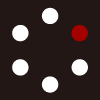
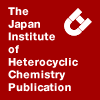
HETEROCYCLES
An International Journal for Reviews and Communications in Heterocyclic ChemistryWeb Edition ISSN: 1881-0942
Published online by The Japan Institute of Heterocyclic Chemistry
e-Journal
Full Text HTML
Received, 11th March, 2009, Accepted, 20th April, 2009, Published online, 21st April, 2009.
DOI: 10.3987/COM-09-11704
■ First Synthesis of Racemic Concentricolide, an Anti-HIV-1 Agent Isolated from the Fungus Daldinia concentrica
Li-Zhen Fang and Ji-Kai Liu*
State Key Laboratory of Phytochemistry and
Plant Resources in West China, Kunming Institute of Botany, Chinese Academy of Sciences, Heilongtan, Kunming 650204, Yunnan, China
Abstract
Concentricolide, a novel compound with anti-HIV-1 activity and isolated from ascomycete Daldinia concentrica, has been synthesised for the first time as a racemate from furan-3-carbaldehyde and dihydrofuran-2(3H)-one via a Diels-Alder reaction. The identity of the synthetic sample was verified by comparison of its spectral data with those of an authentic sample.Concentricolide (1) is a new natural product isolated by us from ascomycete Daldinia concentrica in 2005, and found to exhibit significant anti-HIV-1 activity.1 It inhibited HIV-1-induced cytopathic effects with an EC50 value of 0.31 μg/mL. The therapeutic index (TI) of this product was 247. Concentricolide is also effective in the blockage (EC50 0.83 μg/mL) of syncytium formation between HIV-1 infected cells and normal cells. In the following investigation, several new compounds with this type of structure were isolated from the culture broth of D. concentrica.2,3 Because these compounds might show promise as antiviral agents3 and anti-AIDS agents, synthetic studies are needed. Herein, we describe the first synthesis of (±)-concentricolide (1).
Our synthetic strategy is presented in Scheme 1. (±)-Concentricolide (1) could be obtained via a Diels-Alder reaction with known precursors 3-vinylfuran (3) and 4-ethylbut-2-en-4-olide (4), which are derived from commercially available 3-carbaldehyde (5) and 2-(trimethylsiloxy)furan (6), respectively. 3-Vinylfuran (3) was obtained as a mixture via a Grignard reaction that occurred under refluxing with Mg turnings and TMSCH2Cl in dry Et2O, under nitrogen. This was followed by vigorous stirring with aqueous 1.0 M HCl until all traces of 5 disappeared, as observed by TLC (AcOEt: hexane 1:5). This mixture was kept at -20 °C, protected from light, and used directly in the Diels-Alder reaction. 2-Trimethylsiloxyfuran (2) was alkalated with iodoethane in the presence of a molar excess of silver trifluoroacetate to give the 4-ethylbut-2-en-4-olide (4) in 60% yield. Unfortunately, the reaction between synthons 3 and 4 did not give the anticipated Diels-Alder product. Instead, a complex mixture of compounds resulted despite repeated trials, and with added Lewis acid catalyst BF3.Et2O. This outcome indicated that the two synthons were mismatched,and the reactivity of synthon 4 with synthon 3 must be improved to give the Diels-Alder product. Addition of an α-sulfinyl group to the electron-withdrawing group of the dienophile can be used to increase reactivity in normal Diels-Alder reactions.4 The desired adduct was formed by the addition of the dienophile to the furan 2,3-double bond 3-vinyl group diene system.5 An added advantage of this activating group was observed: it readily underwent elimination after the cycloaddition, and introduced the double bond. We thus altered synthons 4 to 12 in this way, as shown in the synthetic pathway depicted in Scheme 2.
