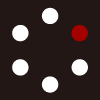
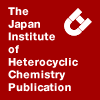
HETEROCYCLES
An International Journal for Reviews and Communications in Heterocyclic ChemistryWeb Edition ISSN: 1881-0942
Published online by The Japan Institute of Heterocyclic Chemistry
e-Journal
Full Text HTML
Received, 28th July, 2009, Accepted, 25th August, 2009, Published online, 26th August, 2009.
DOI: 10.3987/COM-09-S(S)82
■ Synthesis of Macrosphelides Containing a Heterocyclic Side Chain as a Novel Apoptosis Inducer
Yuji Matsuya,* Ayana Hori, Tomomi Kawamura, Heba F. Emam, Kanwal Ahmed, Da-Yong Yu, Takashi Kondo, Naoki Toyooka, and Hideo Nemoto
Faculty of Pharmaceutical Sciences, Toyama University, 2630 Sugitani, Toyama, Toyama 930-0194, Japan
Abstract
Synthesis of macrosphelides containing a pyridyl or benzimidazolyl side chain is described. The synthesis was accomplished through sequential assembling of suitable chiral blocks and ring-closing metathesis (RCM) with a high efficiency. These novel derivatives were evaluated on the apoptosis-inducing activity against human lymphoma cells.INTRODUCTION
Macrosphelides are natural macrolides isolated from Microsphaeropsis sp. FO-5050 and Periconia byssoides, and characterized by a 16-membered tri-lactone framework (Figure 1).1 These macrolide compounds have been reported to exhibit potent cell-cell adhesion inhibitory activity on human leukemia HL-60 cells and human-umbilicalvein endothelial cells (HUVECs).2 Besides these reports, we have recently revealed that macrosphelides and analogous compounds exert apoptosis-inducing activity against human lymphoma U937 cells.3 In addition, these effects were significantly enhanced in combination with mild hyperthermia.4 Encouraged by these new findings, we have pushed a further project on design, synthesis, and biological evaluation of macrosphelides and relating compounds.5,6 In the course of our ongoing research, we found that several artificial macrosphelides (1 and 2), incorporated with some heteroaromatic side chain at the 15-position, exhibited improved apoptosis-inducing activity compared with natural macrosphelides.6 These molecular designs were inspired by extensive studies on epothilones, 16-membered natural macrolides possessing extraordinarily potent anti-tumor activity and apoptosis-inducing activity against broad tumor cell lines.7 These results prompted us to further investigate the bioactivities of heteroaromatic variants, as a part of the structure-activity relationship studies of macrosphelides. In this paper, we describe the synthetic study of three new derivatives (3, 4, and 5) based on a ring-closing metathesis (RCM) strategy, and their apoptosis-inducing activity.
RESULTS AND DISCUSSION
Thiazole side chain, found in natural epothilones, has been reported to play an important role for interacting with the target heterodimeric protein (tubulin), mainly due to action as a hydrogen-bonding acceptor.7 Therefore, the pyridine ring, possessing more basic nitrogen, can be a good surrogate of the thiazole ring, and the 2-pyridyl analogue was revealed to exhibit more potent anti-tumor activity.8 On the other hand, the position of nitrogen was decisively important; namely, corresponding 3- and 4-pyridyl analogues significantly decreased in the activity.8 We also observed that macrosphelide with the thiazole side chain (1) and the 2-pyridyl side chain (2) showed comparable level of apoptosis-inducing activity at 1 µM concentrations,6 although the mechanism of action remained unclear. For seeking a similarity of biological profiles of macrosphelides and epothilones, comparison of the bioactivities among isomeric pyridyl macrosphelides seemed to be meaningful. In addition, effects of the benzimidazolyl substitutent on the bioactivity was also investigated, in the light of recent findings that introduction of such substituents brought about positive results on anti-tumor activity of epothilones.9
Three new derivatives 3–5 were planned to be synthesized via a common synthetic pathway depicted in Scheme 1. The aldehydes 6a–c, prepared by modified procedures of reported methods,6,10 were subjected to asymmetric allylation utilizing (+)-B-allyldiisopinocampheylborane11 to give chiral homoallylalcohols 7a–c with a high enantiomeric purity,12 which was estimated by a standard Mosher’s ester analysis (> 98% ee). Dehydrative condensation of these alcohols with chiral carboxylic acid 8,6 followed by desilylation afforded alcohols 10a–c, which were subsequently conducted to the second condensation with chiral carboxylic acid 116 according to the Yamaguchi’s method13 to produce diesters 12a–c with high efficiency. Removal of the TBS group of 12a–c upon treatment with TBAF required addition of AcOH to avoid retro-Michael elimination at the C2–C3 position. Although acryloylation of the resulting alcohols 13a–c were first performed using acryloyl chloride as a reagent, this transformation resulted in considerably low yields (10–20%) probably due to quaternalization of the heteroaromatic moiety and subsequent side reactions. Thus we employed the Yamaguchi’s method again to achieve the preparation of RCM substrates 14a–c.
In our continuous studies regarding macrosphelide syntheses,6,14 the standard RCM conditions (10 mol% of 2nd Grubbs’catalyst A, CH2Cl2, rt, overnight) have worked well for macrocyclization. However, the RCM reactions of 14a and 14b were very sluggish (Table 1, entries 1 and 5), while the substrate 14c, having benzimidazole moiety, smoothly cyclized in excellent yield (98%) under these conditions. These were unexpected results for us, because the corresponding 2-pyridyl compound 2 could be efficiently synthesized under the same conditions.6 These difficulties may be attributed to relatively high Lewis basicity of the pyridine nitrogen at the less hindered positions; namely ligation effects to the ruthenium complex. Consequently, we examined the RCM cyclization of 14a and 14b, searching for optimal reaction conditions (Table 1). Gentle heating (catalyst A, CH2Cl2, reflux) increased the yield of 15a (entry 2), while Hoveyda-Grubbs catalyst B gave a poor result (entry 3). Pretreatment of the substrate with 1 equiv. of TsOH, however, significantly improved the cyclization yield up to 66% (entry 4),15 thus indicating that masking of the nitrogen lone pair by protonation was crucial for the RCM of the substrate 14a. On the other hand, the substrate 14b did not produce the desired product under the refluxing conditions (entries 6–8), although the reasons remained unclear. The MEM group of 15a and 15c was removed upon treatment with TFA, and finally alcohols 16a and 16c were subjected to Dess-Martin oxidation. Thus, the syntheses of the target compounds 3 and 5 were accomplished, except for the 4-pyridyl compound 4.
