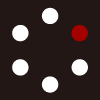
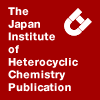
HETEROCYCLES
An International Journal for Reviews and Communications in Heterocyclic ChemistryWeb Edition ISSN: 1881-0942
Published online by The Japan Institute of Heterocyclic Chemistry
e-Journal
Full Text HTML
Received, 24th March, 2009, Accepted, 15th May, 2009, Published online, 18th May, 2009.
DOI: 10.3987/COM-09-11713
■ Reactions of 9-Aryl-6-cyanopurines with Primary Amines
Amal Al-Azmi* and K. Anita Kumari
Department of Chemistry, Faculty of Science, Kuwait University, Safat 13060, P.O. Box 5969, Kuwait
Abstract
Two structural isomers (9-aryl-6-cyanopurines and imidazole-4,5-dicarbonitriles) were isolated from the reaction of (Z)-N1-aryl-N2-(2-amino-1,2-dicyanovinyl)formamidines with triethyl orthoacetate or propionate. On the other hand, 9-aryl-6-cyanopurines were the only product, when triethyl orthoformate was used. The reaction of 9-aryl-6-cyanopurines with hydroxylamine hydrochloride in dichloromethane/ethanol at room temperature furnished 6-amidinopurines, while reaction with primary amines afforded pyrimido[5,4-d]pyrimidines. In addition, 9-aryl-6-cyanopurines reacted with hydrazine monohydrate under mild conditions to give 4-imino-N8-arylpyrimido[5,4-d]pyrimidines. The latter furnished novel pyrimido[4,5-e][1,2,4]triazolo[1,5-c]pyrimidines when refluxed with an excess of triethyl orthoesters. The new compounds were fully characterized and single crystal X-ray analyses have been carried out on 9-(4-methoxyphenyl)-9H-purine-6-carboximidamide and 2-methyl-1-[(E)-p-tolyliminomethyl]-1H-imidazole-4,5-dicarbonitrile.INTRODUCTION
Purines continue to gain importance due to their biologically active nature. A number of reports have described several methods to synthesize various purine derivatives that played a significant role in drug development.1-15 One of these compounds is 6-cyanopurine which has been used as a versatile reagent for the synthesis of a wide range of 6-substituted purines through the modification of the cyano group. 6-Substituted purines have been shown to have potency as antimetabolites,16-18 antiepileptic, and antiarrhythmic agents.19-22 The biological importance of these compounds extends to the mechanism of enzyme-mediated purine interconversion.23 As a result, the development of selective and efficient methods for the modification of the cyano function is desirable. One of the modifications reported in the literature is the conversion of 6-cyanopurines 2 to 6-amidinopurines 3 (Scheme 1).4-15
Higashino et al. demonstrated that 6-amidinopurines 3 could be obtained in good yields when 9-aryl-6-cyanopurines 2 react with n-butylamine, hydrazine monohydrate, piperidine and hydroxylamine hydrochloride in methanol.7 However, a careful investigation of these reactions showed that 6-amidinopurines 3 are formed only when hydroxylamine hydrochloride is used, while other amines afforded pyrimido[5,4-d]pyrimidines 4.6 6-Amidinopurines 3 (some as hydrochloride salts) were also synthesized through a two-step conversion of 6-cyanopurines 2 to 6-alkoxyformimidoylpurines 6 followed by treatment with methylamine hydrochloride, whereas reactions of 6-cyanopurines 2 with other primary amines gave pyrimido[5,4-d]pyrimidines 4.4-6,15
Generally, direct isolation of 6-amidinopurines 3 from the reaction of 6-cyanopurines 2 with primary amines other than hydroxylamine hydrochloride has been reported to be difficult, as these reactions lead mainly to pyrimido[5,4-d]pyrimidines 4.6 So far, the reason behind the formation of either pyrimido[5,4-d]pyrimidines or 6-amidinopurines is still not clear. This prompted us to reinvestigate the reaction of 9-aryl-6-cyanopurines with primary amine salts under various conditions.
RESULTS AND DISCUSSION
9-Aryl-6-cyanopurines 2a,b were prepared in good yields following the literature procedure.4 In an attempt to prepare 2-methyl-9-(4-tolyl)-6-cyanopurine 2d from refluxing formamidine 1a with triethyl orthoacetate for 2 hours, a solid was formed after cooling the reaction mixture. The 1H NMR analysis of the solid revealed the presence of 6-cyanopurine 2d and another unknown compound in (2:3) ratio, respectively. X-ray analysis on the unknown product showed that the compound is 2-methyl-1-[(E)-p-tolyliminomethyl]-1H-imidazole-4,5-dicarbonitrile 7d (Scheme 2 and Figure 1).
To generalise this observation, another two reactions were carried out and it was found that both formamidines 1a,b furnished the two structural isomers, i.e. 6-cyanopurine 2c,e and imidazole-4,5-dicarbonitrile 7c,e when refluxed with triethyl orthopropionate. It is possible that the difference in the product of reaction of triethyl orthoformate and other orthoesters is the result of the difference in the rate of the formation of reactive oxocarbenium ion intermediates from the orthoesters. Thus, the product ratios arise from two factors stemming from alkyl groups: stabilization of an oxocarbenium intermediate and release of steric strain from the more hindered orthoesters seems to facilitate elimination of the ethoxy group to provide highly reactive species that would rapidly undergo imidation to provide 8 (Route A), which would eventually afford imidazole derivative 7. This process is, however, unfavourable for orthoformate having no such alkyl group. As a result, formation of imidate 8 in this case becomes slow and therefore Route B is more pronounced to provide cyanopurines selectively (Scheme 3).
