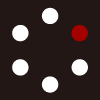
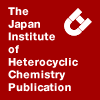
HETEROCYCLES
An International Journal for Reviews and Communications in Heterocyclic ChemistryWeb Edition ISSN: 1881-0942
Published online by The Japan Institute of Heterocyclic Chemistry
e-Journal
Full Text HTML
Received, 31st July, 2009, Accepted, 4th September, 2009, Published online, 9th September, 2009.
DOI: 10.3987/COM-09-S(S)96
■ Reaction Behavior of Cumulene: Diels–Alder, Friedel–Crafts, and Pd-Catalyzed Domino Reactions
Tomohiro Asakawa, Mie Iinuma, Yuko Wakasugi, Mayumi Kuno, Takumi Furuta,* Satoshi Fujii, Kiyoshi Tanaka, and Toshiyuki Kan*
Laboratory of Synthetic Organic & Medicinal Chemistry, Schol of Pharmaceutical Sciences, University of Shizuoka, 52-1 Yada, Shizuoka 422-8526, Japan
Abstract
Diels–Alder, Friedel–Crafts, and domino reactions mediated by Pd-catalyst of [3]cumulene 1 were investigated. Although the Diels–Alder reaction with cyclopentadiene occurred selectively on the C2, C3 double bond to give tetrasubstituted allenyl products, the Friedel–Crafts type addition of electron-rich heteroaromatics and subsequent protonation cleanly occurred on the C3, C4 double bond to afford tetrasubstituted conjugated dienes. In the Heck reaction with aryl iodide, the arylpalladium species was selectively inserted into the C3, C4 double bond and a subsequent C–H activation reaction with a neighboring phenyl group gave indene derivatives with a tetrasubstituted olefin moiety. Furthermore, Pd-catalyzed domino cyclization–allylation reaction of in situ generated [3]cumulene alkoxide afforded tetrasubstituted furan.INTRODUCTION
Cumulene derivatives have attracted much attention due to their characteristic structures, which are composed of linearly extended cumulated polyene systems, as well as their unique physiological properties.1 Cumulenes are found as partial structures in natural products; one prominent example is the neocarzinostatin chromophore where a biradical species generated by a Bergman type cycloaromatization of the eneyne[3]cumulene plays a key role in its potent anti-tumor activity.2
Although the reactivity of allene,3 which is the structurally simplest cumulene related molecule, has been thoroughly investigated, the reactivities of cumulene derivatives, such as [3]cumulenes, have not been well explored like as the case of allene. Thus, the reaction behavior of cumulenes toward reagents, including nucleophiles, electrophiles, and enophiles, is not well understood, although the cumulene π-system should possess remarkable reactivity. Furthermore, only few reports on the reactivity of cumulenes have investigated unsymmetrically substituted derivatives.4 Therefore, further surveys on the reaction behavior of unsymmetrically substituted cumulenes are needed.5
The tetrasubstituted alkene moiety, which could be readily derived from cumulated polyene, has promising bioactivities.6 As shown in Figure 1, tamoxifen and raloxifene, which are representative compounds, are promising anti-cancer drugs.7 Thus, the development of a stereoselective synthesis of tetrasubstituted alkene derivatives from cumulated polyenes is also required in medicinal chemistry.6
Although allenes have been utilized as valuable substrates to prepare tetrasubstituted alkene derivatives,6 similar cumulene derivatives may be superior due to the presence of additional and convertible double bonds. For these reason, we have surveyed the reaction behavior of an unsymmetrically substituted [3]cumulene derivative. Herein, we describe details of the Diels–Alder (DA), Friedel–Crafts, and Heck reactions of 2-methyl-5,5’-diphenyl-penta-2,3,4-trienal (1),8 which yields allene 2, diene 3,9 and indene 4,10 respectively, and all include a tetrasubstituted allene or an alkene structure. Furthermore, we wish to present a Pd(II)-catalyzed domino cyclization–allylation reaction to afford tetrasubstituted furan 5 (Figure 2).