Apoptosis inducing activity of new compounds synthesized in this study was investigated, and the results of comparison between 2-pyridyl (2) and 3-pyridyl (3) derivatives are presented in Table 2. To our regret, the benzimidazolyl derivative (5) did not exhibit the activity (data not shown). The assay was performed using a human lymphoma cell line (U937), and assessment of early apoptosis and secondary necrosis was carried out by flow cytometry of annexin V/FITC and propidium iodide (PI) staining cells.6 As shown in Table 2, a great disparity was observed between the 2-pyridyl and the 3-pyridyl compounds on the apoptosis-inducing effects. For example, the 2-pyridyl compound 2 induced apoptotic cell death (ca. 9% with 18% secondary necrosis) at 20 µM concentration, while the 3-pyridyl compound 3 only gave the values of the control level at the same concentration. Although detailed mechanism of action remains unclear at present, these results suggest that the nitrogen atom on the pyridyl substituent installed at a suitable position may play an important role for interactions with a living substance controlling an apoptotic program. Further studies for elucidation of the action mechanisms of macrosphelide analogues will be performed in our laboratory.
EXPERIMENTAL
All nonaqueous reactions were carried out under an Ar atmosphere. Reagents were purchased from commercial sources and used as received. Anhydrous solvents were prepared by distillation over CaH2, or purchased from commercial sources. 1H and 13C NMR spectra were obtained on a Varian Gemini 300 instrument, using chloroform peak as an internal reference. Mass spectra were measured on a JEOL D-200 or a JEOL AX 505 mass spectrometer, and the ionization method was electron impact (EI, 70 eV). IR spectra were recorded on a JASCO FT/IR-460Plus spectrometer. Column chromatography was carried out by employing Cica Silica Gel 60N (spherical, neutral, 40-50 µm or 63-210 µm). Aldehydes 6a and 6b were prepared by Wittig reaction of commercially available 3- or 4-formylpyridines in accordance with the reported procedure.6 Aldehyde 6c was synthesized according to the reported method.10 Details of biological evaluation experiments have already been reported.6
Asymmetric Allylation of Compounds 6a–c
(+)-Ipc2B(allyl) (1.0 M pentane solution, 6.0 mL, 6 mmol) was added to a solution of the aldehyde 6 (4 mmol) in Et2O (16 mL) at –100°C, and the mixture was stirred for 1 h at –100°C. After addition of MeOH (1.6 mL), the mixture was warmed to room temperature, and then 2-aminoethanol (2.4 mL, 40 mmol) was added. After stirring for 24 h, the mixture was diluted with sat. aq. NH4Cl and extracted with CH2Cl2, dried over MgSO4, and evaporated. The residue was purified by silica gel column chromatography to afford the compounds 7a–c as colorless oil (7a, b) and colorless solid (7c). Optical purity was determined by a standard Mosher’s ester analysis (> 98% ee). 7a: Yield quant.; 1H-NMR (CDCl3): δ 8.50 (1H, s), 8.43 (1H, d, J = 4.9 Hz), 7.57 (1H, d, J = 7.7 Hz), 7.25 (1H, dd, J = 7.7, 4.9 Hz), 6.49 (1H, s), 5.91–5.77 (1H, m), 5.19 (1H, d, J = 17 Hz), 5.16 (1H, d, J = 10 Hz), 4.24 (1H, d, J = 7.4 Hz), 2.43 (2H, q, J = 7.4 Hz), 1.87 (3H, s); 13C-NMR (CDCl3): δ 149.89, 147.15, 142.27, 135.85, 134.15, 133.22, 122.97, 121.69, 118.24, 75.98, 40.18, 14.01; IR (neat): 3276 cm-1; MS (EI): m/z 189 (M+); HRMS Calcd for C12H15NO: 189.1154 (M+), found: 189.1158; [α]D24 +16.9 (c = 1.165, CHCl3). 7b: Yield 99%; 1H-NMR (CDCl3): δ 8.41 (2H, d, J = 4.5 Hz), 7.08 (2H, d, J = 4.5 Hz), 6.41 (1H, s), 5.79 (1H, ddt, J = 17, 9.9, 7.1 Hz), 5.10 (1H, d, J = 17 Hz), 5.05 (1H, d, J = 9.9 Hz), 4.59–4.25 (1H, br), 4.19 (1H, t, J = 6.3 Hz), 2.57 (2H, dd, J = 7.1, 6.3 Hz), 1.85 (3H, s); 13C-NMR (CDCl3): δ 148.81, 145.50, 144.95, 134.24, 123.52, 122.34, 117.42, 75.79, 39.97, 14.19; IR (neat): 3031 cm-1; MS (EI): m/z 189 (M+); HRMS Calcd for C12H15NO: 189.1154 (M+), found: 189.1143; [α]D25 +23.9 (c = 1.050, CHCl3). 7c: Yield 86%; mp 124–125°C; 1H-NMR (CDCl3): δ 7.62 (1H, s), 7.28 (1H, d, J = 8.2 Hz), 7.24 (1H, d, J = 8.2 Hz), 5.85–5.76 (1H, m), 5.14 (1H, dd, J = 16, 1.4 Hz), 5.09 (1H, dd, J = 10, 1.9 Hz), 4.85 (1H, t, J = 6.6 Hz), 3.71 (3H, s), 2.59–2.54 (4H, m), 2.59 (1H, brs), 2.00 (1H, br); 13C-NMR (CDCl3): δ 151.90, 141.90, 138.24, 134.86, 120.10, 117.34, 116.14, 108.42, 73.64, 44.08, 29.82, 13.66; IR (KBr): 3162, 1522 cm-1; MS (EI): m/z 216 (M+); HRMS Calcd for C13H14N2O2: 216.1038 (M+), found: 216.1025; [α]D25 +45.0 (c = 1.20, CHCl3).