We then turned our attention to study the reaction of 6-cyanopurines with primary amine hydrochlorides (Scheme 4). A mixture of 6-cyanopurine 2a, hydroxylamine hydrochloride (5 equiv.), and sodium acetate (4 equiv.) in dichloromethane was stirred at room temperature. After stirring for 18 hours, TLC indicated the completion of the reaction. Formation of either of the two structural isomers, 6-amidinopurine 3a or pyrimido[5,4-d]pyrimidine 9, was expected. The 1H NMR spectrum resembles the spectra of several known 6-amidinopurines derivatives reported previously.6a On the other hand, the 1H NMR spectrum of compound 9 would be expected to show three D2O exchangeable singlets each for one proton. This 1H NMR result is more consistent with structure 3.
Further, X-ray analysis confirmed the structure of product 3a as 9-(4-methoxyphenyl)-9H-purine-6-carboximidamide (Figure 2 and Figure 3).
Under similar reaction conditions, 9-(4-tolyl)-6-cyanopurine 2b gave 6-amidinopurine 3b (Scheme 4).
Recently, the formation of 6-amidinopurines from the reaction of 6-cyanopurines with hydroxylamine hydrochloride and sodium acetate in refluxing methanol has been described.6a The use of methanol in an acidic medium was postulated to be the reason behind the conversion of the cyano group to an imidate intermediate, which facilitated the nucleophilic reaction. Isolation of 6-amidinopurines 3a,b at room temperature, using dichloromethane as solvent, suggests that using methanol might not be crucial for an in situ formation of the imidate intermediate. The results obtained in this study present two important findings: Firstly, methanol does not seem to have a significant role in the formation of 6-amidinopurines, and that there might be other factors influencing the reaction pathway. And secondly, using different amine hydrochlorides, under the same conditions used to synthesize 3a,b might afford 6-amidinopurines. Consequently, reaction of 9-aryl-6-cyanopurines with methylamine hydrochloride was investigated, in order to determine the role of acid catalysts in the formation of 6-amidinopurines directly from 6-cyanopurines.
When 6-cyanopurine 2a was reacted with methylamine hydrochloride under the same conditions used to prepare compounds 3a,b (Table 1, entry 5), a mixture of purine 2a and a trace of the rearranged pyrimido[5,4-d]pyrimidine 4 were obtained as evidenced by 1H NMR analysis. On the other hand, the 6-cyanopurine 2a was recovered when ethanol was used. The Dimroth rearranged pyrimido[5,4-d]pyrimdine 5 was isolated when a mixture of 6-cyanopurine 2a, methylamine hydrochloride (5 equiv.) and sodium acetate (4 equiv.) was refluxed in ethanol. These results indicate that neither the alcohol nor the acid catalyst are important factors in the formation of 6-amidinopurines 3 (Table 1).
The reason why hydroxylamine hydrochloride behaves in a different way is not yet clear. Nevertheless, several studies carried out by Kirby and his co-workers on the nature of hydroxylamine demonstrated that this ambident α–effect nucleophile exists in its crystal structure as a mixture of equal amount of both H3N+OH and H3N+O¯, where H3N+O¯ is the more stable ion.24,25a Also, a recent study showed that hydroxylamine acts as an oxygen nucleophile.25b
The reaction of hydroxylamine through the oxygen site has also been noticed in both acylation and phosphorylation by other researchers.26-28 This shows that in its reactions, hydroxylamine favours hard electrophiles. Furthermore, Kukushkin et al.29 have investigated the reaction rate of hydroxylamine with nitriles and oximes, and noticed that it is more reactive towards addition to nitriles than oximes by a factor of 1.7 × 104.
It is conceivable that the two isomeric forms observed by Kirby in the solid state may also be observed in the present reaction. In addition, it has been reported previously that oxygen nucleophiles such as ethanol and methanol attack the cyano function and not the C-8 in the 6-cyanopurine.5,6 It is possible that the C≡N function in the 6-cyanopurine is attacked by the tautomer H3N+O¯ via its oxygen site to form an imidate intermediate in situ, which would make the carbon more electrophilic. It is also possible that the buffer behaviour of the medium would allow the exchange with the acetate to furnish 6-amidinopurines 3.
At room temperature, 6-cyanopurines 2a,b reacted with an excess of hydrazine monohydrate in dichloromethane. After 20 hours stirring, the expected pyrimido[5,4-d]pyrimidines 10 precipitated from the reaction mixture in good yields (Scheme 5).
The structure of pyrimido[5,4-d]pyrimidines 10a,b suggests that these molecules could be utilised as precursors to synthesize novel triheterocyclic compounds, and thus opens new routes to potentially important heterocycles. Analogues of triheterocyclic compounds have been shown to be useful in cancer therapy.30-32 In addition, the use of pyrimido[5,4-d]pyrimidines-based derivatives in medicine as antiviral and antitumour agents have been widely described by several authors.8-15, 33-35 When pyrimido[5,4-d]pyrimidines 10a,b were refluxed with an excess of triethyl orthoesters for 6-24 hours, bright yellow solids started to precipitate on cooling. The products 11a-f were isolated in moderate yields.
CONCLUSION
This work shows that two structural isomers i.e. 6-cyanopurines and imidazole-4,5-dicarbonitriles are formed from the reaction of (Z)-N1-aryl-N2-(2-amino-1,2-dicyanovinyl)formamidines with triethyl orthoesters. On the other hand, reaction with triethyl orthoformate afforded only 6-cyanopurines. Introducing alkyl groups was found to facilitate the formation of the imidazoles. Furthermore, generation of 6-amidinopurines from the reaction of 6-cyanopurines with hydroxylamine hydrochloride is found to be independent of both alcohol and an acidic medium, but depends on the type of nucleophile used such as an α–effect nucleophile like hydroxylamine which attacks hard electrophiles through its zwitterion H3N+O¯. Also, reaction of 6-cyanopurines with hydrazine monohydrate under mild conditions led to pyrimido[5,4-d]pyrimidines. These were used as intermediates to synthesize novel pyrimido[4,5-e][1,2,4]triazolo[1,5-c]pyrimidines in moderate yields. Finally, the obtained results contribute to the synthesis of a new series of heterocyclic compounds which are potentially useful in the pharmaceutical industry.
