 Consequently, this reaction occurred because of iodine’s oxidative character. The detailed mechanism in our conditions remains unclear, but the slight change of a polarity and/or the absence of the active benzylic proton in a solvent would enhance the yield of 2a. This reaction might be applicable to the indolyl analogues. The excellent yield was achieved by raising the temperature and increasing the amount of iodine (Entry 9). And the substituted compounds at the phenyl rings of indolyl moiety also formed the corresponding products in good yields (Entries 10 and 11).
To examine the substituent effect of structure A on optical properties, we tried to synthesize 3,10-diphenyldipyrrolo[1,2-a:2’,1’-c]quinoxaline (2e) in our coupling method. However, we were unable to form the starting 1,2-di(2-phenyl-1-pyrrolyl)benzene using the CuI-mediated reaction. Therefore, we obtained 2e using the reaction of palladium-catalyzed arylation with 2a according to the procedure reported by Sanford and his co-workers (Scheme 2).23
The absorption and fluorescence spectra were measured in the THF solution. The physical data discussed in this manuscript are presented in Table 2. We first examined the effect of the expansion of the conjugated system from 2a. The absorption and emission peaks of 2e showed longer wavelengths than that of 2a (Entry 1 vs. 2 in Table 2). Kaupp and co-workers reported the absorption peaks of 2,11-diphenyldipyrrolo[1,2-a:2’,1’-c]quinoxaline (2f), which is an analogue of 2a bearing two phenyl rings at 4 and 4’ positions of each pyrrole moiety.17b It showed a blue-shift against 2a (Entry 1 vs. 3). Consequently, the introduction of additional π-system at 5 and 5’ positions of pyrrole part of structure A is efficient to impart a red shift in the absorption spectra. Moreover, 2b, which has the fused aromatic system in 2a, also showed longer wavelength of the absorption peak (Entries 1 and 4). Although the peaks of 2a and 2e at λmax were broadened, the spectrum of 2b showed a characteristic fine structure (absorption peaks: 371.5, 351, and 336.5 nm) with the sharp edge of the absorption (Figure 1). The structure B will have the more fixed conformation by the fused benzene rings than the structure A. And we think that it is the reason to give the different properties between structure A and B. But we were unable to find a proper explanation for such a difference between structure A and B despite computational studies at the DFT B3LYP/6-31G* level calculation.
A marked dissimilarity between dipyrrolo[1,2-a:2’,1’-c]quinoxaline derivatives (2a and 2e) and diindolo[1,2-a:2’,1’-c]quinoxaline (2b) was also observed in the fluorescence spectra (Figure 1). The three main peaks similar to those in the absorption spectrum were observed in the emission spectrum of 2b, although one broadened emission peak was obtained in the case of 2a and 2e. It is particularly interesting that the Stokes shift of 2b is small (2 nm), suggesting that the difference between the equilibrium geometries of the ground and excited states is small.24 Consequently, the fluorescence quantum yield (ΦF) was increased (2a; 0.43, 2b; 0.55) (Entries 1 and 4 in Table 2).
We further investigated the substituent effect of 2b on absorption and fluorescence properties (Table 2 and Figure 2). When two phenyl rings were attached at 5 and 5’ positions of the indolyl moiety of 2b, the absorption peak of 2c showed a red shift (Δλmax = 7 nm) (Entries 4 and 5). The emission peak shifted similarly (Δλem = 10 nm in the shortest wavelength). In comparison with 2c, a larger red shift from the spectra of 2b was observed both in the absorption (Δλmax = 26 nm) and in the emission (Δλem = 42 nm in the shortest wavelength) spectra of 2d, which possessed in the phenyl groups at 6 and 6’ positions of indole moiety of 2b (Entry 4 vs. 6). Therefore, we showed that the introduction of another π-system into 2b at the 6 position of the iodole moiety is efficient to achieve expansion of π-system in diindolo[1,2-a:2’,1’-c]quinoxaline system (B). The fine structures were observed even in the introduction of the phenyl groups with gradual broadening.