Dehydrative Condensation of Alcohol 7a–c and Carboxylic Acid 8
1-Ethyl-3-(3-dimethylaminopropyl)carbodiimide Hydrochloride (EDC, 2 mmol) was added to a stirred solution of the alcohol 7 (1 mmol), the carboxylic acid 8 (2 mmol), and 4-dimethylaminopyridine (DMAP, 0.1 mmol) in CH2Cl2 (5 mL) at 0°C under Ar atmosphere. After continuous stirring at room temperature for several hours (monitored by TLC), the solvent was evaporated to leave a residue, which was triturated with ether and filtered through Celite. Evaporation of the solvent followed by chromatography on silica gel afforded the corresponding ester 9a–c as colorless oil. 9a: Yield quant.; 1H-NMR (CDCl3): δ 8.49 (1H, s), 8.44–8.43 (1H, m), 7.54 (2H, d, J = 8.0 Hz), 7.27–7.22 (1H, m), 6.45 (1H, s), 5.73 (1H, ddq, J = 17, 15, 10 Hz), 5.34 (1H, q, J = 6.9 Hz), 5.12 (1H, dd, J = 17, 1.6 Hz), 5.07 (1H, dd, J = 10, 1.6 Hz), 4.31–4.24 (1H, m), 2.54 (2H, dd, J = 15, 6.9 Hz), 2.48–2.36 (2H, m), 1.86 (3H, s), 1.19 (3H, d, J = 6.0 Hz), 0.87 (9H, s), 0.06 (3H, s), 0.04 (3H, s); 13C-NMR (CDCl3): δ 169.92, 149.58, 147.11, 137.67, 135.44, 132.72, 132.33, 123.65, 122.55, 117.45, 65.37, 44.63, 37.23, 25.53, 23.59, 17.72, 13.77, –4.72, –5.11; IR (neat): 1737 cm-1; MS (EI): m/z 389 (M+); HRMS Calcd for C22H35NO3Si: 389.2386 (M+), found: 389.2357; [α]D25 +20.8 (c = 1.115, CHCl3). 9b: Yield quant.; 1H-NMR (CDCl3): δ 8.55 (2H, d, J = 6.0 Hz), 7.16 (2H, d, J = 6.0 Hz), 6.44 (1H, s), 5.80–5.65 (1H, m), 5.72 (1H, t, J = 6.6 Hz), 5.12 (1H, d, J = 17 Hz), 5.09 (1H, d, J = 10 Hz), 4.25–4.33 (1H, m), 2.48–2.36 (4H, m), 1.90 (3H, s), 1.20 (3H, d, J = 6.0 Hz), 0.87 (9H, s), 0.07 (3H, s), 0.05 (3H, s); 13C-NMR (CDCl3): δ 170.08, 148.76, 144.81, 139.94, 132.67, 124.70, 123.47, 117.72, 77.42, 65.50, 44.71, 37.33, 25.65, 23.71, 17.86, 14.19, –4.59, –5.00; IR (neat): 1735 cm-1; MS (EI): m/z 389 (M+); HRMS Calcd for C22H35NO3Si: 389.2386 (M+), found: 389.2394; [α]D24 +16.1 (c = 0.94, CHCl3). 9c: Yield 97%; 1H-NMR (CDCl3): δ 7.64 (1H, s), 7.27 (1H, d, J = 7.4 Hz), 7.22 (1H, d, J = 7.4 Hz), 5.90 (1H, t, J = 6.9 Hz), 5.72–5.61 (1H, m), 5.05 (1H, dd, J = 16, 1.4 Hz), 5.00 (1H, dd, J = 10, 1.9 Hz), 4.24–4.20 (1H, m), 3.71 (3H, s), 2.60 (3H, s), 2.74–2.31 (4H, m), 1.14 (3H, d, J = 6.0 Hz), 0.86 (9H, s), 0.04 (3H, s), 0.01 (3H, s); 13C-NMR (CDCl3): δ 170.45, 152.15, 142.07, 135.20, 133.81, 133.30, 120.96, 117.66, 116.75, 108.45, 75.53, 65.63, 44.98, 41.11, 29.82, 25.76, 23.83, 13.71, –4.58, –4.91; IR (neat): 1732 cm-1; MS (EI): m/z 416 (M+); HRMS Calcd for C23H36N2O3Si: 416.2464 (M+), found: 416.2495; [α]D26 +38.5 (c = 1.70, CHCl3).
Desilylation of TBS Ether 9a–c
A 1 M solution of tetra-n-butylammonium fluoride (TBAF) in THF (2 mL, 2 mmol) was added to a stirred solution of the TBS ether 9 (1 mmol) in THF (2 mL) at room temperature under Ar atmosphere, and the mixture was stirred for several hours at room temperature (monitored by TLC). The solvent was evaporated off to leave a residue, which was dissolved in AcOEt and the resulting organic layer was washed with water and brine, and dried over MgSO4. Evaporation of the solvent left a residue, which was chromatographed on silica gel to give the corresponding alcohol 10a–c as colorless oil. 10a: Yield 69%; 1H-NMR (CDCl3): δ 8.50 (1H, s), 8.43 (1H, d, J = 4.8 Hz), 7.60 (1H, d, J = 7.7 Hz), 7.30–7.25 (1H, m), 6.45 (1H, s), 5.80–5.65 (1H, m), 5.30 (1H, t, J = 6.7 Hz), 5.15 (1H, d, J = 18 Hz), 5.09 (1H, d, J = 10 Hz), 4.16–4.09 (1H, m), 2.60–2.45 (4H, m), 1.88 (3H, s), 1.25 (3H, d, J = 6.3 Hz); 13C-NMR (CDCl3): δ 170.97, 149.31, 146.90, 135.67, 132.63, 132.38, 123.48, 122.68, 117.58, 77.62, 77.19, 63.81, 43.31, 37.17, 22.60, 13.79; IR (neat): 3309, 2974, 1733 cm-1; MS (EI): m/z 275 (M+); HRMS Calcd for C16H21NO3: 275.1521 (M+), found: 275.1513; [α]D26 +34.6 (c = 1.06, CHCl3). 10b: Yield 87%; 1H-NMR (CDCl3): δ 8.38 (2H, d, J = 5.9 Hz), 7.01 (2H, d, J = 5.9 Hz), 6.32 (1H, s), 5.70–5.55 (1H, m), 5.24 (1H, t, J = 6.9 Hz), 5.00 (1H, d, J = 17 Hz), 4.96 (1H, d, J = 10 Hz), 4.30–4.19 (1H, br), 4.19–4.09 (1H, m), 2.47–2.27 (4H, m), 1.77 (3H, s), 1.13 (3H, d, J = 6.3 Hz); 13C-NMR (CDCl3): δ 170.92, 148.85, 144.42, 139.58, 132.53, 124.52, 123.32, 117.68, 63.80, 43.38, 37.15, 22.69, 14.08; IR (neat): 3010 cm-1; MS (EI): m/z 275 (M+); HRMS Calcd for C16H21NO3: 275.1521 (M+), found: 275.1499; [α]D26 +28.1 (c = 1.00, CHCl3). 10c: Yield 83%; 1H-NMR (CDCl3): δ 7.65 (1H, s), 7.26 (1H, d, J = 9.3 Hz), 7.25 (1H, d, J = 9.3 Hz), 5.95 (1H, t, J = 6.0 Hz), 5.75–5.63 (1H, m), 5.09 (1H, dd, J = 16, 1.4 Hz), 5.04 (1H, dd, J = 10, 1.9 Hz), 4.12–4.09 (1H, m), 3.71 (3H, s), 3.00 (1H, br), 2.60 (3H, s), 2.75–2.46 (4H, m), 1.19 (3H, d, J = 6.3 Hz); 13C-NMR (CDCl3): δ 171.76, 152.32, 142.20, 135.33, 133.53, 133.21, 120.80, 117.85, 116.67, 108.58, 75.93, 64.04, 43.15, 41.02, 29.87, 22.49, 13.75; IR (neat): 3389, 1731, 1519 cm-1; MS (EI): m/z 302 (M+); HRMS Calcd for C17H22N2O3: 302.1630 (M+), found: 302.1631; [α]D25 +65.2 (c = 1.00, CHCl3).