EXPERIMENTAL
Crystal Data36
Compound 3a
Chemical formula (moiety) C13H12N6O2, chemical formula (total) C13H12N6O2, formula weight 284.29, temperature 150(2) K, radiation, wavelength MoKα, 0.71073 Å, crystal system, space group monoclinic, P21/n, unit cell parameters a = 10.586(3) Å, α = 90°, b = 8.231(2) Å, β = 92.244(5)°, c = 14.605(4) Å, γ = 90°, cell volume 1271.7(6) Å3, Z 4, calculated density 1.485 g/cm3, absorption coefficient μ 0.107 mm−1, F(000) 592, crystal colour and size: colourless, 0.12 × 0.10 × 0.05 mm3, reflections for cell refinement 2938 (θ range 2.2 to 28.2°), data collection method Bruker SMART 1K CCD diffractometer thin-slice ω scans, θ range for data collection 2.3 to 25.0°, index ranges h −12 to 12, k −9 to 9, l −16 to 17, completeness to θ = 25.0° 99.6 %, reflections collected 6603, independent reflections 2222 (Rint = 0.0405), reflections with F2>2σ 1714, absorption correction none, structure solution direct methods, refinement method Full-matrix least-squares on F2, weighting parameters a, b 0.0690, 0.2093, data / restraints / parameters 2222 / 0 / 204, final R indices [F2>2σ] R1 = 0.0455, wR2 = 0.1096, R indices (all data) R1 = 0.0639, wR2 = 0.1209, goodness-of-fit on F2 1.047, extinction coefficient 0.0071(17), largest and mean shift/su 0.000 and 0.000, largest diff. peak and hole 0.30 and −0.25 e Å−3.
Crystal Data37
Compound 7d
Chemical formula (moiety) C14H11N5, formula weight 249.28, temperature 150(2) K, radiation, wavelength MoKα, 0.71073 Å, crystal system, space group monoclinic, P21/n, nit cell parameters a = 7.1876(16) Å, α = 90°, b = 12.4551(12) Å, β = 99.085(18)°, c = 14.318(2) Å, γ = 90°, cell volume 1265.7(4) Å3, Z 4, calculated density 1.308 g/cm3, absorption coefficient 0.084 mm−1, F(000) 520, crystal colour and size colourless, 0.52 × 0.40 × 0.40 mm3, reflections for cell refinement 129 (θ range 2.5 to 27.5°), data collection method Nonius KappaCCD diffractometer, φ and ω scans, θ range for data collection 4.1 to 27.5°, index ranges h −9 to 9, k −16 to 16, l −18 to 18, completeness to θ = 26.0° 99.3 % , reflections collected 13867, independent reflections, 2897 (Rint = 0.0224), reflections with F2>2σ 2426, absorption correction semi-empirical from equivalents, min. and max. transmission 0.9576 and 0.9672, structure solution direct methods, refinement method Full-matrix least-squares on F2, weighting parameters a, 0.0418, 0.4658, data / restraints / parameters 2897 / 0 / 174, final R indices [F2>2σ] R1 = 0.0355, wR2 = 0.0872, R indices (all data) R1 = 0.0463, wR2 = 0.0940, goodness-of-fit on F2 1.033, largest and mean shift/su 0.000 and 0.000, largest diff. peak and hole 0.25 and −0.20 e Å−3.
General
6-Cyanopurines 2a-c, and pyrimido[5,4-d]pyrimdine 4 were prepared according to literature procedures.4,38 1H NMR spectra were recorded on a Bruker DPX 400 spectrometer at 400 MHz using CDCl3 or DMSO-d6 as a solvent and TMS as an internal standard; chemical shifts are reported in δ units (ppm). 13C NMR spectra were obtained using a Bruker DPX 400 spectrometer at 100 MHz. Mass spectra were recorded on a VG autospec Q spectrometer with a digital data output. IR was recorded on a RT-IR Perkin Elmer System 2000, using KBr discs and (νmax) was recorded in cm-1. Melting points were determined by using a Gallenkamp melting point apparatus and are uncorrected. TLC was performed on a 0.25 mm pre-coated silica gel plates (Merck).
General procedure for the synthesis of 6-cyanopurines 2d,e and imidazoles-4,5-dicarbonitrile 7c-e
Formamidines 1 (1 equiv.) were refluxed with an excess of either triethyl orthoacetate/propionate (10-15 equiv.) for 2 h. On cooling, a precipitate started to form, which was filtered and washed with petroleum ether. TLC of the precipitate showed the presence of two products (2d and 7d), which were separated by column chromatography (hexane:EtOAc) (9:1). Compounds (2c,e and 7c,e) were separated by recrystallization from EtOH.
2-Methyl-6-cyano-9-(4-methylphenyl)-9H-purine 2d: white solid (0.27 g, 1.1 mmol, 25%); mp 205-208 oC [Found: accurate mass: 249.1007; m/z (EI) M+ 249, 81%, 118, 100%, C14H11N5 requires: 249.1008; M 249]; δH 400 MHz (DMSO-d6, Me4Si) 2.42 (s, 3H, CH3), 2.76 (s, 3H, CH3), 7.44 (d, 2H, J 8 Hz, ArH), 7.73 (d, 2H, J 8 Hz, ArH), 9.19 (s, 1H, CH); δC 100 MHz (DMSO-d6, Me4Si) 162.97, 153.68, 149.44, 138.83, 133.92, 131.60, 130.56, 129.45, 124.28, 114.83, 25.98, 21.15; νmax: (KBr) 3433, 3111, 1594, 1519, 1395, 1215, 952, 811 cm−1.
