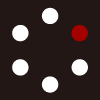
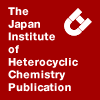
HETEROCYCLES
An International Journal for Reviews and Communications in Heterocyclic ChemistryWeb Edition ISSN: 1881-0942
Published online by The Japan Institute of Heterocyclic Chemistry
e-Journal
Full Text HTML
Received, 11th August, 2009, Accepted, 18th September, 2009, Published online, 18th September, 2009.
DOI: 10.3987/COM-09-S(S)108
■ Quinone Dimers Connected by 1,4-Phenylene and 2,5-Thienylene Moieties as a π Linker
Naoto Hayashi,* Teru Sakakibara, Takahiro Ohnuma, Junro Yoshino, and Hiroyuki Higuchi
Department of Chemistry, Graduate School of Science and Engineering, Toyama University, Gofuku 3190, Toyama 930-8555, Japan
Abstract
The synthesis and characterization of quinone dimers connected by 1,4-phenylene (QPQ) and 2,5-thienylene (QTQ) linkers are described. QPQ and QTQ were synthesized by means of Suzuki coupling and subsequent oxidation. Significant bathochromic shifts were observed in the electronic absorption spectra of QPQ and QTQ as compared to that of the quinine dimers without a linker. In addition, cyclic voltammetry and DFT calculations demonstrated that QTQ was a stronger electron acceptor than QPQ due to its planar structure, even though QTQ contains an electron-rich thiophene ring.INTRODUCTION
π-linkers are important tools to control the structure and properties of π-conjugated compounds.1 In general, π-linkers are required to be stable and structurally rigid, in order for their p orbitals to overlap efficiently with the p orbitals of the π-moieties situated at both ends. In this regard, acetylene, diacetylene, arylene, and oligo(arylene) groups have been frequently employed as π-linkers.2-9
Recently, we reported the synthesis and properties of quinone dimers bearing acetylene (QAQ) and diacetylene (QAAQ) moieties as a π-linker.10 As compared to the quinine dimers without a linker (QQ),11 QAQ and QAAQ showed significant bathochromic shifts and increases in their first reduction potentials. We proposed that this was due to the essentially planar π-conjugated systems of QAQ and QAAQ based on their X-ray diffraction analysis and molecular-orbital calculations. In contrast, the X-ray structure of QQ showed that the quinone moieties were tilted by 38°.
In the present study, we report the synthesis and characterization of quinone dimers linked by arylene moieties, QPQ and QTQ. As the π-linker, we selected 1,4-phenylene and 2,5-thienylene, since these functional groups are widely employed in oligomer and material chemistry. The 1,4-phenylene moiety is the simplest in structure and can be synthesized in a facile manner. However, the p orbital overlap with the adjacent π-moieties is generally inefficient because of a large dihedral angle between 1,4-phenylene and the adjacent π-moiety due to the steric repulsion between the ortho-hydrogen atoms. In contrast, π-systems constructed with a 2,5-thienylene linker usually adopt a planar conformation in order to give
fully conjugated π-system. Nevertheless, the 2,5-thienylene linker appeared to be disadvantageous in the present study because electron-rich character of thiophene could potentially impair the electron acceptor character of the quinone moiety. The target compounds were synthesized by means of Suzuki coupling of the quinone precursor and π-linker,12 which has been quite widely employed in the synthesis of biaryls.13 tert-Butyl groups were incorporated to increase the solubility and stability of the compounds. Properties of the π-conjugated system of QPQ and QTQ were investigated by electronic absorption spectroscopy, cyclic voltammetry, and DFT calculations. The experimental and theoretical results were then compared with those of QQ.