Preparation of 5-ethyl-3-(phenylsulfinyl)furan-2(5H)-one (12) began with inexpensive dihydrofuran-2(3H)-one (7) using a modification of the method of outlined by Sweeney and Smith.7,8 Bromination of dihydrofuran-2(3H)-one gave 3-bromo-5-ethyl-dihydrofuran-2(3H)-one (8), which underwent nucleophilic attack by thiophenol to offer 5-ethyl-3-(phenylthio)-dihydrofuran-2(3H)-one (9) in excellent yield. α-Chlorination of 9 with N-chlorosuccinimide (NCS) provided 3-chloro-5-ethyl-3-phenylthio-dihydrofuran-2(3H)-one (10) in 82% yield, and this compound underwent conjugative elimination of HCl upon reaction with a mixture of lithium bromide and lithium carbonate in refluxing THF to give 5-ethyl-3-(phenylthio)furan-2(5H)-one (11) in 35% yield. The corresponding sulphoxide was prepared via m-CPBA oxidation of 11 in 82% yield.
The Diels-Alder reaction was carried out in the presence of 10.67 mg of hydroquinone to prevent the formation of a complex mixture.9 Treatment of a solution of 3 in dry toluene at reflux with three equivalents of 12 in for 72 h afforded 13, which was immediately stirred with calcium carbonate in anhydrous toluene without isolation for 19 h at 90 ºC, providing the precursor by elimination of PhSOH. The precursor mixtures were swiftly converted into the concentricolide by oxidation, which occurred during purification by column chromatography, affording the target product 1. In our experiment, only about 7.6 mg of concentricolide was obtained from the 91.0 mg (0.97 mmol) of 3 and 677.6 mg (2.87 mmol) of 12. It was noted that the yield was low and the further improvements are the subject of ongoing research.
EXPERMENTAL
General Experimental Procedures
Optical rotation was measured using a Horiba SEPA-300 polarimeter. IR spectra were obtained on a Bruker Tensor 27 with KBr pellets. NMR spectra were recorded on Bruker AM-400 and Bruker DRX-500 spectrometers. EI-MS was recorded with a VG Autospec-3000 spectrometer and HR-ESI-MS was recorded with an API QSTAR Pulsar 1 spectrometer. Silica gel (200-300 mesh, Qingdao Marine Chemical Inc., China) was used for column chromatography. Fractions were monitored by TLC and spots were visualised by heating silica gel plates sprayed with 10% H2SO4 in ethanol. All commercial agents were produced by Sigma-Aldrich.
Synthesis of 3-Vinylfuran (3): Chlorotrimethylsilane (1.02 g, 8.28 mmol) was added dropwise to magnesium turnings (0.24 g, 9.9 mmol) in dry Et2O (5 mL) under nitrogen, and the mixture was refluxed (45 °C) for 9 h, then cooled to 0 °C and treated with a solution of furan-3-carbaldehyde (0.69 g, 7.2 mmol) in dry Et2O (7 mL). After stirring for 4 h at 0 °C and then 14 h at 23 °C, the reaction mixture was quenched with sat. aq. NH4Cl (5 mL) at 0 °C. The layers were separated, the aqueous layer was extracted with Et2O (3 × 5 mL), and the combined organic layers were washed with sat. aq. NaHCO3 (2 × 5 mL), brine (10 mL), and dried over Na2SO4. Following solvent evaporation in vacuo, the residue was dissolved in Et2O (5 mL) and stirred vigorously with 1.0 M HCl (3.4 mL) for 80 min. until it was not visible by TLC (AcOEt: hexane =1:5). The layers were separated, the aqueous layer was extracted with Et2O (2 × 5 mL), and the combined organic layers were washed with sat. aq. NaHCO3 (2 × 5 mL) and brine (5 mL), and dried over Na2SO4. The solution was carefully concentrated in vacuo to ca. 5 mL with no heating to give the product (676.9 mg, ca. 50%) as a mixture with TMSOH and Et2O. This mixture was kept at -20 °C, protected from light and was used immediately. 1H NMR (500 MHz, CDCl3): δ 7.10 (br s, 1H), 7.04 (br s, 1H), 6.23 (br s, 2H), 5.13 (dd, 1H, J = 16 Hz, 7.5 Hz), 4.78 (dd, 1H, J =7.5 Hz); 13C NMR (125 MHz, CDCl3): δ 142.78 (CH), 139.90 (CH), 126.07 (CH), 124.34 (C), 112.23 (CH2), 106.50 (CH).