2-Ethyl-6-cyano-9-(4-methylphenyl)-9H-purine 2e: colourless powder (0.83 g, 3.2 mmol, 43%); mp 125-128 oC [Found: accurate mass: 263.1164; m/z (EI) M+ 263, 95%, 262, 100%, C15H13N5 requires: 263.1165; M 263]; δH 400 MHz (DMSO-d6, Me4Si) 1.31 (t, 3H, J 7.6 Hz, -CH2CH3), 2.42 (s, 3H, CH3), 3.02 (q, 2H, J 7.6 Hz, -CH2CH3), 7.45 (d, 2H, J 8 Hz, ArH), 7.77 (d, 2H, J 8 Hz, ArH), 9.20 (s, 1H, CH); δC 100 MHz (DMSO-d6, Me4Si) 166.53, 153.17, 149.00, 138.28, 133.65, 131.26, 130.11, 129.09, 123.64, 114.46, 31.75, 20.69, 12.70; νmax: (KBr) 3115, 2988, 1585, 1519, 1395, 1338, 1210, 1202, 1174, 955, 824 cm−1.
2-Ethyl-1-[(E)-p-methoxyphenyliminomethyl]-1H-imidazole-4,5-dicarbonitrile 7c: light green needles (0.69 g, 2.5 mmol, 60%); mp 158-161 oC [Found: accurate mass 279.1114; m/z (EI) M+ 279, 76%, 134, 100%, C15H13N5O requires: 279.1114; M 279]; δH 400 MHz (DMSO-d6, Me4Si) 1.27 (t, 3H, J 7 Hz, -CH2CH3), 3.06 (q, 2H, J 7 Hz, -CH2CH3), 3.80 (s, 3H, OCH3), 7.04 (d, 2H, J 9 Hz, ArH), 7.42 (d, 2H, J 9 Hz, ArH), 8.94 (s, 1H, CH); δC 100 MHz (DMSO-d6, Me4Si) 159.09, 156.59, 139.86, 137.97, 123.85, 123.08, 114.73, 112.50, 109.48, 108.92, 55.57, 20.55, 10.82; νmax: (KBr) 2972, 2231, 1650, 1600, 1514, 1411, 1299, 1252, 1166, 1023, 1003, 839 cm−1.
2-Methyl-1-[(E)-p-tolyliminomethyl]-1H-imidazole-4,5-dicarbonitrile 7d: light green crystals (0.80 g, 3.20 mmol, 66%); mp 183-185 oC [accurate mass Found: 249.1008; m/z (EI) M+ 249, 73%, 118, 100%, C14H11N5 requires: 249.1008; M 249]; δH 400 MHz (DMSO-d6, Me4Si) 2.35 (s, 3H, CH3), 2.70 (s, 3H, CH3), 7.29 (d, 2H, J 8.7 Hz, ArH), 7.31 (d, 2H, J 8.7 Hz, ArH), 8.88 (s, 1H, CH); δC 100 MHz (DMSO-d6, Me4Si) 152.65, 142.64, 141.31, 137.07, 129.85, 123.05, 121.93, 112.16, 109.15, 108.62, 20.61, 13.86; νmax: (KBr) 3435, 2239, 2227, 1654, 1523, 1401, 1366, 1327, 1303, 1235, 966 cm−1.
2-Ethyl-1-[(E)-p-tolyliminomethyl]-1H-imidazole-4,5-dicarbonitrile 7e: off-white needles (0.56 g, 2.20 mmol, 51%); mp 155-157 oC [Anal. Calcd for C15H13N5: C, 68.44; H, 4.94; N; 26.62; Found: C, 68.20; H, 4.85; N, 26.66; m/z (EI) M+ 263, 76%, 118, 100%, M 263]; δH 400 MHz (DMSO-d6, Me4Si) 1.26 (t, 3H, J 7.4 Hz, -CH2CH3), 2.35 (s, 3H, CH3)3.02 (q, 2H, J 7.4 Hz, -CH2CH3), 7.28 (d, 2H, J 8.5 Hz, ArH), 7.31 (d, 2H, J 8.5 Hz, ArH), 8.90 (s, 1H, CH); δC 100 MHz (DMSO-d6, Me4Si) 157.08, 143.10, 141.50, 137.60, 130.60, 123.54, 122.50, 112.77, 109.72, 109.31, 21.14, 20.88, 11.14; νmax: (KBr) 3429, 2943, 2230, 1656, 1513, 1410, 1304, 1217, 938 cm−1.
General procedure for the reaction of 6-cyanopurines 2a,b and d,e with hydroxylamine hydrochloride
6-Cyanopurines 2a,b and d,e (1 equiv.), were dissolved/suspended in EtOH or CH2Cl2 (3-5 mL). While stirring, hydroxylamine hydrochloride (5 equiv.) and sodium acetate (4 equiv.) were added. The suspension was then stirred at rt, and the stirring was continued for 2-20 h, the precipitates were filtered off, and recrystallized from hot (EtOH:water) (1:1)/EtOH.
9-(4-Methoxyphenyl)-9H-purine-6-carboximidamide 3a: colourless crystals (0.67 g, 2.40 mmol, 60%); mp 230-232 oC [Anal. Calcd for C13H12N6O2: C, 54.92; H, 4.220; N; 29.57. Found: C, 55.22; H, 4.39; N, 29.81; m/z (EI) M+ 284, 60%, 254, 100%, M 284]; δH 400 MHz (DMSO-d6, Me4Si) 3.85 (s, 3H, OCH3), 6.15 (s, 2H, NH2), 7.17 (d, 2H, J 8.4 Hz, ArH), 7.78 (d, 2H, J 8.4 Hz, ArH), 8.92 (s, 1H, CH), 8.96 (s, 1H, CH), 10.64 (s, 1H, OH); δC 100 MHz (DMSO-d6, Me4Si) 159.98, 153.10, 152.68, 149.71, 148.93, 147.08, 131.05, 128.07, 126.54, 115.72, 56.60; νmax: (KBr) 3417, 3315, 3132, 2832, 1635, 1584, 1517, 1464, 1327, 1247, 1171, 1111, 1021, 776 cm−1.
