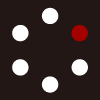
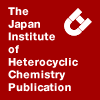
HETEROCYCLES
An International Journal for Reviews and Communications in Heterocyclic ChemistryWeb Edition ISSN: 1881-0942
Published online by The Japan Institute of Heterocyclic Chemistry
e-Journal
Full Text HTML
Received, 31st July, 2009, Accepted, 18th September, 2009, Published online, 18th September, 2009.
DOI: 10.3987/COM-09-S(S)98
■ Synthesis of Tetrabenzoporphyrins Fused with Fluoranthenes
Jun Nakamura, Tetsuo Okujima,* Yuya Tomimori, Naoki Komobuchi, Hiroko Yamada, Hidemitsu Uno, and Noboru Ono
Department of Chemistry and Biology, Graduate School or Science and Engineering, Ehime University, Matsuyama 790-8577, Japan
Abstract
Tetrabenzoporphyrins fused with fluoranthenes were prepared by the retro Diels-Alder reaction of the precursors composed of a porphyrin and four fluoranthenes connected with bicyclo[2.2.2]octadiene (BCOD). These porphyrins exhibited the Soret (480–494 nm, ε = 243,000–277,000) and intense Q bands (739–760 nm, ε = 375,000–509,000). Free bases and Zn complexes of them fluoresce at 760 nm and 746 nm with small Storks shifts and high quantum yields of 30 and 10 %, respectively.INTRODUCTION
Linearly π-expanded porphyrins have attracted the interest of many research groups for applications such as light emitting displays, thin film transistor, solar cells, photosensitizers for photodynamic therapy (PDT), or other opt-electronic materials.1 One of the simple strategy for π-expansion of porphyrin is fusion of aromatic rings such as benzene ring at β-positions. Although tetrabenzoporphyrins (TBPs) are most extensively studied porphyrinoid fused with benzene rings, little is known about tetra[2,3]naphtho- and tetra[2,3]anthraporphyrins without meso-substituents compared to TBPs.2–5 Linearly ring-expansion results in the destabilization against oxidation and reduction as predicted by the molecular orbital calculation by Kobayashi et al.6 In spite of the instability, we have succeeded in the synthesis of tetra[2,3]anthraporphyrins by retro Diels-Alder strategy from the BCOD-fused precursors, which showed strong absorptions in the near-IR region.7 On the other hand, the porphyrins fused with polycyclic aromatic rings such as acenaphthylene or phenanthrene show weak Q bands at 600–900 nm.8–10 In 2006, we have reported the synthesis of fluoranthobenzoporphyrin 1 by the [3 + 1] porphyrin synthesis and the subsequent retro Diels-Alder reaction.11 The porphyrin 1 showed a strong absorption at 651 nm (log ε 5.45) for S0–S1 transition. Both Soret and Q bands are very strong absorptions with large molar absorption coefficient over 105 M-1cm-1. The fused aromatic ring in 1 consists of naphthalene and acenaphthylene. Thus, this strategy is expected to afford efficiently π-expanded porphyrins with intense absorptions in the near-IR region. We report herein the synthesis of tetra[8,9]fluoranthoTBPs 2 and 3, which are expected to be more stable linearly π-expanded porphyrins than tetra[2,3]anthraporphyrins, from the BCOD-fused precursors by the retro Diels-Alder reaction.
RESULTS AND DISCUSSION
Synthesis of BCOD-fused porphyrins 7 and 8 is summarized in Scheme 1. Diels-Alder reaction of 411,12 with 513 followed by oxidation with DDQ afforded fluorantho[8,9-f]isoindoles 6 in good yields. Porphyrins 7 were synthesized by reduction of 6 with LiAlH4 followed by tetramerization and oxidation with DDQ14 and purified by column chromatography on silica gel with CHCl3 to give two fractions of Rf 0.9 and 0.4 for 7a and Rf 0.5 and 0.2 for 7b, respectively. The relative ratio of Rf 0.9/0.4 (7a) is 69/31 and that of Rf 0.5/0.2 (7b) is 35/65. Free base porphyrins 7a and 7b were treated with Zn(OAc)2 to give their zinc complexes 8a and 8b in 77% and 96% yields, respectively.