Synthesis of 3-bromo-5-ethyldihydrofuran-2(3H)-one (8): PBr3 (1.44 g, 5.31 mmol) was combined with dihydrofuran-2(3H)-one (6.28 g, 55.09 mmol) in an ice bath, stirred for 20 min, treated with Br2 (8.80 g, 55.09mmol), and stirred for an additional 30 min at rt. The mixture was heated at 90 ºC, and Br2 (8.80 g, 55.00 mmol) was added. After stirring for 4 h, the mixture was poured into ice water (250 mL), and washed with sat. aq. NaHCO3 until the red-brown colour disappeared. The layers were separated, the aqueous layer was extracted with Et2O (3 × 20 mL), and the combined organic layers were washed with sat. aq. NaHCO3 (2 × 5 mL), brine (5 mL), and dried over Na2SO4. The solution was concentrated in vacuo, and the crude product was purified by silica gel column chromatography using petroleum ether-AcOEt (20:1) to afford the product as an oil (6.06 g, 57%). IR νmax (KBr) cm-1: 2971, 2939, 1782, 1185, 965, 946, 669. FAB-MS m/z (%): 193 (3), 109 (20), 95 (35), 81 (51), 69 (69), 55 (100). HR-ESI-MS m/z: C6H9O2NaBr, [M+Na]+ (found: 214.9694, calcd.: 214.9683). 1H NMR (400 MHz, CDCl3): δ 4.69 (m, 1H), 4.46 (dd, 1H, J = 6.7 Hz, 1.5 Hz), 2.55 (m, 1H), 2.41 (m, 1H), 1.78 (m, 1H), 1.04 (m, 3H). 13C NMR (100 MHz, CDCl3): δ 172.48 (C), 80.86 (CH), 39.01 (CH), 38.84 (CH2), 27.15 (CH2), 9.16 (CH3).
Synthesis of 5-ethyl-3-(phenylthio)dihydrofuran-2(3H)-one (9): PhSH (3.93 g, 35.73 mmol) and Et3N (3.61 g, 35.73 mmol) were added to a solution of 3-bromo-5-ethyldihydrofuran-2(3H)-one (6.86 g, 35.73 mmol) in dry THF (50 mL) at 0 ºC over 30 min. The mixture was heated to reflux and stirred for 10 h, then partitioned between water (100 mL) and Et2O (90 mL). The layers were separated, and the aqueous phase was extracted with Et2O (90 mL). The combined organic layers were washed with brine, dried over anhydrous Na2SO4 to give 9 as yellow oil (7.79g, 98%). IR νmax (KBr) cm-1: 2970, 2938, 1772, 1480, 1462, 1440, 1189, 948, 746, 692. FAB MS m/z (%): 223 (100), 177 (24), 149 (5), 113 (7), 57 (9). HR-ESI-MS m/z: C12H15O2S, [M+H]+, (found: 223.0790, calcd.: 223.0792). 1H NMR (500 MHz, CDCl3): δ 7.50 (m, 2H), 7.29 (m, 3H), 4.30 (m, 1H), 3.98-3.86 (m, 1H), 2.27 (m, 1H), 1.84-1.50 (m, 3H), 0.91 (m, 3H). 13C NMR (125 MHz, CDCl3): δ 174.61 (C), 133.00 (CH), 131.72 (C), 128.94 (CH), 80.26 (CH), 44.90 (CH), 35.06 (CH2), 27.96 (CH2), 9.14 (CH3).