RESULTS AND DISCUSSION
Preparation of [3]cumulenes: 1 is a suitable [3]cumulene substrate due to its stability and three distinct double bonds, which possess different electronic and steric properties due to the electron-withdrawing nature of the formyl group and steric bulkiness of the diphenyl moiety. Cumulene 1 was prepared according to the literature.8 Lithiation of 2-methyl-1-butene-3-yne (6) with n-BuLi and the subsequent addition of benzophenone (7) afforded the alkynyl alcohol. Without further purification, oxidation of the double bond with dimethyldioxirane gave 9 in a quantitative yield. Treatment of 9 with BF3·Et2O afforded desired cumulene derivative 1 (Scheme 1). This procedure is applicable to the gram-scale synthesis of 1. [3]Cumulene 11 was prepared from 4,4´-dimethylbenzophenone (8) in a similar manner as 1.
Diels–Alder Reaction: We initially tested the DA reaction of 1 with cyclopentadiene as depicted in Table 1. The DA reaction while heating at 150 °C in toluene proceeded at the C2, C3 double bond of 1 to give tetrasubstituted allenyl product 2 with exo selectively (entry 1).
This exo selectivity might originate from the unfavorable endo transition state due to the steric repulsion between the methyl substituent of 1 and the methylene moiety of cyclopentadiene. A similar exo selectivity has been observed in the DA reaction with methacrolein.11
To increase both the reactivity and selectivity, Lewis acid catalysts were screened. An equivalent amount of Yb(OTf)3 improved the yield of 2 in CH2Cl2 (entry 2). However, a unique solvent effect was observed in SnCl4 catalyzed reactions; although SnCl4 in CH2Cl2 was ineffective (entry 3), the reaction in THF gave the best yield (entry 4). Other Lewis acids, including AlCl3, Me3Al, and TMSOTf, also facilitated the DA reaction, but in diminished yields (entries 5–7). Table 1 shows the structures of the endo- and exo-isomers as determined by NOE experiments.
The reactivity of 1 was further investigated with Danishefsky’s diene.12 The DA reaction proceeded smoothly on the C2, C3 double bond to afford unstable allenyl cycloadduct 12, but further treatment with TBAF afforded trisubstituted benzene derivative 15 as the major product. This unexpected aromatization might occur via isomerization of allene 13 to diene 14 and successive deformylation of 14 by nucleophilic attack of the hydroxide ion (Scheme 2). None of the DA reactions gave products on the C3, C4 or C4, C5 double bonds. Hence, cumulene 1 has a sufficient reactivity toward DA reactions, and is an excellent dienophile with a reactive C2, C3 double bond due to the presence of the electron-withdrawing formyl group.
Friedel–Crafts type reaction: These promising results inspired us to examine the reactivity of 1 with electron-rich heteroaromatics. Although the reaction conditions were similar to the DA reactions, the Friedel–Crafts reaction proceeded smoothly by treating 1 and furan with 50 mol% of Yb(OTf)3 to give corresponding tetrasubstituted conjugated dienes 3 as an E/Z mixture in 93% yield (Scheme 3).13 Similar observations for the different reactivities of dimethylallene-1,3-dicarboxylate in DA and Friedel-Crafts reactions have been reported.14 Allene derivative 17, which could be generated by the protonation at C2 of enolate 16 (path b), was not detected, clearly demonstrating that the conjugate addition of the aryl group and subsequent protonation of enolate 16 selectively occur at C3 and C4, respectively (path a).
The Friedel–Crafts reaction with nitrogen containing heteroaromatics, including pyrrole and indoles, was also investigated (Table 2). In all cases, the reactions proceeded smoothly to give corresponding dienes 1813 and 19–22 in excellent yields. Moreover, neither the DA product nor the corresponding allene derivative was detected. With the indole derivatives, introducing methyl substituents at the 1 and 2 positions on the indole ring improved the Z-selectivity of the product (entries 4 and 5).
X-Ray analysis established the structure of compound 22 (Figure 3). Because the chemical shift of the Z-isomer aldehyde proton is at a higher-field than the corresponding E-isomer, the major isomers of 19–21 should be the Z-isomer due to anisotropic shielding of the neighboring indole ring.
These Friedel–Crafts type conjugate addition–protonations are formal 3, 4-additions to form tetrasubstituted conjugated dienes, which sharply contrasts the DA reaction because the DA reaction occurred at the C2, C3 positions of 1 to give tetrasubstituted allene derivatives.