1H NMR spectra of 7a and 7b in aromatic region are shown in Figure 1. Meso-CH signals of 7a (Rf 0.4) and 7b (0.2) were observed as a singlet peak, while those of 7a (0.9) and 7b (0.5) were observed as two intense and several small singlet peaks, and a number of singlet peaks, respectively. This indicates that 7a (0.4) and 7b (0.2) consist of a single isomer and have either cone- or 1,3-altenative-typed configuration.
The first fraction of 7a and 7b would be a mixture of other isomers. This selectivity would be derived from the bulky tert-butyl groups at phenyl groups. However, their configuration is not clear and under investigation.
Thermogravimetric analysis (TGA) curves of 7a and 8a are shown in Figure 2. The weight loss of 7a started at around 180 ºC and ceased after 280 ºC. The loss of weight was ca. 6%, consistent with the calculated value of 5.81%. Similarly, the retro Diels-Alder reactions of 7b, 8a, and 8b started at 150–200 ºC and were completed by 250–300 ºC. The loss of weight corresponded to the elimination of four ethylene molecules. The retro Diels-Alder reaction of 7 and 8 was carried out at 330 ºC for 1 h in vacuo in a glass tube oven to give tetra[8,9]fluoranthoTBPs 2 and 3 in nearly quantitative yields.
The absorption and emission data and the absolute quantum yields (Φ) of 2, 3, 7, and 8 are summarized in Table 1, and the typical spectra of 3a and 8a are shown in Figure 3. The absorption spectra were dramatically changed by retro Diels-Alder reaction of the BCOD-fused precursors as shown in Figure 3a. The Soret band of 3a showed a remarkable bathochromic shift from 402 nm for 8a (log ε 5.31) to 480 nm (5.42). The Q bands of 3a were also red shifted by 120–150 nm and very intense compared to that of 8a. The Soret and Q bands of fluoranthoTBPs 2 and 3 were observed at 480–500 and 670–760 nm with large molar absorption coefficient over 105 M-1 cm-1, respectively. The intensity of the Q bands was stronger than that of the Soret bands as shown in Table 1. Thus, 2 and 3 were expected to be suitable dyes for PDT. BCOD-porphyrins 7 and 8 showed the strong Soret band (log ε ca. 5.3) at 400–420 nm and the weak Q bands at 500–620 nm similar to those of typical porphyrins. The emission spectra with excitation at their Soret bands showed two bands at 620 and 700 nm for 7 and 580 and 630 nm for 8 with Φ values of 2–4%. On the other hand, fluoranthoTBPs 2 and 3 showed a strong emission at 750–770 nm and two weak bands at 790–850 nm. The intensities of them were stronger than those of BCOD-porphyrins 7 and 8. This observation was consistent with their absolute quantum yields. FluoranthoTBPs showed relatively high Φ values of ca. 35% for free base porphyrins 2 and 10% for the zinc complexes 3 compared to [2,3]naphthoporphyrins (13% in DMF) and meso-fluorene-substituted porphyrins (22% in toluene).15,16
In summary, tetra[8,9]fluoranthoTBPs have been synthesized from the BCOD-fused porphyrins by the retro Diels-Alder reaction. FluoranthoTBPs 2 and 3 showed red-shifted Soret band at ca. 500 nm and Q bands in near-IR region. Although porphyrins fused with polycyclic aromatic rings such as acenaphthylene or phenanthrene also show the Q bands in this region, their Q bands are weak similar to those of typical porphyrins.8–10 On the other hand, linearly π-expanded porphyrins, tetra[2,3]naphtho- or tetra[2,3]anthraporphyrins, are relatively unstable, while they show strong red-shifted Q bands. Thus, FluoranthoTBPs 2 and 3 are stable and exhibit strong absorptions over wide range of the visible-near-IR region, which are expected to show potential application for solar cells or other optics.
EXPERIMENTAL
General. Melting points were determined on a Yanaco micro melting point apparatus MP500D and are reported here uncorrected. DI-EI and FAB mass spectra were measured on a JEOL JMS-700. MALDI-TOF mass spectra were measured on an Applied Biosystems Voyager de Pro. TG analysis was performed on an SII Exstar 600 TG/DTA 6200. IR spectra were measured on a Horiba FT-720 infrared spectrophotometer, and UV-vis spectra on a JASCO V-570 spectrophotometer. The fluorescence emission spectra and the Φ values were measured on a Hamamatsu Photonics K.K. absolute PL quantum yield measurement system C9920-03. 1H NMR spectra (13C NMR spectra) were recorded on a JEOL AL-400 at 400 MHz (100 MHz). Elemental analyses were performed at the Integrated Center for Science, Ehime University.