RESULTS AND DISCUSSION
The synthesis of QPQ is shown in Figure 1. 4-tert-Butyl-2,5-dimethoxyphenylboronic acid (1) and 1,4-dibromobenzene (2) were subjected to Suzuki coupling to give compound 3. Deprotection by BBr3, followed by oxidation using cerium(IV) ammonium nitrate (CAN) afforded QPQ. Synthesis of QTQ was initially attempted in a similar manner, but with unsatisfactory results. Suzuki coupling of 1 and 2,5-dibromothiophene (5) gave thiophene 6. However, the reaction of 6 with BBr3 did not afford hydroquinone 7. This was likely due to the formation of charge-transfer complex of 6 and BBr3. Demethylation of 6 using either EtSNa or Me3SiI was also unsuccessful. The direct oxidation of thiophene 6 with CAN also gave a complex mixture, probably because the thiophene moiety was oxidized by CAN.
Hydroquinone 7 was successfully prepared by an alternative route. As shown in Figure 2, 2-tert-butyl-5-iodo-1,4-dimethoxybenzene was oxidized with CAN to give quinone 8, which was then reduced by Na2S2O4 to yield hydroquinone 9. The hydroxy groups of 9 were protected with methoxymethyl (MOM) groups to provide iodide 10, which was converted to arylboronic acid 11 by conventional methods.14 Boronic acid 11 and 5 were subjected to Suzuki coupling to give 12, which was then deprotected in the presence of acid, and oxidized by CAN to yield QTQ. Both QPQ and QTQ were stable in air, and highly soluble in halogenated solvents, such as chloroform and dichloromethane, and moderately soluble in ethereal solvents.
Electronic absorption spectra of QPQ, QTQ, and QQ are shown in Figure 3. The bathochromic shift of the longest wavelength absorption band (λmax) was observed in QPQ compared to QQ. This is most likely to be attributed to the HOMO of QPQ, which is supposed to be located mainly on the phenylene linker, being higher in energy. The bathochromic shift of λmax of QTQ could be explained in a similar manner. The larger bathochromic shift in QTQ should be due to either the electron-rich character of 2,5-thienylene linker or the highly planar structure of the π-conjugated system, or both.
In order to elucidate the origin of the bathochromic shifts in QPQ and QTQ, DFT calculations (B3LYP/6-31G** methods) were performed for model compounds, QQ', QPQ', and QTQ', which have
no tert-butyl substituents for simplicity. For each compound, geometrical optimizations of a series of conformers shown in Figure 4 were conducted. In the global minimum energy structures for QQ' and QPQ' are the a-conformer and s-conformer, respectively. When the energy levels of s-QPQ' are compared to those of a-QQ', the rise of HOMO (0.52 eV) is more significant than that of LUMO (0.21 eV). This observation, compounded by the fact that HOMO is mainly located on the phenylene moiety (Figure 5), supports our hypothesis that the HOMO located on the linker should be responsible for the bathochromic shift of λmax of QPQ compared to QQ. In the case of QTQ', the sa-conformer is the global minimum energy structure, followed by the aa-conformer, which virtually possesses the same energy. However, the ss-conformer is unstable, thus a minimum energy structure is unobtainable. The π-conjugated systems of the sa- and aa-conformers are highly planar and the HOMO levels are raised
significantly (Table 1). Evidently, these are characteristics of the 2,5-thienylene linker, which should be responsible for the significant bathochromic shift in QTQ.