9-(4-Methylphenyl)-9H-purine-6-carboximidamide 3b: light brown crystals (0.92 g, 3.64 mmol, 82%); mp 239-240 oC [Anal. Calcd for C13H12N6O: C, 58.20; H, 4.47; N; 31.34. Found: C, 58.24; H, 4.54; N, 31.08; m/z (EI) M+ 268, 30%, 238, 100%, M 268]; δH 400 MHz (DMSO-d6, Me4Si) 2.41 (s, 3H, CH3), 6.16 (s, 2H, NH2), 7.42 (d, 2H, J 8 Hz, ArH), 7.77 (d, 2H, J 8 Hz, ArH), 8.97 (s, 1H, CH), 8.98 (s, 1H, CH), 10.5 (s, 1H, OH); δC 100 MHz (DMSO-d6, Me4Si) 152.95, 152.74, 149.71, 148.95, 146.87, 138.84, 132.75, 131.16, 131.03, 124.66, 21.69; νmax: (KBr) 3440, 3335, 3161, 1630, 1584, 1519, 1461, 1380, 1327, 1215, 1106, 928, 823, 771 cm−1.
6-Methyl-9-(4-methylphenyl)-9H-purine-6-carboximidamide 3d: white powder (0.69 g, 2.4 mmol, 61%); mp 263-266 oC [Found: accurate mass: 282.1223; m/z (EI) M+ 282, 30%, 250, 100%, C14H14N6O requires: 282.1223; M 282]; δH 400 MHz (DMSO-d6, Me4Si) 2.41 (s, 3H, CH3), 2.72 (s, 3H, CH3), 6.11 (s, 2H, NH2), 7.42 (d, 2H, J 8 Hz, ArH), 7.74 (d, 2H, J 8 Hz, ArH), 8.85 (s, 1H, CH), 10.58 (s, 1H, OH); δC 100 MHz (DMSO-d6, Me4Si) 161.39, 153.07, 149.14, 148.03, 145.70, 138.23, 132.25, 130.47, 128.53, 124.25, 26.17, 21.12; νmax: (KBr) 3482, 3371, 3270, 1649, 1580, 1520, 1396, 1334, 1231, 1205, 926, 817 cm−1.
6-Ethyl-9-(4-methylphenyl)-9H-purine-6-carboximidamide 3e: pale yellow crystals (0.9 g, 3.0 mmol, 80%); mp 235 oC [Found: accurate mass: 296.1380; m/z (EI) 262, 100%, C15H16N6O requires: 296.1380; M 296]; δH 400 MHz (DMSO-d6, Me4Si) 1.32 (t, 3H, J 7.6 Hz, CH2CH3), 2.40 (s, 3H, CH3), 2.96 (q, 2H, J 7.6 Hz, CH2CH3), 6.11 (s, 2H, NH2), 7.42 (d, 2H, J 8 Hz, ArH), 7.76 (d, 2H, J 8 Hz, ArH), 8.87 (s, 1H, CH), 10.60 (s, 1H, OH); δC 100 MHz (DMSO-d6, Me4Si) 164.92, 152.55, 148.71, 147.70, 145.23, 137.56, 131.96, 129.98, 128.32, 123.52, 31.88, 20.66, 12.91; νmax: (KBr) 3453, 3336, 1650, 1570, 1583, 1521, 1398, 1341, 1224, 1206, 938, 914, 815 cm−1.
General procedure for the synthesis of pyrimido[5,4-d]pyrimidine 5
A mixture of 6-cyanopurines 2a (1 equiv.), methylamine hydrochloride (5 equiv.), and sodium acetate (4 equiv.), in EtOH (15 mL) was refluxed for 3 h. The precipitate was filtered off, washed with water (5 x 3 mL) and with EtOH (3 x 3 mL).
N-(4-Methoxyphenyl)-N`-methylpyrimido[5,4-d]pyrimidine-4,8-diamine 5: cream crystals (0.67 g, 2.67 mmol, 60%); mp 185-187 oC [Anal. Calcd for C14H14N6O: C, 59.57; H, 4.96; N; 29.78. Found: C, 59.86; H, 5.11; N, 29.95; m/z (EI) (M)+ 282, 100%, M 282]; δH 400 MHz (DMSO-d6, Me4Si) 2.99 (d, 3H, J 4.84 Hz, NHCH3), 3.76 (s, 3H, OCH3), 6.94 (d, 2H, J 9 Hz, ArH), 7.86 (d, 2H, J 9 Hz, ArH), 8.43 (br.q, J 4.8 Hz, 1H, NH), 8.47 (s, 1H, CH), 8.48 (s, 1H, CH), 9.88 (s, 1H, NH); δC 100 MHz (DMSO-d6, Me4Si) 159.91, 157.26, 156.54, 155.59, 154.61, 132.78, 132.43, 132.06, 124.01, 114.50, 56.08, 28.31; νmax: (KBr) 3437, 3343, 3265, 1599, 1566, 1542, 1508, 1450, 1415, 1333, 1242, 1122, 1032, 821 cm−1.
General procedure for the synthesis of pyrimido[5,4-d]pyrimidines 10a,b
6-Cyanopurines 2a,c (1 equiv.) were dissolved in CH2Cl2 (3-5 mL). While stirring at rt, hydrazine monohydrate (3-5 equiv.) was added, and the mixture was stirred for 18-20 h. The formed precipitate was filtered off, and washed with CH2Cl2.