[8,9]fluoranthoBCODpyrrole 6a
A solution of 4 (501 mg, 2.31 mmol) and 5a (714 mg, 2.00 mmol) in xylene (20 mL) was stirred at 135 °C for 3 days. After the solvent was removed under reduced pressure, a mixture of the residue and DDQ (454 mg, 2.00 mmol) in CHCl3 (70 mL) was stirred at rt for 21 h. The reaction mixture was poured into sat. aqueous NaHCO3. The organic layer was washed successively with sat. aqueous NaHCO3, water, and brine; dried over Na2SO4; and concentrated under reduced pressure. The residue was purified by column chromatography on silica gel with CHCl3 followed by recrystallization from CHCl3/hexane to give 6a (824 mg, 76%)
pale yellow powder; mp > 300 ºC; MS (70 eV) m/z (relative intensity) 544 (M++1, 9%), 515 (100), and 470 (69); IR (KBr disk) νmax 3305, 3053, 2935, 2863, and 1685 cm-1; 1H NMR (400 MHz, CDCl3) δ 8.43 (br, 1H), 7.36–7.46 (m, 12H), 7.23 (m, 2H), 6.62 (d, 2H, J = 6.6 Hz), 6.61 (s, 1H), 4.82 (m, 1H), 4.30 (m, 1H), 4.20 (q, 2H, J = 7.1 Hz), and 1.16 (t, 3H, J = 7.1 Hz); 13C NMR (100 MHz, CDCl3) δ 161.69, 142.87, 142.15, 139.23, 139.07, 136.72, 136.67, 135.15, 134.52, 134.48, 132.71, 130.61, 129.82, 129.52, 129.40, 129.34, 128.88, 128.84, 128.68, 128.63, 127.54, 127.49, 127.40, 127.36, 125.92, 125.89, 122.51, 122.44, 114.94, 113.64, 59.89, 34.68, 34.19, 27.63, 27.01, and 14.42. Anal. Calcd for C39H29NO2·1/2MeOH: C, 84.77; H, 5.58; N, 2.50. Found: C, 84.90; H, 5.35; N, 2.66.
[8,9]fluoranthoBCODpyrrole 6b
A solution of 4 (236 mg, 1.09 mmol) and 5b (473 mg, 1.01 mmol) in xylene (10 mL) was stirred at 135 °C for 3 days. After the solvent was removed under reduced pressure, a mixture of the residue and DDQ (269 mg, 1.18 mmol) in CHCl3 (70 mL) was stirred at rt for 1 h. The reaction mixture was poured into sat. aqueous NaHCO3. The organic layer was washed successively with sat. aqueous NaHCO3, water, and brine; dried over Na2SO4; and concentrated under reduced pressure. The residue was purified by column chromatography on silica gel with CHCl3 followed by recrystallization from CHCl3/hexane to give 6b (410 mg, 62%)
pale yellow powder; mp 290 ºC (decomp); MS (70 eV) m/z (relative intensity) 656 (M++1, 7%), 627 (12), and 154 (100); IR (KBr disk) νmax 3316, 3064, 2949, 2900, 2864, and 1674 cm-1; 1H NMR (400 MHz, CDCl3) δ 8.49 (br, 1H), 7.56–7.63 (m, 6H), 7.34–7.49 (m, 4H), 7.22 (t, 2H, J = 7.8 Hz), 6.63 (d, 2H, J = 2.7 Hz), 6.58 (dd, 2H, J = 5.4 and 6.9 Hz), 4.84 (m, 1H), 4.35 (m, 1H), 4.19 (m, 1H), 1.58–1.83 (m, 4H), 1.49 (s, 18H), and 1.15 (t, 3H, J = 7.1 Hz); 13C NMR (100 MHz, CDCl3) δ 161.83, 150.37, 150.16, 143.02, 142.20, 136.92, 136.87, 136.07, 135.24, 134.75, 134.66, 133.23, 132.74, 132.72, 130.75, 129.35, 129.30, 129.07, 128.99, 128.91, 127.37, 127.34, 125.77, 125.74, 125.70, 125.61, 125.52, 125.47, 122.45, 122.41, 114.92, 113.75, 34.84, 34.80, 34.75, 34.18, 31.64, 27.68, and 27.14. Anal. Calcd for C47H45NO2: C, 86.07; H, 6.92; N, 2.14. Found: C, 86.13; H, 6.97; N, 2.11.