Esterification of Alcohol 10a–c with Carboxylic Acid 11
To a solution of the carboxylic acid 11 (1.2 mmol) and Et3N (1.5 mmol) in toluene (10 mL) was added 2,4,6-trichlorobenzoyl chloride (1.2 mmol) at room temperature under Ar atmosphere, and the resulting mixture was stirred at room temperature for 0.5 h. The alcohol 10 (1 mmol) and DMAP (1 mmol) were added, and the reaction mixture was stirred for several hours. After the reaction completed (by TLC), the mixture was diluted with benzene, and washed with sat. aq. NaHCO3 and brine, and dried over MgSO4. The solvent was evaporated off to leave a residue, which was chromatographed on silica gel to afford the corresponding ester 12a–c as colorless oil. 12a: Yield 78%; 1H-NMR (CDCl3): δ 8.69–8.51 (1H, br), 8.50–8.45 (1H, br), 7.60 (1H, d, J = 7.7 Hz), 7.35–7.25 (1H, m), 6.83 (1H, dd, J = 16, 6.3 Hz), 6.45 (1H, s), 5.95 (1H, d, J = 16 Hz), 5.79–5.65 (1H, m), 5.49–5.30 (2H, m), 5.13 (1H, d, J = 18 Hz), 5.09 (1H, d, J = 10 Hz), 4.72–4.66 (2H, m), 4.03 (1H, t, J = 5.5 Hz), 3.89–3.73 (3H, m), 3.66–3.51 (2H, m), 3.38 (3H, s), 2.76 (1H, dd, J = 16, 6.9 Hz), 2.55–2.50 (2H, m), 1.86 (3H, s), 1.33 (3H, d, J = 6.3 Hz), 1.14 (3H, d, J = 6.3 Hz), 0.86 (9H, s), 0.04 (3H, s), 0.02 (3H, s); 13C-NMR (CDCl3): δ 168.98, 164.84, 149.61, 147.24, 145.84, 137.84, 136.06, 132.92, 132.80, 124.00, 123.14, 117.97, 93.84, 79.85, 79.77, 78.13, 71.60, 70.51, 67.31, 67.17, 58.99, 41.07, 37.46, 25.84, 25.79, 19.94, 18.07, 14.03, –4.51, –4.71; IR (neat): 1722 cm-1; MS (EI): m/z 605 (M+); HRMS Calcd for C32H51NO8Si: 605.3384 (M+), found: 605.3399; [α]D26 –19.6 (c = 0.97, CHCl3). 12b: Yield quant.; 1H-NMR (CDCl3): δ 8.60–8.52 (2H, br), 7.12 (2H, d, J = 4.9 Hz), 6.80 (1H, dd, J = 16, 6.3 Hz), 6.39 (1H, s), 5.93 (1H, d, J = 16 Hz), 5.69 (1H, ddd, J = 17, 10, 7.1 Hz), 5.38–5.28 (2H, m), 5.11 (1H, d, J = 17 Hz), 5.07 (1H, d, J = 10 Hz), 4.73–4.64 (2H, m), 4.02–3.99 (1H, m), 3.80–3.58 (2H, m), 3.52–3.49 (3H, m), 3.35 (3H, s), 2.73 (1H, dd, J = 15, 7.1 Hz), 2.54 (1H, dd, J = 16, 7.1 Hz), 2.50–2.44 (2H, m), 1.85 (3H, s), 1.31 (3H, d, J = 6.3 Hz), 1.12 (3H, d, J = 6.3 Hz), 0.84 (9H, s), 0.02 (3H, s), 0.00 (3H, s); 13C-NMR (CDCl3): δ 168.93, 164.82, 149.31, 145.88, 144.58, 139.60, 132.67, 125.09, 123.57, 123.11, 118.06, 93.81, 79.73, 77.92, 71.60, 70.50, 67.28, 67.16, 58.99, 41.05, 37.41, 25.79, 19.95, 18.06, 14.29, –4.51, –4.72; IR (neat): 1732, 1717 cm-1; MS (EI): m/z 605 (M+); HRMS Calcd for C32H51NO8Si: 605.3384 (M+), found: 605.3413; [α]D25 –16.3 (c = 1.295, CHCl3). 12c: Yield 72%; 1H-NMR (CDCl3): δ 7.63 (1H, s), 7.29 (1H, d, J = 9.3 Hz), 7.24 (1H, d, J = 9.3 Hz), 6.85 (1H, dd, J = 13, 6.3 Hz), 5.94 (1H, t, J = 6.7 Hz), 5.90 (1H, dd, J = 16, 1.4 Hz), 5.74–5.60 (1H, m), 5.33–5.30 (1H, m), 5.06 (1H, dd, J = 15, 1.4 Hz), 5.03 (1H, dd, J = 10, 1.9 Hz), 4.76–4.62 (2H, m), 4.03 (1H, t, J = 5.2 Hz), 3.71 (3H, s), 3.38 (3H, s), 3.89–3.60 (3H, m), 3.59–3.51 (2H, m), 2.60 (3H, s), 2.78–2.46 (4H, m), 1.28 (3H, d, J = 6.3 Hz), 1.15 (3H, d, J = 6.3 Hz), 0.86 (9H, s), 0.04 (3H, s), 0.02 (3H, s); 13C-NMR (CDCl3): δ 169.16, 164.86, 152.32, 145.67, 133.61, 133.22, 123.23, 120.98, 117.89, 116.88, 108.62, 93.88, 79.82, 75.98, 71.65, 70.54, 69.87, 67.42, 67.16, 58.99, 53.43, 41.07, 41.03, 29.95, 29.74, 25.82, 19.92, 18.09, 13.82, –4.50, –4.69; IR (neat): 1713, 1643 cm-1; MS (EI): m/z 632 (M+); HRMS Calcd for C33H52N2O8Si: 632.3538 (M+), found: 632.3493; [α]D25 +8.9 (c = 1.43, CHCl3).