Additionally, we tried to investigate the electronic effect of the substituents at 6 and 6’ position of indolyl parts. For this purpose, 2g and 2h were synthesized by the reaction of the CuI-catalyzed coupling reaction followed by the iodine-mediated cyclization reaction. The 2g bearing 4-methoxyphenyl groups showed a small difference in the absorption and fluorescence spectra compared to those of 2d (Entries 6 and 7 in Table 2). A specific difference was observed when 2h bearing 4-trifluoromethylphenyl groups was examined. In the absorption spectrum, almost identical maximum absorption peaks with 2g were obtained with a decrease of the absorption coefficient. In contrast, the emission peaks of 2h showed a red shift of about 10 nm compared to those of 2d and 2g (Entry 6, 7 vs. 8). These results suggest that the introduction of the electron-withdrawing substituent at 6 and 6’ positions of indole part in diindolo[1,2-a:2’,1’-c]quinoxaline structure efficiently changes the emission peaks, with a small change of the absorption peaks.
In conclusion, we synthesized dipyrrolo- and diindolo[1,2-a:2’,1’-c]quinoxaline derivatives and investigated their optical properties. Their syntheses were achieved using the novel C–C bond formation with iodine, whose reaction conditions are simple and inexpensive. The UV-VIS and fluorescence spectra revealed different characteristics of dipyrrolo- and diindolo[1,2-a:2’,1’-c]quinoxaline structures. Furthermore, an efficient change in the spectra of structure B was obtained when the additional π-systems at 6 and 6’ positions of indolyl moiety were introduced. Notably, the electron-withdrawing group at those positions induced a greater red shift of the emission peak than the absorption peak. These findings will elucidate not only synthetic methods of novel pyrrole-containing compounds but also facilitate the design of functional materials of new types.
EXPERIMENTAL
General Procedures. Melting points were determined with Yanaco MP-J3 and values were uncorrected. 1H NMR spectra were recorded at 300 MHz on Varian GEMINI 2000 spectrometer with TMS as an internal standard. J-Values were given in Hz. IR spectra were measured on a JASCO FT/IR-350 spectrometer. UV-VIS spectra were measured on a JASCO V570 spectrophotometer. Fluorescence spectra were measured on a JASCO FP-6600 spectrofluorometer. Elemental analyses (EA) and high-resolution mass spectroscopy (HRMS) were carried out by the Chemical Analysis Center of Chiba University.
Procedure for Synthesis of 1,2-Di(1-pyrrolyl)benzene (1a). In a test tube with a screw cap was added 1,2-diiodobenzene (0.13 mL, 1.00 mmol), pyrrole (0.21 mL, 3.0 mmol), CuI (19.0 mg, 0.1 mmol), trans-1,2-diaminocyclohexane (0.12 mL, 1.0 mmol), K3PO4 (0.850 g, 4.0 mmol), and toluene (1.0 mL). The combined mixture was warmed to 110 oC for 10 h. The reaction mixture was cooled down to room temperature. The mixture was filtered off with a pad of Celite and Florisil® and washed with EtOAc. Combined organic solution was evaporated in vacuo. The residual mixture was subjected to column chromatography on silica gel (hexane : CHCl3 = 6 : 1) to give 1a (0.152 g, 73%) as colorless solid. Rercrystallization from hexane gave colorless plate crystals: mp 75.0-75.5 oC (hexane); 1H NMR (CDCl3) δ 6.22 (t, J = 2.2 Hz, 4H), 6.54 (t, J = 2.2 Hz, 4H), 7.37-7.44 (m, 4H); IR (KBr/cm-1) 3132, 3101, 3068, 1603, 1514, 1484, 1333, 1070, 761, 723. Anal. Calcd for C14H12N2: C, 80.74; H, 5.81; N, 13.45. Found: C, 80.78; H, 5.88; N, 13.54.
1,2-Di(1-indolyl)benzene (1b).25 The titled compound was prepared for 3 d in 33% according to a procedure similar to that mentioned above: colorless plate crystals: mp 164.7-165.3 oC (hexane); 1H NMR (CDCl3) δ 6.36 (d, J = 3.2 Hz, 2H), 6.54 (d, J = 3.2 Hz, 2H), 7.12 (m, 4H), 7.32 (dd, J = 5.3 and 3.2 Hz, 2H), 7.56 (m, 4H), 7.70 (m, 2H); IR (KBr/cm-1) 3048, 1594, 1516, 1456, 1331, 1308, 1238, 1211, 1134, 1009, 766, 748, 715.