Porphyrin 7a
To a solution of 6a (326 mg, 0.600 mmol) in dry THF (20 mL) was added slowly LiAlH4 (113 mg, 2.98 mmol) at 0 °C under an Ar atmosphere in a shaded vessel. The resulting mixture was stirred below 0 ºC for 4 h. After slow addition of water, the mixture was diluted with CHCl3 and filtrated with Celite. The organic layer was separated, washed successively with water and brine, dried over Na2SO4, and concentrated under reduced pressure. The residue was diluted with degassed CHCl3 (150 mL) and p-TsOH·H2O (11 mg) was added in a shaded vessel. After stirring at rt for 16 h, DDQ (90 mg, 0.40 mmol) was added. The resulting mixture was stirred at rt for 40 min. The mixture was washed successively with sat. aqueous NaHCO3, water, and brine; dried over Na2SO4; and concentrated under reduced pressure. The residue was purified by column chromatography on alumina with CHCl3, column chromatography on silica gel with CHCl3, and recrystallization from CHCl3/MeOH to give 7a (Rf 0.9) (125 mg, 43%) and 7a (Rf 0.4) (54 mg, 19%).
7a (Rf 0.9): brown powder; mp 220 ºC (decomp); MS (FAB) m/z 1929 (M++2) and 1816; UV-vis (CHCl3) λmax, nm (log ε) 315 (4.49), 330 (4.60), 400 (5.31), 499 (4.27), 531 (3.99), 566 (3.89), and 619 (3.24);
1H NMR (400 MHz, CDCl3) δ 9.95–10.17 (m, 4H), 7.20–8.07 (m, 56H), 6.47–7.00 (m, 8H), 5.94–6.12 (m, 8H), 2.04–2.63 (m, 16H), and -4.61–-4.44 (br, 2H). Anal. Calcd for C148H94N4·H2O: C, 91.33; H, 4.97; N, 2.88. Found: C, 91.36; H, 4.85; N, 2.87.
7a (Rf 0.4): brown powder; mp 220 ºC (decomp); MS (FAB) m/z 1929 (M++2) and 1816; UV-vis (CHCl3) λmax, nm (log ε) 315 (4.53), 330 (4.64), 402 (5.31), 502 (4.28), 534 (4.01), 569 (3.90), and 621 (3.24); 1H NMR (400 MHz, CDCl3) δ 10.04 (s, 4H), 7.91 (m, 24H), 7.84 (d, 8H, J = 7.6 Hz), 7.75 (m, 8H), 7.66 (d, 8H, J = 8.2 Hz), 7.31 (dd, 8H, J = 7.1 and 8.2 Hz), 6.66 (d, 8H, J = 7.1 Hz), 6.02 (m, 8H), 2.20 (m, 8H), 1.69 (m, 8H), and -4.27 (br, 2H). Anal. Calcd for C148H94N4·H2O: C, 91.33; H, 4.97; N, 2.88. Found: C, 91.09; H, 4.96; N, 2.82.
Porphyrin 7b
To a solution of 6b (262 mg, 0.400 mmol) in dry THF (15 mL) was added slowly LiAlH4 (75 mg, 1.98 mmol) at 0 °C under an Ar atmosphere in a shaded vessel. The resulting mixture was stirred below 0 ºC for 4.5 h. After slow addition of water, the mixture was diluted with CHCl3 and filtrated with Celite. The organic layer was separated, washed successively with water and brine, dried over Na2SO4, and concentrated under reduced pressure. The residue was diluted with degassed CHCl3 (150 mL) and p-TsOH·H2O (10 mg) was added in a shaded vessel. After stirring at rt for 16 h, DDQ (64 mg, 0.28 mmol) was added. The resulting mixture was stirred at rt for 30 min. The mixture was washed successively with sat. aqueous NaHCO3, water, and brine; dried over Na2SO4; and concentrated under reduced pressure. The residue was purified by column chromatography on alumina with CHCl3, column chromatography on silica gel with CHCl3, and recrystallization from CHCl3/MeOH to give 7b (Rf 0.5) (54 mg, 23%) and 7b (Rf 0.2) (97 mg, 41%).