Desilylation of TBS Ether 12a–c
A 1 M solution of TBAF in THF (4 mL, 4 mmol) was added to a stirred solution of the TBS ether 12 (0.2 mmol) and acetic acid (0.3 mL, 5.2 mmol) in THF (1.2 mL) at room temperature under Ar atmosphere, and the mixture was stirred for 1 to 3 days at room temperature. The ethereal solution of the residue resulting from the evaporation of the solvent was washed with water, sat. aq. NaHCO3, and brine, and dried over MgSO4. Evaporation of the solvent left a residue, which was chromatographed on silica gel to give the corresponding alcohol 13a–c as colorless oil. 13a: Yield 86%; 1H-NMR (CDCl3): δ 8.50–8.49 (1H, br), 8.47–8.40 (1H, br), 7.55 (1H, d, J = 7.6 Hz), 7.25 (1H, dd, J = 7.6, 4.9 Hz), 6.82 (1H, dd, J = 16, 6.3 Hz), 6.43 (1H, s), 5.99 (1H, d, J = 16 Hz), 5.71 (1H, ddd, J = 17, 8.4, 7.1 Hz), 5.43–5.33 (2H, m), 5.12 (1H, d, J = 8.4 Hz), 5.08 (1H, d, J = 8.4 Hz), 4.74–4.65 (2H, m), 4.17–4.14 (1H, m), 3.92–3.56 (3H, m), 3.55–3.52 (2H, m), 3.37 (3H, s), 3.01–2.91 (1H, br), 2.73 (1H, dd, J = 17, 7.7 Hz), 2.57 (1H, dd, J = 15, 5.5 Hz), 2.46 (2H, dd, J = 11, 7.1 Hz), 1.84 (3H, s), 1.33 (3H, d, J = 6.3 Hz), 1.11 (3H, d, J = 6.6 Hz); 13C-NMR (CDCl3): δ 168.96, 164.74, 149.74, 147.30, 144.15, 137.84, 135.93, 132.82, 123.95, 123.58, 122.93, 117.95, 94.28, 80.80, 78.12, 71.58, 68.93, 68.85, 67.57, 67.44, 58.92, 41.13, 37.43, 20.00, 17.80, 14.08; IR (neat): 3422, 1719 cm-1; MS (EI): m/z 491 (M+); HRMS Calcd for C26H37NO8: 491.2519 (M+), found: 491.2483; [α]D25 –18.7 (c = 0.76, CHCl3). 13b: Yield 83%; 1H-NMR (CDCl3): δ 8.52 (2H, d, J = 4.9 Hz), 7.12 (2H, d, J = 4.9 Hz), 6.81 (1H, dd, J = 16, 6.0 Hz), 6.39 (1H, s), 5.97 (1H, d, J = 16 Hz), 5.74–5.63 (1H, m), 5.38–5.30 (2H, m), 5.11 (1H, d, J = 9.3 Hz), 5.09 (1H, d, J = 9.3 Hz), 4.70–4.68 (2H, m), 4.63–4.14 (1H, m), 3.91–3.88 (1H, m), 3.66–3.60 (2H, m), 3.54–3.51 (2H, m), 3.36 (3H, s), 2.73 (1H, dd, J = 16, 7.4 Hz), 2.56 (1H, dd, J = 15, 7.4 Hz), 2.49–2.44 (2H, m), 1.85 (3H, s), 1.32 (3H, d, J = 6.3 Hz), 1.09 (3H, d, J = 6.6 Hz); 13C-NMR (CDCl3): δ 168.95, 164.73, 149.29, 144.61, 144.03, 139.65, 132.67, 125.07, 123.57, 123.48, 118.06, 94.31, 80.75, 77.96, 71.58, 68.88, 67.50, 58.94, 41.08, 37.41, 19.97, 17.69, 14.29; IR (neat): 3441, 1719 cm-1; MS (EI): m/z 491 (M+); HRMS Calcd for C26H37NO8: 491.2519 (M+), found: 491.2522; [α]D24 –32.8 (c = 1.235, CHCl3). 13c: Yield 56%; 1H-NMR (CDCl3): δ 7.70 (1H, s), 7.32 (1H, d, J = 8.2 Hz), 7.28 (1H, d, J = 8.2 Hz), 6.83 (1H, dd, J = 16, 6.4 Hz), 5.93 (1H, dd, J = 16, 1.4 Hz), 5.91 (1H, t, J = 6.9 Hz), 5.66–5.64 (1H, m), 5.38–5.35 (1H, m), 5.05 (1H, dd, J = 17, 1.6 Hz), 5.02 (1H, dd, J = 10, 1.1 Hz), 4.72–4.59 (2H, m), 4.20 (1H, br), 4.14 (1H, t, J = 4.4 Hz), 3.78 (3H, s), 3.38 (3H, s), 3.89–3.52 (5H, m), 2.75 (3H, s), 2.69–2.54 (4H, m), 1.28 (3H, d, J = 6.3 Hz), 1.14 (3H, d, J = 6.3 Hz); 13C-NMR (CDCl3): δ 168.96, 164.65, 144.61, 135.38, 133.66, 132.69, 123.18, 122.21, 118.00, 114.94, 109.52, 93.92, 80.30, 75.50, 71.43, 68.62, 67.18, 67.15, 58.72, 53.35, 52.63, 40.84, 40.74, 30.19, 19.76, 18.28, 12.57; IR (neat): 3424, 1731, 1652 cm-1; MS (EI): m/z 518 (M+); HRMS Calcd for C27H38N2O8: 518.2618 (M+), found: 518.2628; [α]D24 +7.8 (c = 1.64, CHCl3).