Domino Heck–C−H activation reaction: Next, we explored the Heck reaction of 1, and initially expected a reactivity similar to the DA reaction would provide allene 24 via insertion of the arylpalladium species into the carbonyl conjugated C2, C3 double bond15 (path a) and successive β-hydride elimination of 23. However, the reaction afforded 2,3-diaryl indene derivative 26.16 This product can be explained by a reaction that proceeds through a domino process, and includes selective insertion of an arylpalladium species at the C3, C4 double bond (path b) and a subsequent C−H activation reaction17 with a neighboring phenyl moiety to afford palladacycle 25 (Scheme 4).
Next, the appropriate conditions for the Heck reaction with various aryl halides were surveyed (Table 3).
Upon treating 1 and phenyl iodide with 10 mol% Pd(OAc)2 and i-Pr2NEt, the reaction proceeded smoothly to afford indene derivative 4 as an E/Z mixture in good yield (entry 1). Using 2.0 equivalents of phenyl iodide improved the chemical yield (entry 2), but adding more phenyl iodide did not remarkably improve the yield (entry 3). Moreover, functionalized phenyl iodides were applied to this reaction (entries 5–11). The reaction with p-methoxyphenyl iodide gave corresponding indene derivative 27 in 46% yield (entry 5). In addition, aryl iodides with electron-withdrawing groups were compatible. Although p-mesyloxyphenyl iodide gave a moderate result (entry 6), a nitro substituent increased the yield to 66% (entry 7). Because phenyl bromide did not react (entry 4), the transformation with p-bromophenyl iodide afforded 30 without bromo substituent loss (entry 8). Aryl iodides with both electron-releasing and withdrawing groups at the meta-position also yielded the corresponding products in good yields (entries 9–11).
Because the aromatic region in the NMR spectra of products 4, 27–33 was highly overlapped, structural confirmations and stereochemistry determinations of the tetrasubstituted double bond were problematic. Therefore, the structures of the domino products were determined using the reaction with cumulene 11, an appropriate substrate to produce simple NMR signals. The domino reaction of 11 with p-nitrophenyl iodide proceeded smoothly to give an E/Z mixture of 34, which has much simpler signals in the aromatic region than 4 due to both the methyl and nitro substituents on the phenyl rings.
Therefore, each signal could be assigned. The NOE correlations between the aromatic (Ha) and the methyl protons, and the aromatic (Hb) and the formyl proton clearly indicated that the Z-isomer on the tetrasubstituted olefin was the major product. On the other hand, the NOEs between (Hc) and formyl proton, (Hd) and methyl protons were observed for the E-isomer (Scheme 5). Moreover, X-ray crystallographic analysis of 34, which included the stereochemistry, was unambiguously determined as shown in Figure 4. Because anisotropic shielding of the neighboring p-nitrophenyl moiety caused the Z-isomer aldehyde proton of 34 to shift to a higher-field compared to the corresponding E-isomer, the major isomer of 4, 27-33 should be the Z-isomer.
Moreover, we investigated the Heck reaction with nitrogen and sulfur containing heteroaromatics, such as indole and thiophene (Table 4). In addition to an excellent yield, N-non-protected 5-iodoindole improved the Z-selectivity of the product (entry 1). Employing N-methyl and N-tosylindoles in this reaction gave 36 and 37, respectively (entries 2 and 3), but using N-Boc indole, the product decomposed (entry 4). 2-Iodothiophene was also applicable to the domino reaction to afford 38 in excellent yield (entry 5).
Scheme 6 depicts a plausible mechanism for the domino Heck–C−H activation reaction. The first crucial step of the Heck reaction is insertion of arylpalladium iodide into the C3, C4 double bond to give intermediate 39.18 Because β-hydride elimination of 39 is not accessible, C−H activation of the neighboring phenyl moiety proceeds smoothly to afford six-membered palladacycle 25, which is successively cyclized to give indene derivative 26 via reductive elimination of the palladium intermediate. The addition of arylpalladium iodide to 1 is regioselective so that palladium(II) and the aryl group are positioned at C3 and C4, respectively, in 39. 16a,19
Multiple aryl modified tetrasubstituted alkenes have attracted attention as key structural elements for bioactive compounds, including tamoxifen,7 which is a clinically used drug for breast cancer. The Heck–C–H activation reaction of unsymmetrically substituted cumulenes described herein may be useful and valuable for constructing such bioactive tetrasubstituted alkenes with the appropriate functional groups on both the aromatic and alkene moieties.