7b (Rf 0.5): brown powder; mp 220 ºC (decomp); MS (MALDI-TOF) m/z 2376 (M++1), 2349, and 2323; UV-vis (CHCl3) λmax, nm (log ε) 296 (5.07), 315 (4.54), 330 (4.66), 403 (5.31), 501 (4.29), 535 (4.01), 569 (3.93), and 622 (3.35); 1H NMR (400 MHz, CDCl3) δ 9.92–10.21 (m, 4H), 6.97–7.97 (m, 48H), 6.08–6.96 (m, 8H), 5.83–6.08 (m, 8H), 1.73–2.79 (m, 16H), 1.33–1.69 (m, 72H), and -4.61–-4.02 (m, 2H). Anal. Calcd for C180H158N4·H2O: C, 90.26; H, 6.73; N, 2.34. Found: C, 90.40; H, 6.69; N, 2.29.
7b (Rf 0.2): brown powder; mp 210 ºC (decomp); MS (FAB) m/z 2376 (M++2), 2349, and 2321; UV-vis (CHCl3) λmax, nm (log ε) 296 (5.04), 315 (4.52), 330 (4.64), 407 (5.25), 506 (4.27), 537 (3.93), 576 (3.89), and 623 (3.28); 1H NMR (400 MHz, CDCl3) δ 10.01 (s, 4H), 7.82 (m, 24H), 7.57 (d, 8H, J = 8.1 Hz), 7.49 (m, 8H), 7.37 (d, 8H, J = 7.1 Hz), 7.18 (t, 8H, J = 7.3 Hz), 6.47 (m, 8H), 5.91 (s, 8H), 2.27 (m, 8H), 1.95 (m, 8H), 1.49 (s, 72H), and -4.07 (br, 2H); 13C NMR (100 MHz, CDCl3) δ 150.60, 143.47, 136.73, 136.63, 135.04, 133.40, 132.78, 129.54, 129.38, 129.24, 127.27, 126.68, 126.21, 125.84, 122.68, 96.25, 37.37, 35.02, 31.78, and 27.97. Anal. Calcd for C180H158N4: C, 90.94; H, 6.70; N, 2.36. Found: C, 90.71; H, 6.79; N, 2.31.
(Porphyrinato)zinc(II) 8a
A saturated solution of Zn(OAc)2·2H2O in MeOH (2 mL) was added to a solution of 7a (Rf 0.4) (11 mg, 0.0065 mmol) in CHCl3 (50 mL) at rt. The resulting mixture was stirred at same temperature for 1 h. The reaction mixture was poured into water. The organic layer was washed successively with water and brine, dried over Na2SO4, and concentrated under reduced pressure. The residue was purified by column chromatography on silica gel with CHCl3 followed by recrystallization from CHCl3/MeOH to give 8a (9 mg, 77%).
reddish purple powder; mp 210 ºC (decomp); MS (FAB) m/z 1991 (M++2); UV-vis (CHCl3) λmax, nm (log ε) 315 (4.53), 330 (4.64), 402 (5.31), 534 (4.01), and 569 (3.90); 1H NMR (400 MHz, CDCl3) δ 10.15 (s, 4H), 7.85–8.02 (m, 32H), 7.76 (m, 8H), 7.67 (d, 8H, J = 8.0 Hz), 7.32 (dd, 8H, J = 7.1 and 8.0 Hz), 6.66 (d, 8H, J = 7.1 Hz), 6.11 (s, 8H), 2.24 (m, 8H), and 1.75 (m, 8H). Anal. Calcd for C148H92N4Zn·2MeOH: C, 87.63; H, 4.90; N, 2.73. Found: C, 87.93; H, 5.19; N, 2.98.