It is noteworthy that, even though the thienylene group is inherently electron-donating in nature, the LUMO level of QTQ' is considerably lower than that of s-QPQ' and comparable to that of a-QQ'. A similar tendency was observed in the reduction potentials of QQ,10 QPQ, and QTQ as measured by cyclic voltammetry (Table 1). Both QPQ and QTQ showed the reversible first (E1) and second (E2) waves, which were both attributed to one-electron reductions, followed by the irreversible third (E3) wave. As expected from the theoretical calculations, E1 of QTQ was positively shifted by 0.09 V as compared to that of QPQ. The higher electron-acceptor character should be due to the extended LUMO of QTQ. In fact, as shown in Figure 5, the LUMO of QTQ' is efficiently delocalized between the quinone and thienylene moieties, while the LUMO of QPQ' is localized on the quinone groups. The difference between E1 and E2 (= ΔE) is larger in QTQ (0.17 V) than in QPQ (0.08 V). This is also indicative of strong interaction between the two quinone moieties and the presence of efficiently delocalized LUMO in QTQ with the aid of 2,5-thienylene linker. In spite of a large dihedral angle (37°), a significantly higher ΔE was found in QQ (0.34 V), probably because of the large inductive (field) effects of the quinone moieties.11
In conclusion, two quinone dimers bearing arylene π linkers, QPQ and QTQ, have been synthesized, and their π-conjugated systems were characterized. In QPQ, a significant bathochromic shift of λmax was observed as compared to QQ, which was mainly attributed to the rise of the HOMO level. The LUMO level of QPQ was also higher than that of QQ, which led to the negative shift of E1. In QTQ, greater bathochromic shift of λmax was observed, which was also due to the rise of the HOMO level. Despite the electron-rich character of the 2,5-thienylene moiety, E1 of QTQ was positively shifted as compared to that of QQ. DFT calculations revealed that this should arise from the planar structure of QTQ, which results in a fully conjugated π-system. These studies suggest that even an electron-rich arylene moiety could serve as a viable linker to construct an efficiently conjugated π system without impairing the electron acceptor character, and therefore could provide insight to the design of higher quinine oligomers.
EXPERIMENTAL
General. All commercially available chemicals were used without further purification except Et2O and THF, which were dried over sodium and distilled from sodium containing benzophenone to form the ketyl before use, respectively. Melting points were determined on microscopic thermometer without correction. 1H and 13C NMR spectra were recorded on a JEOL JNM-ECP600 (600 MHz for 1H) or a JEOL ECX-300/TRH (300 MHz for 1H and 75 MHz for 13C) with tetramethylsilane as internal reference. Mass spectra were conducted on a JEOL MStation JMS-700 (EI and HRMS/EI). Infrared spectra were measured on a JASCO FT/IR-6100. Cyclic voltammetry (CV) was performed on an ALS model 600A in 1.0 mM of substrate. All CV measurements were carried out in anhydrous CH2Cl2 containing 0.1 M tetrabutylammonium perchlorate (nBu4NClO4) as a supporting electrolyte, purging with argon prior to conduct the experiment. Platinum electrode was used as a working electrode and a platinum wire as a counter electrode. All potentials were recorded versus Ag/Ag+ electrode (in MeCN) as a reference electrode.
Synthesis of 4,4''-di-tert-butyl-2,2'',5,5''-tetramethoxyl-1,1':4',1''-terphenyl (3). A bi-layer solution of 4-tert-butyl-2,5-dimethoxyphenylboronic acid (1) (1.29 g, 5.4 mmol) and 1,4-dibromobenzene (2) (0.51 g, 2.2 mmol) in toluene (30 mL), EtOH (10 mL) and 1 mol L–1 aqueous sodium carbonate (20 mL) was degassed with argon. After tetrakis(triphenylphosphine)palladium (0.25 g, 0.22 mmol) was added, the reaction mixture was refluxed overnight. After cooling, organic layer was separated, and aqueous layer was extracted with Et2O. Combined organic phase was washed with brine, dried over Na2SO4, and evaporated to dryness. From the crude product, 3 (0.77 g, 71%) was isolated by preparative chromatography (SiO2, n-hexane/EtOAc 9:1) as a white powder.
Mp 218-220 °C. 1H NMR (600 MHz, CDCl3): δ = 7.59 (s, 4H, Ar), 6.97 (s, 2H, Ar), 6.93 (s, 2H, Ar), 3.84 (s, 6H, OCH3), 3.79 (s, 6H, OCH3), 1.43 (s, 18H, tert-Bu). 13C NMR (75 MHz, CDCl3): δ = 152.77, 150.04, 138.36, 136.91, 129.02, 128.36, 114.71, 111.72, 56.61, 55.80, 35.01, 29.74. MS: m/z = 462 (M+). HRMS (m/z): 462.2766 (M+, calcd. 462.2771 for C30H38O4).