Acryloylation of Alcohol 13a–c
In accordance with the synthesis of the compounds 12, acrylic acid (0.8 mmol) and the alcohol 13a–c (0.2 mmol) gave the ester 14a–c as colorless oil. 14a: Yield 95%; 1H-NMR (CDCl3): δ 8.51 (1H, s), 8.48 (1H, d, J = 4.6 Hz), 7.60 (1H, d, J = 8.0 Hz), 7.29 (1H, dd, J = 8.0, 4.6 Hz), 6.81 (1H, dd, J = 16, 6.0 Hz), 6.45 (1H, s), 6.40 (1H, d, J = 17 Hz), 6.16 (1H, dd, J = 17, 10 Hz), 6.03 (1H, d, J = 16 Hz), 5.83 (1H, d, J = 10 Hz), 5.73 (1H, ddt, J = 17, 10, 8.2 Hz), 5.42–5.34 (1H, m), 5.37 (1H, t, J = 6.7 Hz), 5.13 (1H, d, J = 8.4 Hz), 5.09 (1H, d, J = 8.4 Hz), 4.79–4.72 (1H, m), 4.72–4.66 (2H, m), 4.40–4.36 (1H, m), 3.81–3.59 (2H, m), 3.54–3.51 (2H, m), 3.37 (3H, s), 2.76 (1H, dd, J = 15, 7.5 Hz), 2.57 (1H, dd, J = 15, 5.9 Hz), 2.54–2.48 (2H, m), 1.86 (3H, s), 1.35 (3H, d, J = 6.3 Hz), 1.22 (3H, d, J = 6.6 Hz); 13C-NMR (CDCl3): δ 169.01, 165.18, 164.66, 149.06, 146.73, 143.71, 143.43, 138.26, 136.61, 132.77, 130.99, 128.27, 124.02, 123.79, 118.10, 93.75, 78.15, 71.58, 71.40, 67.66, 67.26, 67.21, 59.04, 41.10, 37.49, 29.76, 20.00, 14.98, 14.11; IR (neat): 2934, 1723 cm-1; MS (EI): m/z 545 (M+); HRMS Calcd for C29H39NO9: 545.2625 (M+), found: 545.6213; [α]D25 –23.3 (c = 0.735, CHCl3). 14b: Yield 98%; 1H-NMR (CDCl3): δ 8.61–8.50 (2H, br), 7.16 (2H, d, J = 4.1 Hz), 6.77 (1H, dd, J = 15, 6.0 Hz), 6.41 (1H, s), 6.40 (1H, d, J = 17 Hz), 6.10–6.00 (2H, m), 5.83 (1H, d, J = 10 Hz), 5.80–5.65 (1H, m), 5.40–5.29 (2H, m), 5.13–5.05 (3H, m), 4.72–4.64 (2H, m), 4.39–4.25 (3H, m), 3.81–3.56 (2H, m), 3.54–3.51 (2H, m), 3.37 (3H, s), 2.79–2.40 (2H, m), 1.87 (3H, s), 1.34 (3H, d, J = 6.0 Hz), 1.18 (3H, d, J = 6.3 Hz); 13C-NMR (CDCl3): δ 168.95, 164.60, 157.23, 154.76, 149.06, 145.54, 143.27, 139.96, 132.67, 131.04, 128.27, 127.95, 125.05, 124.00, 118.15, 100.56, 93.73, 77.97, 71.58, 67.63, 67.31, 59.82, 59.04, 41.07, 37.46, 33.75, 20.00, 15.07, 14.37; IR (neat): 1734, 1717 cm-1; MS (EI): m/z 545 (M+); HRMS Calcd for C29H39NO9: 545.2625 (M+), found: 545.6208; [α]D25 –23.3 (c = 0.735, CHCl3). 14c: Yield 80%; 1H-NMR (CDCl3): δ 7.67 (1H, s), 7.27 (1H, d, J = 6.9 Hz), 7.24 (1H, d, J = 6.9 Hz), 6.76 (1H, dd, J = 16, 6.2 Hz), 6.39 (1H, d, J = 17 Hz), 6.01 (1H, t, J = 10 Hz), 6.14–5.90 (2H, m), 5.83 (1H, dd, J = 10, 1.4 Hz), 5.71–5.61 (1H, m), 5.34–5.30 (1H, m), 5.05 (1H, t, J = 6.3 Hz), 5.02 (1H, t, J = 8.2 Hz), 4.71–4.58 (2H, m), 4.38 (1H, t, J = 5.6 Hz), 3.74 (3H, s), 3.36 (3H, s), 3.79–3.50 (5H, m), 2.66 (3H, s), 2.78–2.49 (4H, m), 1.29 (3H, d, J = 6.3 Hz), 1.19 (3H, d, J = 6.6 Hz); 13C-NMR (CDCl3): δ 169.08, 165.21, 164.63, 153.79, 150.63, 143.32, 138.81, 132.12, 131.02, 128.27, 124.07, 118.76, 113.60, 110.41, 100.57, 93.67, 73.67, 71.60, 71.48, 67.58, 67.20, 65.87, 59.03, 41.02, 40.87, 30.94, 29.77, 20.02, 15.39; IR (neat): 1723 cm-1; MS (EI): m/z 572 (M+); HRMS Calcd for C30H40N2O9: 572.2734 (M+), found: 572.2684; [α]D24 +10.5 (c = 0.65, CHCl3).