(Porphyrinato)zinc(II) 8b
A saturated solution of Zn(OAc)2·2H2O in MeOH (1 mL) was added to a solution of 7b (Rf 0.2) (30 mg, 0.013 mmol) in CHCl3 (30 mL) at rt. The resulting mixture was stirred at same temperature for 1 h. The reaction mixture was poured into water. The organic layer was washed successively with water and brine, dried over Na2SO4, and concentrated under reduced pressure. The residue was purified by column chromatography on silica gel with CHCl3 followed by recrystallization from CHCl3/MeOH to give 8b (30 mg, 96%).
red powder; mp 220 ºC (decomp); MS (MALDI-TOF) m/z 2439 (M++2), 2412, 2385, 2358 and 2329; UV-vis (CHCl3) λmax, nm (log ε) 296 (5.06), 315 (4.55), 330 (4.65), 373 (4.80), 417 (5.40), 540 (4.28), and 571 (4.18); 1H NMR (400 MHz, CDCl3) δ 10.17 (s, 4H), 7.86 (s, 16H), 7.59 (d, 8H, J = 8.1 Hz), 7.54 (d, 8H, J = 7.6 Hz), 7.34 (d, 8H, J = 7.9 Hz), 7.21 (m, 8H), 6.53 (m, 8H), 5.99 (s, 8H), 2.36 (m, 8H), 2.06 (m, 8H), and 1.49 (s, 72H); 13C NMR (100 MHz, CDCl3) δ 150.49, 149.76, 143.85, 142.43, 136.72, 136.69, 134.85, 133.31, 132.73, 129.49, 129.34, 129.16, 127.21, 126.65, 126.17, 125.71, 122.64, 97.28, 37.58, 34.92, 31.71, and 28.13. Anal. Calcd for C180H56N4Zn·1/2CHCl3: C, 86.71; H, 6.31; N, 2.24. Found: C, 86.53; H, 6.46; N, 2.47.
Retro Diels-Alder reaction
Porphyrins 7, and 8 (ca. 10 mg each) were heated at 330 ºC under reduced pressure for 1 h in a glass tube to give fluoranthoTBPs 2 and 3 in nearly quantitative yields.
2a: brown powder; mp > 300 ºC; MS (FAB) m/z 1816 (M++1); UV-vis (CHCl3) λmax, nm (log ε) 314 (5.11), 348 (5.01), 397 (4.71), 494 (5.44), 679 (4.64), 712 (4.60), and 751 (5.63); Anal. Calcd for C140H78N4: C, 92.59; H, 4.33; N, 3.08. Found: C, 92.42; H, 4.63; N, 3.09.
2b: brown powder; mp > 300 ºC; MS (MALDI-TOF) m/z 2264 (M++1); UV-vis (CHCl3) λmax, nm (log ε) 315 (5.14), 348 (5.03), 398 (4.72), 499 (5.39), 687 (4.64), 722 (4.65), and 760 (5.57); 1H NMR (400 MHz, CDCl3) δ 10.20 (s, 4H), 9.54 (s, 8H), 9.72 (d, 16H, J = 8.1 Hz), 7.71–7.79 (m, 24H), 7.39 (dd, 8H, J = 7.1 and 8.1 Hz), 6.52 (d, 8H, J = 7.1 Hz), 1.76 (s, 72H), and -0.88 (br, 2H). Anal. Calcd for C172H142N4·H2O: C, 90.49; H, 6.36; N, 2.45. Found: C, 90.46; H, 6.41; N, 2.43.
3a: dark brown powder; mp > 300 ºC; MS (FAB) m/z 1879 (M++2); UV-vis (CHCl3) λmax, nm (log ε) 322 (5.14), 480 (5.42), 672 (4.59), 703 (4.61), and 739 (5.71). Anal. Calcd for C140H76N4Zn·H2O: C, 88.61; H, 4.14; N, 2.95. Found: C, 88.88; H, 4.45; N, 2.99.