Synthesis of 4,4''-di-tert-butyl-1,1':4',1''-terphenyl-2,2',5,5'-tetraol (4). To a solution of 3 (0.40 g, 0.86 mmol) in CHCl3 (10 mL) was added BBr3 (0.32 mL, 3.5 mmol) at 0 °C dropwisely under argon. After stirring 4 h, MeOH and water were added in this order. After removing methanol in vacuum, the organic layer was separated and the aqueous layer was extracted with Et2O. Combined organic phase was washed with brine, dried over Na2SO4, and evaporated to dryness to leave 4 quantitatively as a white powder. The crude 4 was used in the following reaction without further purification.
Synthesis of 1,4-bis(5-tert-butyl-1,4-benzoquinon-2-yl)benzene (QPQ). To a solution of 4 (0.43 g, 1.1 mmol) in MeCN (40 mL) was added cerium ammonium nitrite (CAN, 2.36 g, 4.3 mmol) at ambient temperature in the dark. After stirring for 4 h, the reaction mixture was quenched with water, then subjected to supersonic treatment until the solid was precipitated. The precipitate was separated by suction filtration, washed with acetone/water (1:1 v/v), and dried to give analytically pure QPQ as a yellow powder (0.35 g, 85%).
Mp 291-294 °C. 1H NMR (600 MHz, CDCl3): δ = 7.57 (s, 4H, Ar), 6.81 (s, 2H, CH), 6.70 (s, 2H, CH), 1.33 (s, 18H, tert-Bu). 13C NMR (75 MHz, CDCl3): δ = 187.59, 187.40, 156.02, 143.42, 134.99, 133.82, 132.07, 129.29, 35.15, 29.14. MS: m/z = 402 (M+). HRMS (m/z): 402.1832 (M+, calcd. 402.1832 for C26H26O4).
Synthesis of 2,5-bis(4-tert-butyl-2,5-dimethoxyphenyl)thiophene (6). A bi-layer solution of 1 (2.60 g, 10 mmol) and 2,5-dibromothiophene (5) (0.54 g, 4.9 mmol) in toluene (30 mL), EtOH (10 mL) and 1 mol L–1 aqueous sodium carbonate (20 mL) was degassed with argon. After tetrakis(triphenylphosphine)palladium (0.58 g, 0.50 mmol) was added, the reaction mixture was refluxed overnight. After cooling, organic layer was separated, and aqueous layer was extracted with Et2O. Combined organic phase was washed with brine, dried over Na2SO4, and evaporated to dryness. From the crude product, 6 (1.60 g, 70%) was isolated by preparative chromatography (SiO2, n-hexane/EtOAc 9:1) as a pale yellow solid.
Mp 155-157 °C. 1H NMR (600 MHz, CDCl3): δ = 7.43 (s, 2H, Ar), 7.15 (s, 2H, Ar), 6.96 (s, 2H, Ar), 3.89 (s, 6H, OCH3), 3.88 (s, 6H, OCH3), 1.40 (s, 18H, tert-Bu). 13C NMR (75 MHz, CDCl3): δ = 152.88, 149.50, 139.00, 138.57, 125.28, 121.72, 112.36, 111.87, 56.77, 55.79, 35.02, 29.69. MS: m/z = 468 (M+). HRMS (m/z): 468.2333 (M+, calcd. 468.2334 for C28H36O4S).
Synthesis of 2-tert-butyl-5-iodo-1,4-benzoquinone (8). To a solution of 2-tert-butyl-5-iodo-1,4-dimethoxybenzene (4.39 g, 13.7 mmol) in MeCN (80 mL) was added CAN (22.9 g, 41.8 mmol) dissolved in a minimum amount of water. After stirring for 3.5 h in the dark, the reaction mixture was diluted by water, and then exposed to supersonic waves. The precipitation was collected by suction filtration, washed by water, and dried under vacuum to give analytically pure 8 as an orange powder (3.88g, 98%).