These bioactive tetrasubstituted alkenes may be key substituents for interactions with receptors as well as pivotal in further chemical transformations. Moreover, products 4, 26–38 can be regarded as conformationally locked analogs of tamoxifen where one of the aromatic rings is fixed as the partial structure of the indene framework. Using these indene derivatives as bioprobes for tamoxifen related nuclear receptors are also interesting.
Domino cyclization–allylation reaction: Furthermore, we investigated Pd-catalyzed domino cyclization and allylation of 1 via nucleophilic addiditon of Grignard reagent (Table 5). After addition of MeMgBr to 1, Pd(OAc)2 (5 mol%) and allyl bromide were successively introduced to the reaction mixture to afford tetrasubstituted furan 5 in 38% yield (entry 1). The use of 10 mol% of Pd(OAc)2 increased the yield of 5 up to 42% (entry 2). Although employment with PdCl2 was also effective, the yield was diminished (entry 3).
On the other hand, Pd(PPh3)4 was ineffective for the reaction (entry 4). Therefore, the cyclization reaction would proceed with Pd(II) catalyst from the corresponding in situ generated cumulene alkoxide 40 by MeMgBr addition.20 Intramolecular oxypalladation at the C3, C4 double bond of 40 would afford cyclized intermediate 41, and successive allylation reaction and dehalopalladation to give 42, which could readily aromatize to 5 (Scheme 7).21
Highly substituted furans have attracted attention for their occurrence in a variety of bioactive natural products and pharmaceuticals.22 The Grignard reagent addiditon–Pd(II)-catalyzed cyclization–allylation sequence from 1 would be one of the most promissing and covenient way to access tetrasubstituted furans.23
In conclusion, we have found characteristic Diels–Alder and Friedel–Crafts type conjugate addition reactions of 1, which have concerted and stepwise reaction pathways. Furthermore, both reactions provide novel and useful routes to highly substituted allene and diene derivatives. Moreover, we have found unique Pd-catalyzed domino Heck–C–H activation reaction and cyclization–allylation reaction, which provides indene derivatives with tetrasubstituted alkene moieties and tetrasubstituted furan, respectively. Thus, a detailed mechanistic study and further chemical transformations of the reaction products to bioactive tetrasubstituted olefins such as tamoxifen related molecules as well as tetrasubstituted furan derivatives are currently under investigation.
EXPERIMENTAL
1H NMR spectra were obtained on JEOL ECA-500 at 500 MHz or JEOL EX-270 at 270 MHz with chemical shifts reported as ppm from tetramethylsilane as an internal standard. 13C NMR spectra were measured on JEOL ECA-500 at 125 MHz or JEOL EX-270 at 68 MHz. The mass spectra were measured on a JEOL MStation JMS-700 spectrometer. IR spectra were recorded on a JASCO WS/IR-8000. Unless otherwise noted, all reactions were carried out under an argon atmosphere. Column chromatography was performed using silica gel 60N spherical (63-210 mesh, KANTO CHEMICAL). Silica Gel 60 F254 plates (Merck) were used for preparative TLC (prep. TLC).
3-(2-Methyloxiran-2-yl)-1,1-di-p-tolylprop-2-yn-1-ol (10).
n-BuLi (2.5 mL, 4.0 mmol) was added to a solution of 2-methyl-1-butene-3-yne (0.38 mL, 4.0 mmol) in Et2O (2 mL) under an argon atmosphere at –50 °C, and the mixture was stirred for 30 min. 4,4’-Dimethylbenzophenone (8) (840 mg, 4.0 mmol) in THF (5 mL) was added, and the mixture was stirred and warmed to room temperature for 24 h. Then the mixture was washed with satd. aq. NH4Cl and brine, and extracted with Et2O. The organic layer was dried over anhydrous MgSO4, filtered, and evaporated under reduced pressure. The residue was dissolved in a DMDO acetone solution, and stirred at room temperature for 1 h. The reaction mixture was evaporated, and CH2Cl2 was added. The residue was purified by column chromatography (n-hexane:AcOEt = 4:1) to afford 10 (866 mg, 74%, 2 steps) as a colorless oil.