RCM of Substrates 14a–c
Grubbs’ ruthenium catalyst (second generation) (10 µmol) was added to a solution of substrate 14 (0.1 mmol) in CH2Cl2 (100 mL) under Ar atmosphere. For the substrate 14a, pretreatment with TsOH hydrate (0.1 mmol) was performed in refluxing CH2Cl2 for 0.5 h. After reflux for 24 h on an oil bath, the solvent was evaporated to afford a residue, which was chromatographed on silica gel to give the compound 15a and 15c as colorless oil. 15a: Yield 66%; 1H-NMR (CDCl3): δ 8.70–8.59 (2H, br), 8.14–8.09 (1H, br), 7.82–7.75 (1H, br), 6.78 (1H, dd, J = 16, 7.4 Hz), 6.73 (1H, dd, J = 16, 7.4 Hz), 6.59 (1H, s), 5.95 (1H, d, J = 16 Hz), 5.87 (1H, d, J = 16 Hz), 5.52–5.49 (1H, m), 5.34–5.24 (1H, m), 4.98–4.90 (1H, m), 4.77–4.71 (2H, m), 4.15–4.10 (1H, m), 3.81–3.65 (2H, m), 3.57–3.54 (2H, m), 3.39 (3H, s), 2.79–2.54 (4H, m), 1.95 (3H, s), 1.41 (3H, d, J = 6.0 Hz), 1.34 (3H, d, J = 6.3 Hz); 13C-NMR (CDCl3): δ 169.42, 168.88, 164.08, 151.31, 144.92, 143.64, 136.60, 131.04, 129.84, 128.10, 125.36, 124.31, 118.45, 94.02, 78.72, 71.55, 67.78, 67.39, 67.31, 59.03, 41.36, 37.12, 29.74, 19.79, 15.10, 14.19; IR (neat): 1722 cm-1; MS (EI): m/z 517 (M+); HRMS Calcd for C27H35NO9: 517.2311 (M+), found: 517.2345. 15c: Yield 98%; 1H-NMR (CDCl3): δ 7.70 (1H, s), 7.30 (1H, d, J = 7.1 Hz), 7.27 (1H, d, J = 7.1 Hz), 6.90–6.75 (2H, m), 6.05 (1H, d, J = 17 Hz), 6.01–5.95 (1H, m), 5.83 (1H, d, J = 17 Hz), 5.38–5.30 (1H, m), 5.15 (1H, q, J = 6.9 Hz), 4.77–4.65 (2H, m), 4.45–4.20 (1H, m), 3.77 (3H, s), 3.70–3.55 (4H, m), 3.39 (3H, s), 2.80–2.49 (4H, m), 1.35 (3H, d, J = 6.3 Hz), 1.28 (3H, d, J = 6.3 Hz); 13C-NMR (CDCl3): δ 169.92, 169.39, 165.31, 164.42, 144.66, 143.26, 142.48, 125.12, 124.29, 123.52, 120.72, 109.05, 93.91, 93.16, 74.17, 71.48, 71.22, 67.70, 67.16, 59.79, 58.91, 40.77, 34.64, 30.10, 29.22, 20.08, 16.80, 13.50; IR (neat): 1731, 1652 cm-1; MS (EI): m/z 544 (M+); HRMS Calcd for C28H36N2O9: 544.2452 (M+), found: 544.2421; [α]D24 –13.4 (c = 1.94, CHCl3).
Removal of the MEM Group of Compounds 15a and 15c
Trifluoroacetic acid (TFA, 1 mL) was added to a solution of the MEM ether 15 (0.1 mmol) in CH2Cl2 (1 mL) at 0°C under Ar atmosphere. After continuous stirring for 24 h at room temperature, the reaction mixture was diluted with CH2Cl2 and washed by sat. aq. NaHCO3, and then dried over MgSO4. The solvent was evaporated to afford a residue, which was chromatographed on silica gel to give the alcohol 16a and 16c as colorless oil. 16a: Yield 89%; 1H-NMR (CDCl3): δ 8.79–8.42 (2H, br), 8.15 (1H, br), 7.79–7.65 (1H, br), 6.87 (1H, dd, J = 16, 9.3 Hz), 6.84 (1H, dd, J = 16, 6.3 Hz), 6.66 (1H, s), 5.92 (2H, d, J = 16 Hz), 5.88–5.57 (1H, m), 5.39–5.32 (1H, m), 4.91–4.83 (1H, m), 4.50–4.21 (1H, br), 4.15–4.07 (1H, m), 2.83–2.64 (4H, m), 1.96 (3H, s), 1.40 (3H, d, J = 6.3 Hz), 1.30 (3H, d, J = 6.3 Hz); 13C-NMR (CDCl3): δ 171.27, 166.46, 149.02, 138.38, 129.17, 124.53, 123.22, 122.76, 109.48, 102.04, 78.38, 75.16, 73.58, 69.26, 61.36, 47.71, 41.82, 37.98, 20.07, 18.08, 14.51; IR (neat): 3446, 1726 cm-1; MS (EI): m/z 429 (M+); HRMS Calcd for C23H27NO7: 429.1787 (M+), found: 429.1804; [α]D24 +34.5 (c = 0.31, MeOH). 16c: Yield 84%; 1H-NMR (CDCl3): δ 7.77 (1H, s), 7.44 (1H, d, J = 7.1 Hz), 7.23 (1H, d, J = 7.1 Hz), 6.88 (1H, dd, J = 16, 5.2 Hz), 6.04 (1H, t, J = 5.5 Hz), 5.89 (1H, dd, J = 16, 1.4 Hz), 5.50–5.34 (1H, m), 4.91 (1H, t, J = 6.1 Hz), 4.43 (1H, dd, J = 8.2, 7.7 Hz), 4.23 (1H, t, J = 5.2 Hz), 3.79 (3H, s), 3.79–3.64 (1H, m), 2.72–2.26 (4H, m), 2.63 (3H, s), 1.39 (3H, d, J = 6.3 Hz), 1.22 (3H, d, J = 6.3 Hz), 0.97 (1H, br); 13C-NMR (CDCl3): δ 169.16, 168.72, 164.95, 164.21, 164.09, 145.39, 143.37, 123.73, 122.63, 122.03, 121.54, 110.36, 75.90, 74.15, 73.64, 70.11, 67.15, 59.38, 53.36, 31.37, 29.32, 28.88, 19.61, 17.67; IR (neat): 3351, 1717, 1652 cm-1; MS (EI): m/z 456 (M+); HRMS Calcd for C24H28N2O7: 456.1897 (M+), found: 456.1873; [α]D25 +25.6 (c = 0.88, CHCl3).