Mp 83-84 °C. 1H NMR (300 MHz, CDCl3): δ = 7.55 (s, 1H, CH), 6.82 (s, 1H, CH), 1.28 (s, 9H, tert-Bu). 13C NMR (75 MHz, CDCl3): δ = 184.18, 181.34, 156.62, 147.92, 129.78, 117.48, 35.45, 29.02. MS: m/z = 290 (M+). HRMS (m/z): 289.9818 (M+, calcd. 289.9804 for C10H11O2I). IR (Nujol. cm–1): 1649 (νC=O).
Synthesis of 2-tert-butyl-5-iodobenzen-1,4-diol (9). A bi-layer solution of 8 (7.54 g, 26.0 mmol), Na2S2O4 (22.6 g, 130 mmol) in water (100 mL) and CHCl3 (80 mL) was stirred for 2 h in the dark. The reaction mixture was acidified with dilute HCl, extracted with CHCl3, dried over MgSO4, and concentrated under vacuum to give analytically pure 9 as a brownish yellow solid (7.41g, 98%).
Mp 115-116 °C. 1H NMR (300 MHz, CDCl3): δ = 6.97 (s, 1H, CH), 6.92 (s, 1H, CH), 4.89 (s, 1H, OH), 4.86 (s, 1H, OH), 1.36 (s, 9H, tert-Bu). 13C NMR (75 MHz, CDCl3): δ = 148.74, 148.69, 139.17, 124.80, 113.58, 80.42, 34.59, 29.23. MS: m/z = 292 (M+). HRMS (m/z): 291.9968 (M+, calcd. 291.9960 for C10H13O2I). IR (Nujol. cm–1): 3494, 3408 (νO–H).
Synthesis of 1-tert-butyl-4-iodo-2,5-bis(methoxymethoxy)benzene (10). NaH (60% in mineral oil, 1.64g, 0.99 g as NaH, 41.0 mmol) was added to THF (60 mL) under argon. The mixture was cooled to 0 °C and a solution of 9 (2.00 g, 6.85 mmol) in THF (30 mL) was added portionwisely. After stirring for 30 min at 0 °C, chloromethyl methyl ether was added, and the mixture was stirred for additional 16 h at ambient temperature. After excess amount of aqueous 10% NaOH was added, the mixture was extracted with EtOAc, dried over MgSO4, and concentrated under vacuum. From the crude product, 10 (2.56 g, 88%) was isolated by preparative chromatography (SiO2, n-hexane/EtOAc 9:1) as pale yellow oil.
1H NMR (300 MHz, CDCl3): δ = 7.49 (s, 1H, Ar), 7.04 (s, 1H, Ar), 5.15 (s, 2H, CH2OCH3), 5.13 (s, 2H, CH2OCH3), 3.53 (s, 3H, CH2OCH3), 3.47 (s, 3H, CH2OCH3), 1.36 (s, 9H, tert-Bu). 13C NMR (75 MHz, CDCl3): δ = 151.81, 150.88, 139.90, 124.55, 114.77, 96.00, 94.45, 83.91, 56.25, 55.85, 34.90, 29.39. MS: m/z = 380 (M+). HRMS (m/z): 380.0495 (M+, calcd. 380.0485 for C14H21O4I).
Synthesis of 4-tert-butyl-2,5-bis(methoxylmethoxy)phenylboronic acid (11). To a solution of 10 (6.43 g, 16.9 mmol) in Et2O (100 mL) was dropwised n-butyllithium (1.6 M in n-hexane, 17.0 mL, 27.2 mmol) at –78 °C under argon. After stirring for 1.5 h at –78 °C, trimethyl borate (5.70 mL, 51.3 mmol) was added slowly. The resulting mixture was stirred for additional 1 h, while the temperature was held at –78 °C, then the reaction mixture was allowed to warm to ambient temperature. After cooling to 0 °C, small amount of water was added to quench the reaction, then 25% acetic acid was added to keep pH of the solution to be 6.5.14 The reaction mixture was extracted with Et2O. Combined organic extracts were washed with brine, dried over MgSO4, and evaporated to dryness. The crude product was recrystallized from n-hexane to give 11 (2.45 g; 49%) as a white solid.