10: 1H NMR (270 MHz, CDCl3): δ 1.59 (s, 3H), 2.31 (s, 6H), 2.77 (d, 1H, J = 5.9 Hz), 2.81 (s, 1H), 3.05 (d, 1H, J = 5.9 Hz), 7.11 (d, 4H, J = 8.4 Hz), 7.42 (d, 4H, J = 8.4 Hz); 13C NMR (68 MHz, CDCl3): δ 21.0, 22.8, 47.3, 55.3, 73.9, 84.9, 86.0, 125.8, 128.9, 137.4, 141.8; IR (film) 1913, 2210, 3400 cm-1; FAB (m/z) 293 (M+H)+; HRMS (FAB) calcd for C20H21O2 293.1541 (M+H)+, found 293.1527.
2-Methyl-5,5-di-p-tolylpenta-2,3,4-trienal (11).
To a solution of 10 (245 mg, 0.84 mmol) in THF (7 mL), BF3•OEt2 (22 mL, 0.17 mmol) was added under an argon atmosphere at –78 °C. The mixture was stirred and warmed to room temperature for 3 h. The mixture was washed with brine, and extracted with Et2O. The organic layer was dried over anhydrous MgSO4, filtered, and evaporated under reduced pressure. The residue was purified by column chromatography (n-hexane:AcOEt = 9:1) to afford 11 (134 mg, 58%) as a yellow solid.
11: 1H NMR (500 MHz, CDCl3): δ 2.08 (s, 3H), 2.42 (s, 6H), 7.24 (d, 4H, J = 8.6 Hz), 7.48 (dd, 2H J = 8.6 Hz), 7.50 (dd, 2H, J = 8.6 Hz), 9.62 (s, 1H); 13C NMR (125 MHz, CDCl3): δ 14.3, 21.4, 115.5, 128.9, 129.35, 129.37, 129.6, 129.7, 130.2, 133.1, 134.8, 140.1, 140.2, 149.9, 169.9, 189.5; IR (film, cm-1) 1714, 1733, 2045 cm-1; FAB (m/z) 275 (M+H)+; HRMS (FAB) calcd for C20H19O 275.1436 (M+H)+, found 275.1460.
General Procedure for Diels–Alder Reaction of 1.
To a solution of 1 in a solvent, cyclopentadiene and a Lewis acid were added under an argon atmosphere, and the mixture was stirred. The mixture was added satd. aq. NaHCO3, and extracted with Et2O. The organic layer was washed with brine, dried over anhydrous MgSO4, filtered and evaporated under reduced pressure to give a residue. The residue was purified by column chromatography (n-hexane:AcOEt = 20:1) to afford 2 as a mixture of two isomers. The mixture was further purified by column chromatography (n-hexane:AcOEt = 30:1) to afford 2-exo and 2-endo isomers.
2-exo: 1H NMR (500 MHz, CDCl3): δ 1.20 (s, 3H), 1.73 (d, 1H, J = 9.1 Hz, 1H), 1.90 (d, 1H, J = 9.1 Hz), 3.17 (s, 1H), 3.55 (s, 1H), 6.30–6.35 (m, 1H), 6.45–6.50 (m, 1H), 7.20–7.40 (m, 10H), 9.76 (s, 1H); 13C NMR (125 MHz, CDCl3): δ 20.5, 48.6, 48.8, 50.4, 60.5, 109.2, 114.1, 127.3, 127.4, 128.2, 128.4, 128.5, 128.6, 136.0, 137.1, 137.2, 137.7, 199.2, 202.8; IR (film) 1719, 3016 cm-1; FAB (m/z) 313 (M+H)+; HRMS Calcd for C23H21O (M+H)+ 313.1592, found 313.1597.
2-endo: 1H NMR (500 MHz, CDCl3): δ 1.45 (s, 3H) 1.75 (d, 1H, J = 10 Hz, 1H), 2.00 (d, 1H, J = 10 Hz), 2.91 (s, 1H), 3.61 (s, 1H), 6.25–6.32 (m, 1H), 6.35–6.40 (m, 1H), 7.20-7.40 (m, 10H), 9.28 (s, 1H); 13C NMR (125 MHz, CDCl3): δ 21.0, 48.6, 50.2, 51.3, 60.7, 108.7, 114.2, 127.3, 127.4, 128.35, 128.42, 128.44, 134.8, 136.3, 137.2, 199.3, 203.6; IR (film) 1657, 3017 cm-1; FAB (m/z) 313 (M+H)+; HRMS Calcd C23H21O (M+H)+ 313.1592, found 313.1585.