Synthesis of 3-chloro-5-ethyl-3-phenylthiodihydrofuran-2(3H)-one (10): To a solution of 5-ethyl-3-(phenylthio)dihydrofuran-2(3H)-one (10.21 g, 45.99 mmol) in CCl4 (90 mL) under nitrogen was added N-chlorosuccinimide (6.16 g, 46.14 mmol). After refluxing for 8 h, water (50 mL) was poured into the reaction mixture, the layers were separated, and the aqueous phase was extracted with CH2Cl2. The combined organic layers were washed with sat. aq. NaHCO3 (2 × 50 mL), brine (50 mL), and water (50 mL), dried over Na2SO4, and concentrated in vacuo to give the crude product. The product was further purified by silica gel column chromatography using petroleum ether-AcOEt (20:1) to afford 10 as a golden oil (9.75 g, 82%). IR νmax (KBr) cm-1: 2972, 2938, 1775, 1440, 1350, 1185, 1024, 956, 751, 691. FAB-MS m/z (%): 257 (25), 221 (100), 95 (35), 175 (22), 69 (17), 57 (25). HR-ESI-MS m/z: C12H14O2SCl, [M+H]+ (found: 257.0405, calcd.: 214.0403) 1H NMR (400 MHz, CDCl3): δ 7.71-7.34 (m, 5H), 4.58 (m, 1H), 2.90-2.26 (m, 2H), 1.88-1.60 (m, 2H), 1.05-0.85 (m, 3H). 13C NMR (100 MHz, CDCl3): δ 168.73 (C), 136.64 (CH), 143.84 (CH), 133.82 (CH), 132.85 (C), 129.10 (CH), 83.56 (CH), 46.34 (CH2), 27.68 (CH2), 9.20 (CH3).
Synthesis of 5-ethyl-3-(phenylthio)furan-2(5H)-one (11): Lithium bromide (354.8 mg, 4.08 mmol) and lithium carbonate (1.50 g, 20.27 mmol) were dried for 3 h at 90 ºC under reduced pressure, and added to a solution of 3-chloro-5-ethyl-3-phenylthiodihydrofuran-2(3H)-one (5.23 g, 20.35 mmol) in anhydrous THF (40 mL). The mixture was heated to the point of reflux for 30 min until it became orange-brown in colour. The inorganic salts were filtered off by passage through Celite, and the organic solution was washed with sat. aq. NaHCO3 (50 mL), brine (50 mL), water (50 mL), and dried over Na2SO4. The solution was concentrated in vacuo and the residue was purified via silica gel column chromatography using petroleum ether-AcOEt (15:1) to afford 11 as a yellow oil (1.59 g, 35%). IR νmax (KBr) cm-1: 2972, 2938, 1775, 1440, 1350, 1185, 1024, 956, 751, 691. FAB-MS m/z (%): 221 (100), 203 (28), 175 (22), 175 (22), 111 (15), 97 (22), 83 (30), 69 (37), 55 (70). EI-MS m/z (%): 220 (76), 191 (29), 164 (62), 135 (100), 111 (18), 91 (33), 77(10). HR-ESI-MS m/z: C12H13O2S, [M+H]+ (found: 221.0644, calcd.: 221.0636). 1H NMR (400 MHz, CDCl3): δ 7.54 (m, 2H), 7.42 (m, 3H), 6.55 (d, 1H, J = 1.8 Hz), 1.83-1.61 (m, 2H), 0.95 (t, 3H, J = 7.5 Hz). 13C NMR (100 MHz, CDCl3): δ 169.55 (C), 144.26 (CH), 133.26 (CH), 131.91 (C), 129.47 (CH), 128.94 (CH), 83.30 (CH), 26.22 (CH2), 8.54 (CH3).
Synthesis of 5-ethyl-3-(phenylsulfinyl)furan-2(5H)-one (12): A solution of 3-chloro-5-ethyl-3-phenylthiodihydrofuran-2(3H)-one (0.698 g, 3.17 mmol) in CH2Cl2 (25 mL) was added to a solution of 86% m-chloroperoxybenzoic acid (m-CPBA) (643.6 mg, 3.17 mmol) in CH2Cl2 (9 mL), and the reaction mixture was stirred for 1 h at -10 ºC. Additional CH2Cl2 (25 mL) and 10% aqueous NaHSO3 (15 mL) were added, and the layers were separated. The organic phase was washed with 10% aqueous NaHCO3, 10% brine, and dried over Na2SO4. The solution was concentrated in vacuo, and the residue was purified via silica gel column chromatography using petroleum ether-AcOEt (15:1) to afford 12 as a yellow oil (614.6 mg, 82% yield). HR-ESI-MS m/z: C12H12O3NaS, [M+Na]+ (found: 259.0394, calcd.:, 259.0404). EI-MS m/z (%): 236 (15), 188 (15), 125 (96), 111 (60),103 (33), 97 (42), 77 (100), 65 (38), 57 (55); 1H NMR (400 MHz, CDCl3): δ 7.83 (m, 1H), 7.63 (m, 2H), 7.33 (m, 3H, J = 1.8 Hz), 4.99-4.80 (t, 1H, J = 6.1 Hz),1.75-1.45 (m, 2H), 0.83-0.66 (t, 3H, J = 7.4 Hz). 13C NMR (100 MHz, CDCl3): δ 165.87 (C), 154.46 (CH), 140.75 (C), 131.60 (CH), 128.91(CH), 83.20 (CH), 25.37 (CH2), 8.11 (CH3).