DMP Oxidation of Alcohol 16a and 16c (Synthesis of Macrosphelide Analogues 3 and 5)
To a solution of the alcohol 16 (0.1 mmol) in CH2Cl2 (2 mL) was added Dess-Martin periodinane (DMP, 0.2 mmol), and the mixture was stirred for several hours at room temperature (monitored by TLC). The reaction was quenched with sat. aq. NaHCO3, and the mixture was extracted with CH2Cl2. The organic layer was dried over MgSO4, filtered, and concentrated in vacuo. The residue was purified by column chromatography on silica gel to afford the compounds 3 (colorless oil) and 5 (colorless solid). 3: Yield quant.; 1H-NMR (CDCl3): δ 8.01 (1H, d, J = 8.0 Hz), 7.79 (1H, d, J = 9.3 Hz), 7.45 (1H, dd, J = 9.3, 8.0 Hz), 7.26 (1H, d, J = 16 Hz), 7.06 (1H, dd, J = 15, 8.2 Hz), 6.92 (1H, s), 6.66 (1H, s), 6.57 (1H, d, J = 16 Hz), 6.13 (1H, d, J = 15 Hz), 5.63 (1H, t, J = 4.6 Hz), 5.42–5.32 (1H, m), 5.05 (1H, q, J = 7.1 Hz), 2.82–2.73 (4H, m), 2.22 (3H, s), 1.48 (3H, d, J = 7.1 Hz), 1.45 (3H, d, J = 6.9 Hz); 13C-NMR (CDCl3): δ 176.95, 171.93, 170.10, 168.08, 151.76, 139.88, 129.85, 127.55, 125.98, 124.04, 123.51, 117.65, 109.29, 102.04, 98.70, 78.03, 70.16, 66.96, 55.49, 41.28, 37.97, 16.84, 14.51; IR (neat): 1718, 1704 cm-1; MS (EI): m/z 427 (M+); HRMS Calcd for C23H25NO7: 427.1631 (M+), found: 427.1647; [α]D24 +15.0 (c = 0.315, MeOH). 5: Yield 75%; mp 128–130°C; 1H-NMR (CDCl3): δ 7.78 (1H, s), 7.35–7.26 (3H, m), 7.09–7.05 (1H, m), 6.69 (1H, d, J = 16 Hz), 6.15–6.10 (1H, m), 6.11 (1H, d, J = 16 Hz), 5.38–5.31 (1H, m), 5.16 (1H, q, J = 7.1 Hz), 3.75 (3H, s), 2.80–2.70 (4H, m), 2.18 (3H, s), 1.52 (3H, d, J = 7.1 Hz), 1.33 (3H, d, J = 6.3 Hz); 13C-NMR (CDCl3): δ 197.51, 168.79, 164.60, 162.95, 145.55, 140.51, 133.81, 132.30, 131.49, 130.34, 127.32, 122.64, 121.01, 108.81, 75.03, 74.07, 68.47, 40.45, 29.72, 29.32, 28.87, 19.24, 16.54; IR (KBr): 1725, 1614 cm-1; MS (EI): m/z 454 (M+); HRMS Calcd for C24H26N2O7: 454.1740 (M+), found: 454.1717; [α]D25 +20.9 (c = 0.82, MeOH).
ACKNOWLEDGEMENTS
This work was supported by THE FUGAKU TRUST FOR MEDICINAL RESEARCH, and by Grant-in-Aid for Scientific Research (C) (No. 19590098) from Japan Society for the Promotion of Science (JSPS).
References
1. M. Hayashi, Y.-P. Kim, H. Hiraoka, M. Natori, S. Takamatsu, T. Kawakubo, R. Masuma, K. Komiyama, and S. Ômura, J. Antibiot., 1995, 48, 1435; T. Sunazuka, T. Hirose, Y. Harigaya, S. Takamatsu, M. Hayashi, K. Komiyama, S. Ômura, P. A. Sprengeler, and A. B. Smith III, J. Am. Chem. Soc., 1997, 119, 10247; CrossRef T. Yamada, M. Iritani, M. Doi, K. Minoura, T. Ito, and A. Numata, J. Chem. Soc., Perkin Trans. 1, 2001, 3046; CrossRef For a review; Y. Matsuya and H. Nemoto, Heterocycles, 2005, 65, 1741, and references cited therein. CrossRef
2. S. Takamatsu, Y.-P. Kim, M. Hayashi, H. Hiraoka, M. Natori, K. Komiyama, and S. Ômura, J. Antibiot., 1996, 49, 95.
3. K. Ahmed, Q.-L. Zhao, Y. Matsuya, D.-Y. Yu, L. B. Feril Jr., H. Nemoto, and T. Kondo, Chem. Biol. Interact., 2007, 170, 86. CrossRef
4. K. Ahmed, Q.-L. Zhao, Y. Matsuya, D.-Y. Yu, T. L. Salunga, H. Nemoto, and T. Kondo, Int. J. Hyperthermia, 2007, 23, 353. CrossRef
5. Y. Matsuya, T. Matsushita, K. Sakamoto, and H. Nemoto, Heterocycles, 2009, 77, 483; CrossRef K. Ahmed, Y. Matsuya, H. Nemoto, S. F. H. Zaidi, T. Sugiyama, Y. Yoshihisa, T. Shimizu, and T. Kondo, Chem. Biol. Interact., 2009, 177, 218. CrossRef
6. Y. Matsuya, T. Kawaguchi, K. Ishihara, K. Ahmed, Q.-L. Zhao, T. Kondo, and H. Nemoto, Org. Lett., 2006, 8, 4609; CrossRef Y. Matsuya, Y. Kobayashi, T. Kawaguchi, A. Hori, Y. Watanabe, K. Ishihara, K. Ahmed, Z.-L. Wei, D.-Y. Yu, Q.-L. Zhao, T. Kondo, and H. Nemoto, Chem. Eur. J., 2009, 15, 5799. CrossRef
7. For recent review, K. C. Nicolaou, F. Roschangar, and D. Vourloumis, Angew. Chem. Int. Ed., 1998, 37, 2014; CrossRef K.-H. Altmann, M. Wartmann, and T. O’Reilly, Biochim. Biophys. Acta, 2000, 1470, M79.
8. K. C. Nicolaou, R. Scarpelli, B. Bollbuck, B. Werschkun, M. M. A. Pereira, M. Wartmann, K.-H. Altmann, D. Zaharevitz, R. Gussio, and P. Giannakakou, Chem. Biol., 2000, 7, 593. CrossRef
9. K.-H. Altmann, G. Bold, G. Caravatti, A. Flörsheimer, V. Guagnano, and M. Wartmann, Bioorg. Med. Chem. Lett., 2000, 10, 2765; CrossRef F. Cachoux, T. Isarno, M. Wartmann, and K.-H. Altmann, Angew. Chem. Int. Ed., 2005, 44, 7469; F. Cachoux, T. Isarno, M. Wartmann, and K.-H. Altmann, ChemBioChem, 2006, 7, 54. CrossRef
10. K.-H. Altmann, G. Bold, G. Caravatti, and A. Flörsheimer, PCT Int. Appl. WO2000037473.
11. For a review, P. V. Ramachandran, Aldrichimica Acta, 2002, 35, 23.
12. Absolute configurations were deduced from the empirical rule (see ref. 11).
13. J. Inanaga, K. Hirata, H. Saeki, T. Katsuki, and M. Yamaguchi, Bull. Chem. Soc. Jpn., 1979, 52, 1989. CrossRef
14. Y. Matsuya, T. Kawaguchi, and H. Nemoto, Org. Lett., 2003, 5, 2939; CrossRef T. Kawaguchi, N. Funamori, Y. Matsuya, and H. Nemoto, J. Org. Chem., 2004, 69, 505. CrossRef
15. V. Gracias, A. F. Gasiecki, and S. W. Djuric, Org. Lett., 2005, 7, 3183; CrossRef Y. Chen, H. V. R. Dias, and C. J. Lovely, Tetrahedron Lett., 2003, 44, 1379. CrossRef