3-(2,2-Diphenylvinyl)-4-methylphenol (15).
A solution of 1 (30 mg, 0.12 mmol) in trans-1-methoxy-3-(trimethylsilyloxy)-1,3-butadiene (0.23 mL, 1.2 mmol) was stirred at 130 °C for 1 h. Then the solution was evaporated. The residue was dissolved in THF (0.5 mL) and added to TBAF in THF (1 M) (0.24 mL, 0.24 mmol). The mixture was stirred at room temperature for 24 h, treated with satd. aq. NH4Cl, and extracted with Et2O. The organic layer was dried over anhydrous MgSO4, filtered, and evaporated under reduced pressure to give a residue, which was purified by column chromatography (n-hexane:Et2O = 2:1) to afford 9 (20 mg, 58%) as a dark yellow oil.
15: 1H NMR (270 MHz, CDCl3): δ 2.24 (s, 3H), 6.28 (d, 1H, J = 2.7 Hz), 6.55 (dd, 1H, J = 2.7, 7.8 Hz), 6.91 (s, 1H), 6.99 (d, 1H, J = 7.8 Hz), 7.04-7.40 (m, 10H); 13C NMR (68 MHz, CDCl3): δ 19.3, 113.9, 116.2, 126.8, 127.3, 127.6, 128.1, 128.2, 129.3, 130.6, 130.8, 137.9, 140.0, 143.4, 152.9; IR (film) 3362 cm-1; FAB (m/z) 287 (M+H)+; HRMS Calcd for C21H19O (M+H)+ 287.1436, found 287.1390.
3-(Furan-2-yl)-2-methyl-5,5-diphenylpenta-2,4-dienal (3).
To a solution of 1 (25 mg, 0.10 mmol) in CH2Cl2 (1 mL), Yb(OTf)3 (31 mg, 0.05 mmol) and furan (136 mg, 2.0 mmol) were added under an argon atmosphere at 0 °C. The mixture was stirred at room temperature for 24 h, washed with H2O and brine, extracted with CH2Cl2, dried over anhydrous MgSO4, and evaporated under reduced pressure. The residue was purified by column chromatography (n-hexane:AcOEt = 6:1) to afford 3 (29 mg, 92%, major isomer : minor isomer = 2.0 : 1.0) as a mixture of two isomers.
3 (E/Z mixture): 1H NMR (500 MHz, CDCl3): δ 1.87 (minor isomer), 1.94 (major isomer) (s, 3H), 6.10–7.60 (m, 14H), 9.86 (minor isomer), 10.05 (major isomer) (s, 1H); 13C NMR (125 MHz, CDCl3): δ 12.3, 13.2, 111.5, 112.1, 115.8, 116.1, 121.9, 125.1, 127.9, 127.9, 128.3, 128.4, 128.5, 128.6, 128.6, 128.7, 129.7, 129.7, 131.9, 135.5, 138.9, 139.4, 141.7, 141.9, 142.3, 142.6, 144.0, 144.7, 149.8, 150.3, 150.5, 152.9, 193.2, 193.8; IR (film, cm-1) 1630, 1659, 1692 cm-1; FAB (m/z) 315 (M+H)+; HRMS (FAB) calcd for C22H19O2 315.1385 (M+H)+, Found 315.1357.
2-Methyl-5,5-diphenyl-3-(1H-pyrrol-2-yl)penta-2,4-dienal (18).
To a solution of 1 (25 mg, 0.10 mmol) in CH2Cl2 (1 mL) were added Yb(OTf)3 (31 mg, 0.05 mmol) and pyrrole (134 mg, 2.0 mmol) under an argon atmosphere at 0 °C, and the mixture was stirred at room temperature for 24 h. The mixture was washed with H2O and brine, extracted with CH2Cl2, dried over anhydrous MgSO4, and evaporated under reduced pressure. The residue was purified by the column chromatography (n-hexane : AcOEt = 10 : 1) to afford 17 (22 mg, 70%, major isomer : minor isomer = 1.5 : 1.0) as a dark purple oil.