N8-(4-Methoxyphenyl)-4-imino-3,4-dihydropyrimido[5,4-d]pyrimidine-3,8-diamine 10a: greenish-yellow powder (0.78 g, 2.78 mmol, 70%); mp 150-152 oC (decomp.) [Anal. Calcd for C13H13N7O: C, 55.12; H, 4.59; N; 34.60. Found: C, 55.23; H, 4.27; N, 34.68; m/z (EI) (M-H)+ 282, 100%, M 283]; δH 400 MHz (DMSO-d6, Me4Si) 3.75 (s, 3H, OCH3), 5.80 (s, 2H, NH2), 6.92 (d, 2H, J 8.9 Hz, ArH), 7.77 (d, 2H, J 8.9 Hz, ArH), 8.18 (s, 1H, CH), 8.32 (brs, 1H, NH), 8.48 (s, 1H, NH), 9.60 (s, 1H, CH); δC 100 MHz (DMSO-d6, Me4Si) 157.64, 157.12, 156.69, 155.97, 149.06, 139.00, 132.58, 126.34, 124.24, 114.66, 56.25; νmax: (KBr) 3374, 3193, 1607, 1577, 1533, 1510, 1428, 1315, 1246, 1177, 1034, 813 cm−1.
N8-(4-Methylphenyl)-4-imino-3,4-dihydropyrimido[5,4-d]pyrimidine-3,8-diamine 10b: greenish-yellow powder (0.80 g, 3.02 mmol, 68%); mp 198-200 oC [Found: accurate mass; 267.1221; m/z (EI) (M+) 267, 70%, 236, 100%, C13H13N7 requires: 267.1234; M 267]; δH 400 MHz (DMSO-d6, Me4Si) 2.29 (s, 3H, CH3), 5.96 (s, 2H, NH2), 7.16 (d, 2H, J 8.2 Hz, ArH), 7.78 (d, 2H, J 8.2 Hz, ArH), 8.27 (s, 1H, CH), 8.56 (s, 1H, CH), 8.91 (br.s., 1H, NH), 9.73 (s, 1H, NH); δC 100 MHz (DMSO-d6, Me4Si) 157.59, 157.07, 156.39, 149.11, 138.04, 136.58, 134.38, 130.08, 127.06, 122.72, 21.50; νmax: (KBr) 3434, 3353, 3263, 1638, 1603, 1556, 1531, 1421, 1188, 1036, 816 cm−1.
General procedure for the synthesis of pyrimido[4,5-e][1,2,4]triazolo[1,5-c]pyrimidines 11a-f
Pyrimido[5,4-d]pyrimidines 10a,b (1 equiv.) were refluxed in excess of triethyl orthoesters and a mixture of DMF/dioxane (1:1) (3 mL) for 6-24 h. The mixture was filtered while hot and crystals started to form almost immediately and were filtered off.
N-(4-Methoxyphenyl)-N-pyrimido[4,5-e][1,2,4]triazolo[1,5-c]pyrimidin-7-ylamine 11a: greenish-yellow crystals (0.53 g, 1.83 mmol, 52%); mp 240-242 oC [accurate mass Found: 293.1030; m/z (EI) M+ 292, 100%, C14H11N7O requires: 293.1027; M 293]; δH 400 MHz (DMSO-d6, Me4Si) 3.78 (s, 3H, OCH3), 6.97 (d, 2H, J 8.8 Hz, ArH), 7.81 (d, 2H, J 8.8 Hz, ArH), 8.75 (s, 1H, CH), 8.86 (s, 1H, CH), 9.84 (s, 1H, CH), 10.28 (s, 1H, NH); δC 100 MHz (DMSO-d6, Me4Si) 158.23, 157.75, 157.09, 156.35, 150.35, 139.99, 139.05, 132.20, 125.81, 125.01, 114.71, 56.29; νmax: (KBr) 3245, 2928, 1602, 1551, 1509, 1458, 1419, 1315, 1259, 1212, 1020 831 cm−1.
N-(4-Methoxyphenyl)-N-(2-methylpyrimido[4,5-e][1,2,4]triazolo[1,5-c]pyrimidin-7-yl)amine 11b: light yellow crystals (0.54 g, 1.76 mmol, 50%); mp 258-260 oC [Anal. Calcd for C15H13N7O: C, 58.63; H, 4.23; N; 31.92. Found: C, 58.45; H, 4.33; N, 31.55; m/z (EI) M+ 307, 100%, M 307]; δH 400 MHz (DMSO-d6, Me4Si) 2.62 (s, 3H, CH3), 3.77 (s, 3H, OCH3), 6.96 (d, 2H, J 8.8 Hz, ArH), 7.80 (d, 2H, J 8.8 Hz, ArH), 8.71 (s, 1H, CH), 9.69 (s, 1H, CH), 10.18 (s, 1H, NH); δC 100 MHz (DMSO-d6, Me4Si) 165.91, 158.06, 157.67, 157.03, 150.75, 139.43, 138.35, 132.21, 125.79, 124.97, 114.68, 56.26, 15.32; νmax: (KBr) 3435, 3254, 1603, 1555, 1532, 1511, 1493, 1440, 1311, 1247, 1030, 829 cm−1.
N-(2-Ethylpyrimido[4,5-e][1,2,4]triazolo[1,5-c]pyrimidin-7-yl)-(4-methoxyphenyl) amine 11c: light yellow crystals (0.56 g, 1.76 mmol, 50%); mp 228-230 oC [Anal. Calcd for C16H15N7O: C, 59.81; H, 4.67; N; 30.52. Found: C, 59.60; H, 4.78; N, 30.49; m/z (EI) M+ 321, 100%, M 321]; δH 400 MHz (DMSO-d6, Me4Si) 1.38 (t, 3H, J 7.6 Hz, CH2CH3), 2.96 (d, 2H, J 7.6 Hz, CH2CH3), 3.77 (s, 3H, OCH3), 6.96 (d, 2H, J 9.0 Hz, ArH), 7.80 (d, 2H, J 9 Hz, ArH), 8.72 (s, 1H, CH), 9.72 (s, 1H, CH), 10.20 (s, 1H, NH); δC 100 MHz (DMSO-d6, Me4Si) 170.46, 158.05, 157.71, 157.09, 139.53, 138.34, 132.12, 125.78, 125.00, 114.74, 80.093, 56.27, 22.72, 13.12; νmax: (KBr) 3433, 3257, 1605, 1577, 1559, 1531, 1511, 1417, 1314, 1246, 1178, 1035, 826 cm−1.