3b: dark brown powder; mp > 300 ºC; MS (MALDI-TOF) m/z 2327 (M++2); UV-vis (CHCl3) λmax, nm (log ε) 323 (5.24), 484 (5.44), 677 (4.65), 710 (4.66), and 747 (5.68); 1H NMR (400 MHz, CDCl3) δ 10.20 (s, 4H), 9.54 (s, 8H), 9.72 (d, 16H, J = 8.1 Hz), 7.79–7.71 (m, 24H), 7.39 (dd, 8H, J = 7.1 and 8.1 Hz), 6.52 (d, 8H, J = 7.1 Hz), 1.76 (s, 72H) and -0.88 (brs, 2H). Anal. Calcd for C172H140N4Zn·H2O: C, 88.04; H, 6.10; N, 2.39. Found: C, 87.83; H, 6.07; N, 2.39.
ACKNOWLEDGEMENTS
The authors thank Venture Business Laboratory, Ehime University, for assistance in obtaining the MALDI-TOF mass spectra. We used ethyl isocyanoacetate for the preparation of the pyrroles by Barton-Zard reaction from the Nippon Synthetic Chem. Ind. (Osaka Japan).
References
1. T. D. Lash, ‘The Porphyrin Handbook’ Vol.2., ed. by K. M. Kadish, K. M. Smith, and R. Guilard, Academic Press, Inc., San Diego, 2000, pp. 125-199.
2. M. Rein and M. Hanack, Chem. Ber., 1988, 121, 1601. CrossRef
3. S. Ito, N. Ochi, H. Uno, T. Murashima, and N. Ono, Chem. Commun., 2000, 893. CrossRef
4. V. N. Kopranenkov, A. M. Vorotnikov, and E. A. Luk’yanets, J. Gen. Chem. USSR, 1979, 49, 2467.
5. O. S. Finikova, A. V. Cheprakov, and S. A. Vinogradov, J. Org. Chem., 2005, 70, 9562. CrossRef
6. (a) N. Kobayashi and H. Konami, J. Porphyrins Phthalocyanines, 2001, 5, 233; CrossRef (b) J. Mack, Y. Asano, N. Kobayashi, and M. J. Stillman. J. Am. Chem. Soc., 2005, 127, 17697. CrossRef
7. H. Yamada, D. Kuzuhara, T. Takahashi, Y. Shimizu, K. Uota, T. Okujima, H. Uno, and N. Ono, Org. Lett., 2008, 10, 2947. CrossRef
8. (a) N. Ono, K. Hironaga, S. Kaneko, T. Murashima, T. Ueda, C. Tsukamura, and T. Ogawa, J. Chem. Soc., Perkin Trans. 1, 1996, 417; CrossRef (b) T. Murashima, K. Fujita, K. Ono, T. Ogawa, H. Uno, and N. Ono, J. Chem. Soc., Perkin Trans. 1, 1996, 1403. CrossRef
9. (a) T. D. Lash, J. Porphyrins Phthalocyanines, 2001, 5, 267; CrossRef (b) J. D. Spence and T. D. Lash, J. Org. Chem., 2000, 65, 1530; CrossRef (c) T. D. Lash, Y. Lin, B. H. Novak, and M. D. Parikh, Tetrahedron, 2005, 61, 11601. CrossRef
10. H.-J. Xu, Z. Shen, T. Okujima, N. Ono, and X.-Z. You, Tetrahedron Lett., 2006, 47, 931. CrossRef
11. T. Okujima, N. Komobuchi, H. Uno, and N. Ono, Heterocycles, 2006, 67, 255. CrossRef
12. (a) S. Ito, T. Murashima, H. Uno, and N. Ono, Chem. Commun., 1998, 1661; CrossRef (b) S. Ito, N. Ochi, T. Murashima, H. Uno, and N. Ono, Heterocycles, 2000, 52, 399; CrossRef (c) T. Okujima, G. Jin, Y. Hashimoto, H. Yamada, H. Uno, and N. Ono, Heterocycles, 2006, 70, 619. CrossRef
13. M. Wehmeier, M. Wagner, and K. Müllen, Chem. Eur. J., 2001, 7, 2197. CrossRef
14. N. Ono and K. Maruyama, Chem. Lett., 1988, 1511. CrossRef
15. O. S. Finikova, S. E. Aleshchenkov, R. P. Briñas, A. V. Cheprakov, P. J. Carroll, and S. A. Vinogradov, J. Org. Chem., 2005, 70, 4617. CrossRef
16. B. Li, J. Li, Y. Fu, and Z. Bo, J. Am. Chem. Soc., 2004, 126, 3430. CrossRef