18 (E/Z mixture): 1H NMR (270 MHz, CDCl3): δ 1.82 (minor isomer), 1.92 (major isomer) (s, 3H), 6.00–7.40 (m, 14H), 8.47 (minor isomer), 8.76 (major isomer) (br s, 1H), 9.75 (minor isomer), 9.99 (major isomer) (s, 1H); 13C NMR (68 MHz, CDCl3): δ 12.7, 97.2, 110.0, 111.1, 115.4, 117.2, 120.8, 121.6, 122.5, 127.9, 128.0, 128.2, 128.2, 128.3, 128.3, 128.4, 128.5, 128.7, 129.4, 130.0, 132.4, 137.5, 138.6, 141.5, 155.4, 192.7; IR (film) 1630, 1645 cm-1; FAB (m/z) 314 (M+H)+; HRMS (FAB) calcd for C22H20NO 314.1545 (M)+, found 314.1519.
3-(1H-Indol-3-yl)-2-methyl-5,5-diphenylpenta-2,4-dienal (19).
To a solution of 1 (17 mg, 0.07 mmol) in CH2Cl2 (0.7 mL) were added Yb(OTf)3 (22 mg, 0.035 mmol) and indole (17 mg, 0.14 mmol) under an argon atmosphere at 0 °C, and the mixture was stirred at room temperature for 12 h. The mixture was washed with H2O and brine, extracted with CH2Cl2, dried over anhydrous MgSO4, and evaporated under reduced pressure. The residue was purified by the column chromatography (n-hexane : AcOEt = 4 : 1) to afford 19 (18 mg, 70%, E : Z = 1.0 : 3.0) as a dark yellow solid.
19 (E/Z mixture). 1H NMR (270 MHz, CDCl3) δ 1.81 (E-isomer), 1.96 (Z-isomer) (s, 3H), 6.70–7.70 (m, 15H), 8.41 (Z-isomer), 8.76 (E-isomer) (br s, 1H), 9.64 (Z-isomer), 10.13 (E-isomer) (s, 1H); 13C NMR (125 MHz, CDCl3) δ 13.1, 14.1, 111.2, 113.3, 113.5, 115.9, 120.1, 120.5, 120.7, 122.5, 127.05, 127.11, 127.2, 127.3, 127.4, 127.5, 127.9, 128.2, 128.28, 128.33, 128.4, 128.9, 129.6, 130.0, 134.8, 135.9, 139.3, 142.5, 149.2, 149.3, 149.9, 193.0, 194.2; FAB (m/z) 363 (M+H)+; HRMS (FAB) calcd for C26H21NO 363.1623 (M)+, found 363.1626.
2-Methyl-3-(1-methyl-1H-indol-3-yl)-5,5-diphenylpenta-2,4-dienal (20).
To a solution of 1 (49 mg, 0.20 mmol) in CH2Cl2 (2 mL) were added Yb(OTf)3 (62 mg, 0.10 mmol) and 1-methylindole (52 mg, 0.40 mmol) under an argon atmosphere at 0 °C, the mixture was stirred at room temperature for 24 h. The mixture was washed with H2O and brine, extracted with CH2Cl2, dried over MgSO4, and evaporated under reduced pressure. The residue was purified by the column chromatography (n-hexane : AcOEt = 7 : 1) to afford 20 (55 mg, 73%, E : Z = 1.0 : 2.3) as an orange yellow solid.
20 (E/Z mixture). 1H NMR (270 MHz, CDCl3) δ 1.82 (E-isomer), 1.96 (Z-isomer) (s, 3H), 3.54 (Z-isomer), 3.76 (E-isomer) (s, 3H), 6.60–7.80 (m, 16H), 9.67 (Z-isomer), 10.11 (E-isomer) (s, 1H); 13C NMR (68 MHz, CDCl3) δ 12.9, 14.1, 32.5, 33.0, 109.2, 109.6, 112.5, 120.2, 120.3, 121.3, 122.2, 126.77, 126.84, 127.2, 127.8, 127.9, 128.27, 128.34, 129.6, 130.0, 131.7, 133.5, 134.5, 136.9, 139.6, 142.5, 148.9, 149.7, 192.5, 194.1; IR (CHCl3) 1643 cm-1; FAB (m/z) 378 (M+H)+; HRMS (FAB) calcd for C27H24NO 378.1858 (M+H)+, found 378.1906.