1,2-Di(5-phenyl-1-indolyl)benzene (1c). The titled compound was prepared with 5-phenylindole and 1,2-dibromobenzene for 44 h in 43% yield according to a procedure similar to that mentioned above: colorless solid: mp 155.3-156.6 oC (hexane-CHCl3); 1H NMR (CDCl3) δ 6.44 (d, J = 3.3 Hz, 2H), 6.62 (d, J = 3.3 Hz, 2H), 7.30 (t, J = 7.3 Hz, 2H), 7.40 (s, 4H), 7.43 (t, 7.3 Hz, 4H), 7.58 (dd, J = 6.0 and 3.6 Hz, 2H), 7.63 (d, J = 7.1 Hz, 4H), 7.74 (dd, J = 6.0 and 3.4 Hz, 2H), 7.79 (s, 2H); IR (KBr/cm-1) 3056, 1599, 1508, 1469, 1369, 1334, 1234, 1180, 1120, 887, 787, 754, 721, 698. HRMS Calcd for C34H25N2: M+H. 461.2018, found: m/z 461.1986.
1,2-Di(6-phenyl-1-indolyl)benzene (1d). The titled compound was prepared with 6-phenylindole and 1,2-dibromobenzene for 44 h in 38% yield according to a procedure similar to that mentioned above: colorless needle crystals: mp 201.4-202.7 oC (hexane-CHCl3); 1H NMR (CDCl3) δ 6.44 (d, J = 3.3 Hz, 2H), 6.78 (d, J = 3.0 Hz, 2H), 7.21-7.27 (m, 10H), 7.35 (d, J = 8.1 Hz, 2H), 7.45 (s, 2H), 7.56 (dd, J = 5.9 and 3.5 Hz, 2H), 7.59 (d, J = 8.4 Hz, 2H), 7.73 (dd, J = 5.9 and 3.6 Hz, 2H); IR (KBr/cm-1) 3058, 1599, 1512, 1473, 1433, 1344, 1213, 1138, 1074, 1038,904, 864, 818, 762, 698. HRMS Calcd for C34H25N2: M+H. 461.2018, found: m/z 461.1986.
Procedure for Synthesis of Dipyrrolo[1,2-a:2’,1’-c]quinoxaline (2a). To a solution of 1a (0.104 g, 0.50 mmol) in chlorobenzene (3.0 mL) was dropwise added a solution of iodine (0.139 g, 0.55 mmol) in chlorobenzene (2.0 mL) in a period of 5 min. The mixture was stirred at room temperature. The dark precipitate was observed when the reaction proceeded. After being stirred for 24 h at room temperature, to the reaction mixture was added saturated aqueous Na2S2O3 solution (5 mL) and acetone (ca. 5 mL) to dissolve the precipitate. The combined mixture was extracted with CHCl3 (5 mL × 3). The combined organic layers were dried over MgSO4. Evaporation in vacuo and column chromatography (silica gel; hexane : EtOAc = 10 : 1) provided 2a (0.088 g, 85%) as colorless solid. Recrystallization from hexane gave colorless crystals: mp 153.5-154.5 oC (hexane) (lit.,17a 148-150 oC); 1H NMR (CDCl3) δ 6.54 (dd, J = 3.7 and 1.5 Hz, 2H), 6.57 (t, J = 3.7 Hz, 2H), 7.27 (dd, J = 6.0 and 1.4 Hz, 2H), 7.47 (dd, J = 2.9 and 1.4 Hz, 2H), 7.71 (dd, J = 6.2 and 1.4 Hz, 2H); IR (KBr/cm-1) 3137, 3099, 1625, 1516, 1487, 1348, 1088, 746, 698, 688.