Synthesis of Concentricolide (1): 5-Ethyl-3-(phenylsulfinyl)furan-2(5H)-one (677.6 mg, 2.87 mmol) and hydroquinone (10.67 mg, 0.097 mmol) were added to a solution of 3-vinylfuran (91.0 mg, 0.97 mmol) in dry toluene (1.5 mL) and stirred for 72 h at rt. CaCO3 (9.7 mg, 0.97 mmol) was added, and the reaction mixture was heated to 90 °C with stirring for 15 h. The solvent was evaporated in vacuo, and the residue was purified via silica gel column chromatography using petroleum ether-AcOEt (10:1) before the product was crystallised (7.6 mg, 3.8%). HR-ESI-MS m/z: 225.0522, [M+Na]+, 225.0523 calcd. for C12H10O3Na); 1H NMR (500 MHz, CDCl3): δ 7.92 (dd, 1H, J = 8.0, 3.6 Hz ), 7.80 (d, 1H, J = 2.1 Hz ), 6.91 (d, 1H, J = 2.1 Hz), 5.58 (dd, 1H, J = 7.0 Hz, 4.1 Hz), 2.19 (m, 1H), 1.87 (m, 1H), 1.01 (t, 3H, J = 7.4 Hz). 13C NMR (125 MHz, CDCl3): δ 169.14 (C), 146.58 (CH), 127.88 (C), 116.04 (CH), 106.64 (CH), 82.97 (CH), 27.89 (CH2), 8.81 (CH3). These data were in agreement with those reported for an authentic sample.1
ACKNOWLEDGMENTS
We thank Prof. Da-Wei Ma and Hong-Bin Zhang for their kind and useful advice on synthetic experiments. This project was supported by National Basic Research Program of China (973 Program, 2009CB522300), National Natural Science Foundation of China (30830113), and Chinese Academy of Sciences (KSCX1-YW-R-24; KSCX2-YW-G-025).
References
1. (a) X. D. Qin, Z. J. Dong, J. K. Liu, L. M.Yang, R. R. Wang, T. T. Zheng, Y. Lu, Y. S. Wu, and Q. T. Zheng, Helv. Chim. Acta, 2006, 89, 127; CrossRef (b) J. K. Liu, Y. T. Zheng, X. D. Qin, L. M. Yang, Z. J. Dong, and R. R. Wang, WO2005/037841.
2. H. J. Shao, X. D. Qin, Z. J. Dong, H. B. Zhang, and J. K. Liu, J. Antibiot., 2008, 61, 115. CrossRef
3. X. D. Qin, H. J. Shao, Z. J. Dong, and J. K. Liu, J. Antibiot., 2008, 61, 556. CrossRef
4. M. Fardis, M. Mertzman, W. Thomas, T. Kirschberg, N. Collins, R. Polniaszek, and W. J. Watkins, J. Org. Chem., 2006, 71, 4835. CrossRef
5. G. J. L. Ruano, M. T. Peromingo, M. Alonso, A. Fraile, M. R. Martin, and A. Tito, J. Org. Chem., 2005, 70, 8942. CrossRef
6. A. Benítez, F. R. Herrera, M. Romero, and F. X. Tamás, J. Org. Chem., 1996, 61, 1487. CrossRef
7. G. J. Hollingworth, G. Perkins, and J. Sweeney, J. Chem. Soc., Perkin Trans. 1, 1996, 1913. CrossRef
8. J. A. Kaydos and D. L. Smith, J. Org. Chem., 1983, 48, 1096. CrossRef
9. T. Sasaki, Y. Ishibashi, and M. Ohno, Heterocycles, 1983, 20, 1933 CrossRef