Mp 68-69 °C. 1H NMR (300 MHz, CDCl3): δ = 7.50 (s, 1H, Ar), 7.11 (s, 1H, Ar), 5.24 (s, 2H, CH2OCH3), 5.23 (s, 2H, CH2OCH3), 3.51 (s, 6H, CH2OCH3), 1.40 (s, 9H, tert-Bu). 13C NMR (75 MHz, CDCl3): δ = 157.07, 151.18, 143.48, 120.98, 113.11, 95.44, 94.33, 56.43, 56.13, 35.32, 29.55. MS: m/z = 298 (M+). HRMS (m/z): 298.1579 (M+, calcd. 298.1588 for C14H23O6B).
Synthesis of 2,5-bis{4-tert-butyl-2,5-bis(methoxymethoxy)phenyl}thiophene (12). A b-layer solution of 11 (2.57 g, 8.62 mmol) and 5 (0.846 g, 3.50 mmol) in toluene (30 mL), EtOH (10 mL) and 1 mol L–1 aqueous sodium carbonate (20 mL) was degassed with argon. After tetrakis(triphenylphosphine)palladium (0.402 g, 0.348 mmol) was added, the reaction mixture was refluxed for 18 h. After cooling, organic layer was separated, and aqueous layer was extracted with Et2O. Combined organic phase was washed with brine, dried over MgSO4, and evaporated to dryness. From the crude product, 12 (1.42 g, 69%) was isolated by recrystallization from Et2O and n-hexane as a yellow solid.
Mp 121-123 °C. 1H NMR (600 MHz, CDCl3): δ = 7.40 (s, 2H, Ar), 7.39 (s, 2H, Ar), 7.14 (s, 2H, Ar), 5.24 (s, 4H, CH2OCH3), 5.17 (s, 4H, CH2OCH3), 3.52 (s, 6H, CH2OCH3), 3.51 (s, 6H, CH2OCH3), 1.41 (s, 18H, tert-Bu). 13C NMR (75 MHz, CDCl3): δ = 151.55, 147.60, 139.07, 138.86, 125.23, 123.04, 115.95, 114.25, 95.98, 94.74, 56.36, 56.00, 34.98, 29.75. MS: m/z = 588 (M+). HRMS (m/z): 588.2736 (M+, calcd. 588.2757 for C32H44O8S).
Synthesis of 2,5-bis(4-tert-butyl-2,5-dihydroxyphenyl)thiophene (7). A solution of 12 (0.195 g, 0.331 mmol) and concentrated HCl (1 mL) in MeOH (30 mL) was refluxed for 20 h in the dark. After water was added, the mixture was extracted with ethyl acetate. Combined organic phase was washed with saturated aqueous NaHCO3 and brine, dried over MgSO4, and evaporated to dryness. From the crude product, 7 (0.124 g, 91%) was isolated by recrystallization from Et2O and n-hexane as a slightly purple solid.
Mp 214-216 °C. 1H NMR (300 MHz, CDCl3): δ = 7.28 (s, 2H, Ar), 6.88 (s, 2H, Ar), 6.79 (s, 2H, Ar), 5.16 (s, 2H, OH), 4.74 (s, 2H, OH), 1.41 (s, 18H, tert-Bu). 1H NMR (300 MHz, (CD3)2CO): δ = 8.17 (s, 2H, OH), 7.90 (s, 2H, OH), 7.49 (s, 2H, Ar), 7.11 (s, 2H, Ar), 6.90 (s, 2H, Ar), 1.39 (s, 18H, tert-Bu). 13C NMR (75 MHz, (CD3)2CO): δ = 149.62, 147.05, 139.59, 136.94, 125.87, 119.94, 115.92, 115.39, 35.06, 29.75. MS: m/z = 412 (M+). HRMS (m/z): 412.1717 (M+, calcd. 412.1708 for C24H28O4S). IR (Nujol, cm–1): 3626, 3497, 3341, 3182 (νO–H).