Diindolo[1,2-a:2’,1’-c]quinoxaline (2b). The titled compound was prepared for 24 h at 80 oC in 85% yield according to a procedure similar to that mentioned above: colorless cotton-like solid: mp 244.0-244.6 oC (hexane); 1H NMR (CDCl3) δ 7.08 (s, 2H), 7.25-7.39 (m, 6H), 7.74 (dd, J = 5.9 and 0.9 Hz, 2H), 8.21 (d, J = 8.5 Hz, 2H), 8.41 (m, 2H); IR (KBr/cm-1) 3045, 1504, 1465, 1446, 1380, 1363, 1334, 1265, 792, 775, 738, 727. Anal. Calcd for C22H14N2: C, 86.02; H, 4.32; N, 8.98. Found: C, 86.25; H, 4.61; N, 9.14.
2,13-Diphenyldiindolo[1,2-a:2’,1’-c]quinoxaline (2c). The titled compound was prepared for 24 h at 80 oC in 80% yield according to a procedure similar to that mentioned above: orange solid: mp 287.4-287.9 oC (hexane-CHCl3); 1H NMR (CDCl3) δ 7.19 (s, 2H), 7.37 (t, J = 7.5 Hz, 2H), 7.41 (dd, J = 6.2 and 2.9 Hz, 2H), 7.49 (t, J = 7.5 Hz, 4H), 7.64 (dd, J = 9.0 and 1.8 Hz, 2H), 7.73 (d, J = 7.5 Hz, 4H), 7.98 (d, J = 1.8 Hz, 2H), 8.30 (d, J = 8.7 Hz, 2H), 848 (dd, J = 6.2 and 3.6 Hz, 2H); IR (KBr/cm-1) 3057, 1597, 1537, 1500, 1464, 1377, 1338, 1298, 1269, 879, 758, 696, 621, 526, 451, 418, 404. Anal. Calcd for C34H22N2·1/3H2O: C, 87.90; H, 4.92; N, 6.03. Found: C, 87.98; H, 4.83; N, 5.93.
3,12-Diphenyldiindolo[1,2-a:2’,1’-c]quinoxaline (2d). The titled compound was prepared for 17 h at 80 oC in 75% yield according to a procedure similar to that mentioned above: orange solid: mp 276.2-276.7 oC (hexane-CHCl3); 1H NMR (CDCl3) δ 7.15 (s, 2H), 7.40 (m, 4H), 7.52 (t, J = 7.5 Hz, 4H), 7.57 (d, J = 9.2 Hz, 2H), 7.74 (d, J = 7.5 Hz, 4H), 7.82 (d, J = 8.1 Hz, 2H), 8.42 (s, 2H), 8.52 (dd, J = 5.9 and 3.3 Hz, 2H); IR (KBr/cm-1) 3030, 2362, 1599, 1527, 1498, 1477, 1448, 1427, 1381, 1342, 1300, 1250, 814, 769, 739, 692, 418. Anal. Calcd for C34H22N2·1/4H2O: C, 88.19; H, 4.90; N, 6.05. Found: C, 88.30; H, 4.55; N, 6.08.
Synthesis of 3,10-Diphenyldipyrrolo[1,2-a:2’,1’-c]quinoxaline (2e). PhI(OAc)2 (0.903 g, 2.80 mmol) and PhB(OH)2 (0.342 g, 2.80 mmol) was added to AcOH (14 mL), which was bubbled with N2 gas for 20 min. After being stirred for 15 min at room temperature, 1a (0.143 g, 0.69 mmol) was added to the mixture. The color of the reaction mixture was turned from pale red to black. After being stirred for additional 15min, Pd(OAc)2 (31.2 mg, 0.14 mmol) was added and then the whole was stirred for 24 h at room temperature. The reaction mixture was filtered through a plug of Celite and evaporated in vacuo. After being added water (5 mL). The combined mixture was extracted with CHCl3 (5 mL × 3). The combined organic layers were dried over MgSO4. Evaporation in vacuo and column chromatography (silica gel; hexane : EtOAc = 40 : 1) provided 2e (84.9 mg, 34%) as yellow solid. Recrystallization from MeOH and a bit CHCl3 gave pale yellow crystals: mp 164.0-165.1 oC; 1H NMR (CDCl3) δ 6.55 (d, J = 3.7 Hz, 2H), 6.62 (d, J = 3.7 Hz, 2H), 6.81 (dd, J = 6.3 and 3.5 Hz, 2H), 7.30 (dd, J = 6.3 and 3.5 Hz, 2H), 7.33 (t, J = 7.3 Hz, 2H), 7.41 (t, J = 7.2 Hz, 4H), 7.54 (d, J = 7.1 Hz, 4H); IR (KBr/cm-1) 3057, 1600, 1496, 1471, 1372, 1352, 1335, 755, 702. Anal. Calcd for C26H18N2·1/4H2O: C, 86.04; H, 5.14; N, 7.72. Found: C, 85.86; H, 4.85; N, 7.68.