N-(4-Methylphenyl)-N-pyrimido[4,5-e][1,2,4]triazolo[1,5-c]pyrimidin-7-ylamine 11d: light yellow crystals (0.70 g, 2.54 mmol, 68%); mp 262-264 oC [Found: accurate mass: 277.1071; m/z (EI) 276, 100%, M+ 277, 65%, C14H11N7 requires: 277.1078; M 277]; δH 400 MHz (DMSO-d6, Me4Si) 2.32 (s, 3H, CH3), 7.20 (d, 2H, J 8.3 Hz, ArH), 7.83 (d, 2H, J 8.3 Hz, ArH), 8.79 (s, 1H, CH), 8.87 (s, 1H, CH), 9.84 (s, 1H, CH), 10.25 (s, 1H, NH); δC 100 MHz (DMSO-d6, Me4Si) 158.15, 157.70, 156.36, 150.33, 140.08, 139.11, 136.74, 134.32, 129.97, 125.85, 123.20, 21.60; νmax: (KBr) 3436, 1598, 1548, 1527, 1418, 1368, 1264, 1241, 993, 818 cm−1.
N-(4-Methylphenyl)-N-(2-methylpyrimido[4,5-e][1,2,4]triazolo-[1,5-c]pyrimidin-7-yl)amine 11e: light yellow crystals (0.62 g, 2.13 mmol, 57%); mp 253-255 oC [Anal. Calcd for C15H13N7: C, 61.85; H, 4.46; N; 33.67; Found: C, 61.89; H, 4.51; N, 33.65; m/z (EI) M+ 291, 70%, 290, 100%, M 291]; δH 400 MHz (DMSO-d6, Me4Si) 2.31 (s, 3H, CH3), 3.37 (s, 3H, CH3), 7.19 (d, 2H, J 8.3 Hz, ArH), 7.82 (d, 2H, J 8.3 Hz, ArH), 8.76 (s, 1H, CH), 9.71 (s, 1H, CH), 10.21 (s, 1H, NH); δC 100 MHz (DMSO-d6, Me4Si) 165.94, 157.99, 157.64, 150.75, 139.52, 138.43, 136.78, 134.24, 129.95, 125.83, 123.16, 21.60, 15.33; νmax: (KBr) 3357, 1600, 1554, 1533, 1483, 1415, 1373, 1286, 1252, 1150, 1008, 824 cm−1.
N-(2-Ethylpyrimido[4,5-e][1,2,4]triazolo[1,5-c]pyrimidin-7-yl)-(4-methylphenyl)amine 11f: light yellow crystals (0.57 g, 1.87 mmol, 50%); mp 233-234 oC [Anal. Calcd for C16H15N7 requires: C, 62.95; H, 4.91; N; 32.13. Found: C, 62.87; H, 5.01; N, 31.70; m/z (EI) (M+1)+ 306, 100%, M 305]; δH 400 MHz (DMSO-d6, Me4Si) 1.38 (t, 3H, J 7.2 Hz, CH2CH3), 2.30 (s, 3H, CH3), 2.96 (q, 2H, J 7.2 Hz, CH2CH3), 7.17 (d, 2H, J 8 Hz, ArH), 7.81 (d, 2H, J 8 Hz, ArH), 8.74 (s, 1H, CH), 9.70 (s, 1H, CH), 10.13 (s, 1H, NH); δC 100 MHz (DMSO-d6, Me4Si) 170.44, 157.18, 157.66, 150.56, 139.71, 138.24, 136.72, 134.30, 129.95, 125.79, 123.14, 22.76, 21.59, 13.12; νmax: (KBr) 3435, 3356, 1601, 1554, 1532, 1483, 1415, 1305, 1260, 997, 822 cm−1.
ACKNOWLEDGMENTS
The financial support of Kuwait University received through research grant SC 02/05 and SAF facilities through projects GS 01/01 and GS 03/01 are gratefully acknowledged. Thanks are due to Professor W. Clegg at the University of Newcastle upon Tyne, UK, for providing X-ray crystallographic results.
References
1. J. H. Lister, in The Chemistry of Heterocyclic Compounds, vol. 24 (II); Fused Pyrimidines, Part II, Purines, ed. by E. C. Taylor and A. Weissberger, Wiley-Interscience, New York, 1971, pp. 380-382.
2. K. T. Potts, in Comprehensive Heterocyclic Chemistry, ed. by A. R. Katritzky and C. W. Rees, Pergamon Press, 1984, vol 5, pp. 548-549.
3. C. A. Ramsden, in Comprehensive Heterocyclic Chemistry II, ed. by A. R. Katritzky, C. W. Rees, and E. F. V. Scriven, Pergamon, 1996, vol 7, p. 414.
4. A. Al-Azmi, B. L. Booth, R. A. Carpenter, A. Carvalho, E. Marrelec, R. G. Pritchard, and F. J. R. P. Proença, J. Chem. Soc., Perkin Trans. 1, 2001, 2532. CrossRef
5. M. A. Carvalho, T. M. Esteves, M. F. Proença, and B. L. Booth, Org. Biorg. Chem., 2004, 2, 1019. CrossRef
6. a) M. A. Carvalho, S. Esperança, T. M. Esteves, and M. F. Proença, Eur. J. Org. Chem., 2007, 1324; CrossRef b) A. Yamane, M. Matsuda, and T. Ueda, Chem. Pharm. Bull., 1980, 28, 150; c) M. J. Alves, B. L. Booth, M. A. Carvalho, R. G. Pritchard, and Proença F. J. R. P., J. Heterocycl. Chem., 1997, 34, 913. CrossRef