Synthesis of 2,5-bis(5-tert-butyl-1,4-benzoquinon–2–yl)thiophene (QTQ). To a solution of 7 (0.680 g, 1.65 mmol) in MeCN (40 mL) was added CAN (2.75 g, 5.02 mmol) at ambient temperature in the dark. After stirring for 4 h, the reaction mixture was quenched with water, and subjected to supersonic treatment until the solid was precipitated. The precipitate was separated by suction filtration, washed with water, and dried to give analytically pure QTQ as a reddish purple powder (0.606 g, 90%).
Mp 265-267 °C. 1H NMR (300 MHz, CDCl3): δ = 7.71 (s, 2H, Ar), 6.98 (s, 2H, C=CH), 6.68 (s, 2H, C=CH), 1.32 (s, 18H, tert–Bu). 13C NMR (75 MHz, CDCl3): δ = 187.15, 186.79, 156.35, 138.92, 136.40, 131.51, 130.19, 130.02, 35.22, 29.15. MS: m/z = 408 (M+). HRMS (m/z): 408.1395 (M+, calcd. 408.1395 for C24H24O4S). IR (Nujol. cm–1): 1653, 1635 (νC=O).
Theoretical calculations. Geometry optimizations were conducted with Spartan PC '04 software package on Microsoft Window XP. In all calculations, B3LYP/6-31G** levels were employed and the symmetry of the conformers of QQ', QPQ', and QTQ' were assumed as shown in Table 1.
ACKNOWLEDGMENTS
This work was financially supported by the Saneyoshi Scholarship Foundation, Japan. The authors appreciate the useful advice given by Dr. Yen Ting Chen (Scripps Florida).
References
1. H. Meier, Angew. Chem. Int. Ed., 2005, 44, 2482. CrossRef
2. U. H. F. Bunz, Chem. Rev., 2000, 100, 1605. CrossRef
3. U. H. F. Bunz, Adv. Polym. Sci., 2005, 177, 1.
4. W. Zhang and J. S. Moore, Angew. Chem. Int. Ed., 2006, 45, 4416. CrossRef
5. H. Doucet and J.-C. Hierso, Angew. Chem. Int. Ed., 2007, 46, 834. CrossRef
6. F. Diederich, P. J. Stang, and R. R. Tykwinski, ‘Acetylene Chemistry. Chemistry, Biology and Material Science’, Wiley-VCH, Whinheim, 2005.
7. N. Robertson and C. A. McGowan, Chem. Soc. Rev., 2003, 32, 96. CrossRef
8. P. F. H. Schwab, M. D. Levin, and J. Michl, Chem. Rev., 1999, 99, 1863. CrossRef
9. F. J. M. Hoeben, P. Jonkheijm, E. W. Meier, and A. P. H. J. Schenning, Chem. Rev., 2005, 105, 1491. CrossRef
10. N. Hayashi, T. Ohnuma, Y. Saito, H. Higuchi, and K. Ninomiya, Tetrahedron, 2009, 65, 3639. CrossRef
11. N. Hayashi, T. Yoshikawa, T. Ohnuma, H. Higuchi, K. Sako, and H. Uekusa, Org. Lett., 2007, 9, 5417. CrossRef
12. N. Miyaura and A. Suzuki, Chem. Rev., 1995, 95, 2457. CrossRef
13. I. Cepanec, ‘Synthesis of Biaryls’, Elsevier, Oxford, 2004.
14. G. Giorgioni, S. Ruggieri, F. Claudi, A. Di Stefano, E. Ljung, and T. Carlsson, Med. Chem., 2008, 4, 1. CrossRef