3,12-Di(4-methoxyphenyl)diindolo[1,2-a:2’,1’-c]quinoxaline (2g). The titled compound was prepared in two steps (CuI-mediated coupling reaction in 40% yield, and iodine-mediated cyclization reaction for 16 h at 80 oC in 100% yield) according to a procedure similar to that mentioned above: yellow solid: mp 280.8-283.0 oC (hexane-CHCl3); 1H NMR (CDCl3) δ 3.89 (s, 6H), 7.05 (d, J = 8.7 Hz, 4H), 7.09 (s, 2H), 7.37 (dd, J = 5.9 and 3.5 Hz, 2H), 7.50 (d, J = 8.4 Hz, 2H), 7.65 (d, J = 8.7 Hz, 4H), 7.77 (d, J = 8.4 Hz, 2H), 8.34 (s, 2H), 8.47 (dd, J = 5.8 and 3.1 Hz, 2H); IR (KBr/cm-1) 2935, 2833, 1606, 1502, 1481, 1437, 1375, 1246, 1178, 1039, 818, 737. Anal. Calcd for C36H26N2O2·1/3H2O: C, 82.42; H, 5.12; N, 5.34. Found: C, 82.29; H, 4.92; N, 5.26.
3,12-Di[4-(trifluoromethyl)phenyl]diindolo[1,2-a:2’,1’-c]quinoxaline (2h). The titled compound was prepared in two steps (CuI-mediated coupling reaction in 42% yield, and iodine-mediated cyclization reaction for 22 h at 80 oC in 100% yield) according to a procedure similar to that mentioned above: pale yellow solid: mp 286.0-287.8 oC (hexane-CHCl3); 1H NMR (CDCl3) δ 7.18 (s, 2H), 7.43 (dd, J = 6.2 and 3.4 Hz, 2H), 7.56 (dd, J = 8.1 and 1.2 Hz, 2H), 7.75 (m, 4H), 7.84 (m, 6H), 8.42 (s, 2H), 8.49 (dd, J = 6.2 and 3.5 Hz, 2H); IR (KBr/cm-1) 2927, 2851, 1482, 1439, 1379, 1328, 1166, 1122, 1070, 816, 738. HRMS Calcd for C36H20F6N2: M. 594.1531, found: m/z 594.1538.
ACKNOWLEDGEMENTS
This work was supported by a Grant-in-Aid for Young Scientists (B) (20750026) from the Japan Society for the Promotion of Science and by Toyo Ink Mfg. Co., Ltd.