7. T. Higashino, S. Yoshida, and E. Hayashi, Chem. Pharm. Bull., 1982, 30, 4521.
8. P. D. Cook and D. A. Berry, Eur. Pat. Appl. EP 257, 488/02 Mar, 1988 (Chem. Abstr., 1988, 109, 110861h).
9. P. C. Srivastava, G. Revankar, R. K. Robins, R. D. Marsden, and R. J. Rousseau, J. Med. Chem., 1981, 24, 393. CrossRef
10. H. M. Berman, R. J. Rousseau, R. W. Mancuso, G. P. Kreishman, and R. K. Robins, Tetrahedron Lett., 1973, 33, 3099. CrossRef
11. P. Narayanan and H. M. Berman, Carbohydr. Res., 1975, 44, 169. CrossRef
12. J. D. Westover, G. R. Revankar, R. K. Robins, R. D. Marsden, R. D. Ogden, J. R. North, R. W. Mancuso, R. J. Rousseau, and E. L. Stephen, J. Med. Chem., 1981, 24, 941. CrossRef
13. Y. S. Sanghvi, S. B. Larson, S. S. Matsumoto, L. D. Nord, D. F. Smee, R.C. Willis, T. L. Avery, R. K. Robins, and G. R. Revankar, J. Med. Chem., 1981, 28, 167.
14. M. Hocek, M. Masojidkova, A. Holy, A. Graciela, R. Snoeck, J. Balzarini, and E. DeClerq, Collect. Czech. Chem. Commun., 1996, 61, 1525. CrossRef
15. T. E. Mabry, C. D. Jones, T. S. Chou, J. M. Colacino, G. B. Grindey, J. F. Worzalla, and H. L. Pearce, Nucleosides Nucleotides, 1994, 13, 1125. CrossRef
16. G. B. Elion, G. H. Hitchings, and H. Van der Werff, J. Biol. Chem., 1951, 192, 505.
17. G. H. Hitchings and G. B. Elion, Ann. N.Y. Acad. Sci., 1954, 60, 195.
18. G. H. Hitchtings and G. B. Elion, 3eme Congréss International de Biochemie Rapports, 1955, August 1-6, 185.
19. J. L. Kelley, M. P. Krochmal, J. A. Linn, E. W. McLean, and F. E. Soroko, J. Med. Chem., 1988, 31, 606. CrossRef
20. J. L. Kelley, M. P. Krochmal, J. A. Linn, E. W. McLean, and F. E. Soroko, J. Med. Chem., 1988, 31, 1005. CrossRef
21. J. L. Kelley, D. C. Wilson, V. L. Styles, F. S. Soroko, and B. R. Cooper, J. Heterocycl. Chem., 1995, 32, 1417. CrossRef
22. K. G. Estep, K. A. Josef, E. R. Bacon, P. M. Carabateas, S. R. IV, G. M. Pilling, D. S. Krafte, W. A. Volberg, K. Dillon, N. Dugrenier, G. M. Briggs, P. C. Canniff, W. P. Gorczyca, G. P. Stankus, and A. M. Ezrin, J. Med. Chem., 1995, 38, 2582. CrossRef
23. W. Pendergast and W. R. Hall, J. Heterocycl. Chem., 1989, 26, 1863. CrossRef
24. A. J. Kirby, J. E. Davies, T. A. S. Brandão, P. F. da Silva, W. R. Rocha, and F. Nome, J. Am. Chem. Soc., 2006, 128, 12374. CrossRef
25. a) A. J. Kirby, M. F. Lima, D. da Silva, C. D. Roussev, and F. Nome, J. Am. Chem. Soc., 2006, 128, 16944; CrossRef b) A. J. Kirby, B. S. Souza, M. Medeiros, J. P. Priebe, A. M. Manfrdi, and F. Nome, Chem. Commun., 2008, 37, 4428. CrossRef
26. W. P. Jencks, J. Am. Chem. Soc., 1958, 80, 4581. CrossRef
27. W. P. Jencks and J. Carriuolo, J. Am. Chem. Soc., 1960, 82, 1778. CrossRef
28. a) J. B. Domingos, E. Longhinotti, C. A. Bunton, and F. Nome, J. Org. Chem., 2003, 68, 7051. CrossRef
29. b) J. B. Domingos, E. Longhinotti, T. A. S. Brandao, C. A. Bunton, L. S. Santos, M. N. Eberlin, and F. Nome, J. Org. Chem., 2004, 69, 6024. CrossRef
30. K. V. Luzyanin, V. Yu. Kukushkin, M. L. Kuznetsov, A. D. Ryabov, M. Galanski, M. Haukka, E. V. Tretyakov, V. I. Ovcharenko, M. N. Kopylovich, and A. J. L. Pombeiro, Inorganic Chemistry, 2006, 45, 2296. CrossRef
31. J. R. Bertino, Encyclopedia of Cancer, New York, Academic Press, 1997, 2, 760.
32. J. A. Montgomery, R. James, and G. S. McCaleb, J. Med. Chem., 1967, 10, 668. CrossRef
33. J. M. Valgus, I. Terish, and S. L. Shifflett, Guide to Cancer Chemotherapeutic Regimens, Pharm Pract News Special Edition, 2000, 71.
34. A. Al-Azmi, J. Chem. Res., 2005, 8, 530. CrossRef
35. M. J. Alves, B. L. Booth, M. A. Carvalho, R. G. Pritchard, and F. J. R. P. Proença, J. Heterocycl. Chem., 1997, 34, 739. CrossRef
36. Crystal data for compound 3a: (ref. CCDC 639470) can be obtained on request from the Director, Cambridge Crystallographic Data Centre, 12 Union Road, Cambridge CB2 1EW, UK. CCDC.
37. Crystal data for compound 7d: (ref. CCDC 675997) can be obtained on request from the Director, Cambridge Crystallographic Data Centre, 12 Union Road, Cambridge CB2 1EW, UK. CCDC.
38. A. Al-Azmi, A. Elassar, and B. L. Booth, Tetrahedron, 2003, 59, 2749 CrossRef