References
1. K. C. Majumdar, Synlett, 2008, 2400; CrossRef N. Ono, Heterocycles, 2008, 75, 243; CrossRef S. Cacchi and G. Fabrizi, Chem. Rev., 2005, 105, 2873. CrossRef
2. J. C. Morris and A. J. Phillips, Nat. Prod. Rep., 2008, 25, 95; CrossRef F. Bellina and R. Rossi, Tetrahedron, 2006, 62, 7213; CrossRef C. T. Walsh, S. Garneau-Tsodikova, and A. R. Howard-Jones, Nat. Prod. Rep., 2006, 23, 517; CrossRef J. W. Huffman and L. W. Padgett, Curr. Med. Chem., 2005, 12, 1395. CrossRef
3. J. L. Sessler, S. Camiolo, and P. A. Gale, Coord. Chem. Rev., 2003, 240, 17; J. Soloducho, Synth. Met., 1999, 99, 181; CrossRef A. Deronzier and J. -C. Moutet, Coord. Chem. Rev., 1996, 147, 339. CrossRef
4. Review: G. A. Pagani, Heterocycles, 1994, 37, 2069; CrossRef (a) G. Zotti, B. Vercelli, and A. Berlin, Chem. Mater., 2008, 20, 397; CrossRef (b) V. Bajpai, P. He, L. Goettler, J. H. Dong, and L. Dai, Synth. Met., 2006, 156, 466. CrossRef
5. Review: P. A. Gale, Acc. Chem. Res., 2006, 39, 465; CrossRef (a) A. Ikeda, M. Nakasu, S. Ogasawara, H. Nakanishi, M. Nakamura, and J. Kikuchi, Org. Lett., 2009, 11, 1163; CrossRef (b) C. Ikeda, N. Sakamoto, and T. Nabeshima, Org. Lett., 2008, 10, 4601; CrossRef (c) C. -I. Lin, S. Selvi, J. -M. Fang, P. -T. Chou, C. -H. Lai, and Y. -M. Cheng, J. Org. Chem., 2007, 72, 3537. CrossRef
6. (a) M. M. M. Raposo, A. M. R. C. Sousa, G. Kirsch, P. Cardoso, M. Belsley, E. M. Gomes, and A. M. C. Fonseca, Org. Lett., 2006, 8, 3681; CrossRef (b) K. Ogura, H. Yanai, M. Miokawa, and M. Akazome, Tetrahedron Lett., 1999, 40, 8887. CrossRef
7. (a) S. S. Palayangoda, X. Cai, R. M. Adhikari, and D. C. Neckers, Org. Lett., 2008, 10, 281; CrossRef (b) X. -F. Duan, J. -L. Wang, and J. Pei, Org. Lett., 2005, 7, 4071. CrossRef
8. (a) J. Y. Jeon, T. J. Park, W. S. Jeon, J. J. Park, J. Jang, J. H. Kwon, and J. Y. Lee, Chem. Lett., 2007, 1156; CrossRef (b) Y. Li, L. Cao, Z. Ning, Z. Huang, Y. Cao, and H. Tian, Tetrahedron Lett., 2007, 48, 975; CrossRef (c) Y. Liu, M. Nishiura, Y. Wang, and Z. Hou, J. Am. Chem. Soc., 2006, 128, 5592; CrossRef (d) H. Yanai, D. Yoshizawa, S. Tanaka, T. Fukuda, M. Akazome, and K. Ogura, Chem. Lett., 2000, 238. CrossRef
9. (a) K. Ogura, K. Ooshima, M. Akazome, and S. Matsumoto, Tetrahedron, 2006, 62, 2484; CrossRef (b) K. Ogura, K. Ooshima, M. Akazome, and S. Matsumoto, Tetrahedron, 2006, 62, 2413; CrossRef (c) K. Ogura, R. Zhao, T. Mizuoka, M. Akazome, and S. Matsumoto, Org. Biomol. Chem., 2003, 1, 3845; CrossRef (d) K. Ogura, R. Zhao, M. Jiang, M. Akazome, S. Matsumoto, and K. Yamaguchi, Tetrahedron Lett., 2003, 44, 3595; CrossRef (e) R. Zhao, M. Akazome, S. Matsumoto, and K. Ogura, Tetrahedron, 2002, 58, 10233; CrossRef (f) R. Zhao, M. Akazome, S. Matsumoto, and K. Ogura, Tetrahedron, 2002, 58, 10225; CrossRef (g) K. Ogura, R. Zhao, H. Yanai, K. Maeda, R. Tozawa, S. Matsumoto, and M. Akazome, Bull. Chem. Soc. Jpn., 2002, 75, 2359. CrossRef
10. (a) J. Setsune, A. Tsukajima, N. Okazaki, J. M. Lintuluoto, and M. Lintuluoto, Angew. Chem. Int. Ed. 2009, 48, 771; CrossRef (b) S. A. DiBenedetto, D. Frattarelli, M. A. Ratner, A. Facchetti, and T. J. Marks, J. Am. Chem. Soc., 2008, 130, 7528; CrossRef (c) P. A. Liddell, M. Gervaldo, J. W. Bridgewater, A. E. Keirstead, S. Lin, T. A. Moore, A. L. Moore, and D. Gust, Chem. Mater., 2008, 20, 135; CrossRef (d) Z. Zhang, P. Liang, X. Zheng, D. Peng, F. Yan, R. Zhao, and C. -L. Feng, Biomacromolecules, 2008, 9, 1613; CrossRef (e) S. Pu, G. Liu, L. Shen, and J. Xu, Org. Lett., 2007, 9, 2139; CrossRef (f) M. J. Smith, W. Clegg, K. A. Nguyen, J. E. Rogers, R. Pachter, P. A. Fleitz, and H. L. Anderson, Chem. Commun., 2005, 2433; CrossRef (g) S. Wakim, J. Bouchard, N. Blouin, A. Michaud, and M. Leclerc, Org. Lett., 2004, 6, 3413. CrossRef
11. Reviews: R. Misra and T. K. Chandrashekar, Acc. Chem. Res., 2008, 41, 265; CrossRef J. L. Sessler and D. Seidel, Angew. Chem. Int. Ed., 2003, 42, 5134; CrossRef (a) S. Tokuji, J. -Y. Shin, K. S. Kim, J. M. Lim, K. Youfu, S. Saito, D. Kim, and A. Osuka, J. Am. Chem. Soc., 2009, 131, 7240; CrossRef (b) Z. S. Yoon, J. H. Kwon, M. -C. Yoon, M. K. Koh, S. B. Noh, J. L. Sessler, J. T. Lee, D. Seidel, A. Aguilar, S. Shimizu, M. Suzuki, A. Osuka, and D. Kim, J. Am. Chem. Soc., 2006, 128, 14128; CrossRef (c) H. Rath, V. G. Anand, J. Sankar, S. Venkatraman, T. K. Chandrashekar, B. S. Joshi, C. L. Khetrapal, U. Schilde, and M. O. Senge, Org. Lett., 2003, 5, 3531. CrossRef
12. Reviews: G. Ulrich, R. Ziessel, and A. Harriman, Angew. Chem. Int. Ed., 2008, 47, 1184; CrossRef A. Loudet and K. Burgess, Chem. Rev., 2007, 107, 4891; CrossRef R. Ziessel, G. Ulrich, and A. Harriman, New J. Chem., 2007, 31, 496; CrossRef (a) M. K. Kuimova, G. Yahioglu, J. A. Levitt, and K. Suhling, J. Am. Chem. Soc., 2008, 130, 6672; CrossRef (b) M. A. H. Alamiry, A. C. Benniston, G. Copley, K. J. Elliott, A. Harriman, B. Stewart, and Y. -G. Zhi, Chem. Mater., 2008, 20, 4024; CrossRef (c) V. A. Azov, A. Schlegel, and F. Diederich, Angew. Chem. Int. Ed., 2005, 44, 4635; CrossRef (d) W. Zhao and E. M. Carreira, Angew. Chem. Int. Ed., 2005, 44, 1677. CrossRef
13. (a) J. Yoo, M. -S. Kim, S. -J. Hong, J. L. Sessler, and C. -H. Lee, J. Org. Chem., 2009, 74, 1065; CrossRef (b) J. L. Sessler, D. -G. Cho, and V. Lynch, J. Am. Chem. Soc., 2006, 128, 16518; CrossRef (c) S. V. Shevchuk, V. M. Lynch, and J. L. Sessler, Tetrahedron, 2004, 60, 11283; CrossRef (d) J. L. Sessler, H. Maeda, T. Mizuno, V. M. Lynch, and H. Furuta, J. Am. Chem. Soc., 2002, 124, 13474. CrossRef
14. Review: P. Anzenbacher Jr., R. Nishiyabu, and M. A. Palacios, Coord. Chem. Rev., 2006, 250, 2929; CrossRef (a) M. A. Palacios, R. Nishiyabu, M. Marquez, and P. Anzenbacher Jr., J. Am. Chem. Soc., 2007, 129, 7538; CrossRef (b) H. Miyaji, S. -J. Hong, S. -D. Jeong, D. -W. Yoon, H. -K. Na, J. Hong, S. Ham, J. L. Sessler, and C. -H. Lee, Angew. Chem. Int. Ed., 2007, 46, 2508; CrossRef (c) R. Nishiyabu and P. Anzenbacher Jr., Org. Lett., 2006, 8, 359; CrossRef (d) R. Nishiyabu and P. Anzenbacher Jr., J. Am. Chem. Soc., 2005, 127, 8270; CrossRef (e) H. Miyaji, W. Sato, and J. L. Sessler, Angew. Chem. Int. Ed., 2000, 39, 1777. CrossRef
15. (a) S. Matsumoto, T. Kobayashi, and K. Ogura, Heterocycles, 2006, 68, 283; CrossRef (b) S. Matsumoto, T. Kobayashi, and K. Ogura, Heterocycles, 2005, 66, 319. CrossRef
16. (a) S. Malashikhin and N. S. Finney, J. Am. Chem. Soc., 2008, 130, 12846; CrossRef (b) M. Y. Lo, C. Zhen, M. Lauters, G. E. Jabbour, and A. Sellinger, J. Am. Chem. Soc., 2007, 129, 5808; CrossRef (c) H. N. Lee, N. J. Singh, S. K. Kim, J. Y. Kwon, Y. Y. Kim, K. S. Kim, and J. Yoon, Tetrahedron Lett., 2007, 48, 169; CrossRef (d) Y. Shiraishi, Y. Tokitoh, and T. Hirai, Org. Lett., 2006, 8, 3841; CrossRef (e) S. H. Kim, J. K. Choi, S. K. Kim, W. Sim, and J. S. Kim, Tetrahedron Lett., 2006, 47, 3737. CrossRef
17. Synthesis: (a) A. Berlin, S. Martina, G. Pagani, G. Schiavon, and G. Zotti, Heterocycles, 1991, 32, 85; CrossRef (b) G. Kaupp, H. Voss, and H. Frey, Angew. Chem., Int. Ed. Engl., 1987, 26, 1280. CrossRef
18. Application: (a) A. Berlin, G. Pagani, G. Zotti, and G. Schiavon, Makromol. Chem., 1993, 194, 1137; CrossRef (b) A. Berlin, S. Martina, G. Pagani, G. Schiavon, and G. Zotti, Synth. Met., 1991, 41, 363; CrossRef (c) G. Zotti, G. Schiavon, A. Berlin, and G. Pagani, Synth. Met., 1991, 40, 299. CrossRef
19. F. B. Mallory and C. W. Mallory, Org. React., 1984, 30, 1.
20. Recently, it was reported that the pyrroles undergoes the intermolecular oxidative coupling by the combination of phenyliodine(III) bis(trifluoroacetate) and bromotrimethylsilane to give 2,2’-bipyrrole derivatives: (a) T. Dohi, K. Morimoto, M. Ito, and Y. Kita, Synthesis, 2007, 2913; CrossRef (b) T. Dohi, K. Morimoto, A. Maruyama, and Y. Kita, Org. Lett., 2006, 8, 2007. CrossRef
21. J. C. Antilla, A. Klapars, and S. L. Buchwald, J. Am. Chem. Soc., 2002, 124, 11684. CrossRef
22. (a) M. Takase, V. Enkelmann, D. Sebastiani, M. Baumgarten, and K. Müllen, Angew. Chem. Int. Ed., 2007, 46, 5524; CrossRef (b) M. Lazerges, M. Jouini, P. Hapiot, P. Guiriec, and P. -C. Lacaze, J. Phys. Chem. A, 2003, 107, 5042. CrossRef
23. N. R. Deprez, D. Kalyani, A. Krause, and M. S. Sanford, J. Am. Chem. Soc., 2006, 128, 4972. CrossRef
24. M. Klessinger and J. Michl, ‘Excited States and Photochemistry of Organic Molecules’, VHC, New York, 1995, pp. 260-276.
25. S. Maiorana, C. Baldoli, P. D. Buttero, M. D. Ciolo, and A. Papagni, Synthesis, 1998, 735. In this report, two protons were missing based on the total integration of the proton. In our data, these protons were observed at 6.54 ppm as a doublet. CrossRef