2-Methyl-3-(2-methyl-1H-indol-3-yl)-5,5-diphenylpenta-2,4-dienal (21).
To a solution of 1 (49 mg, 0.20 mmol) in CH2Cl2 (2 mL) were added Yb(OTf)3 (62 mg, 0.10 mmol) and 2-methylindole (52 mg, 0.40 mmol) under an argon atmosphere at 0 °C, the mixture was stirred at room temperature for 10 h. The mixture was washed with H2O and brine, extracted with CH2Cl2, dried over MgSO4, and evaporated under reduced pressure. The residue was purified by the column chromatography (n-hexane : AcOEt = 7 : 1) to afford 21 (57 mg, 76%, E : Z = 1.0 : 4.0) as a orange yellow solid.
21 (E/Z mixture). Mp198–200 °C; 1H NMR (270 MHz, CDCl3) δ 1.71 (E-isomer), 2.03 (Z-isomer) (s, 3H), 2.04 (E-isomer), 2.09 (Z-isomer) (s, 3H), 6.50–7.80 (m, 16H), 9.45 (Z-isomer), 10.40 (E-isomer) (s, 1H); 13C NMR (68 MHz, CDCl3) δ 12.5, 12.9, 23.7, 38.7, 109.9, 110.6, 119.6, 120.1, 121.6, 126.6, 126.9, 127.2, 128.2, 128.3, 128.4, 128.8, 129.2, 135.0, 135.5, 139.5, 142.6, 148.6, 150.0, 183.3, 194.2; IR (CHCl3) 1643 cm-1; FAB (m/z) 378 (M+H)+; HRMS (FAB) calcd for C27H24NO 378.1858 (M+H)+, found 378.1864.
3-(1,2-Dimethyl-1H-indol-3-yl)-2-methyl-5,5-diphenylpenta-2,4-dienal (22).
To a solution of 1 (24 mg, 0.10 mmol) in CH2Cl2 (1 mL) were added Yb(OTf)3 (31 mg, 0.05 mmol) and 1,2-dimethylindole (14 mg, 0.10 mmol) under an argon atmosphere at 0 °C, the mixture was stirred at room temperature for 6 h. The mixture was washed with H2O and brine, extracted with CH2Cl2, dried over anhydrous MgSO4, and evaporated under reduced pressure. The residue was purified by the column chromatography (n-hexane : AcOEt = 9 : 1 to 5 : 1) to afford 22 (30 mg, 78%, E : Z = 1.0 : 8.0) as an orange yellow crystal.
22 (E/Z mixture). Mp 204–207 °C; 1H NMR (270 MHz, CDCl3) δ 1.72 (E-isomer), 1.96 (Z-isomer) (s, 3H), 1.99 (E-isomer), 2.11 (Z-isomer) (s, 3H), 3.30 (Z-isomer), 3.40 (E-isomer) (s, 3H), 6.40–7.70 (m, 15H), 9.44 (Z-isomer), 10.42 (E-isomer) (s, 1H); 13C NMR (68 MHz, CDCl3) δ 11.7, 12.3, 29.1, 108.3, 110.0, 119.5, 119.8, 121.1, 126.1, 126.3, 127.5, 128.1, 128.25, 128.30, 128.6, 135.2, 136.5, 137.5, 139.7, 142.6, 149.0, 149.8, 194.2; FAB (m/z) 392 (M+H)+; HRMS (FAB) calcd for C28H26NO 392.2014 (M+H)+, found 392.2010; Crystallographic data: C28H25NO, M = 391.51, triclinic, P-1 (#2), T = 298 K, a = 9.9399(10) Å, b = 11.8748(8) Å, c = 9.7921(6) Å, a = 95.791(5)°, b = 92.372(7)°, g = 108.820(6)°, V = 1085.07(15) Å3, Z = 2, Dc = 1.198 g/cm3, l (CuKa) = 1.54178 Å, R = 0.0456, wR2[F2] = 0.1798 for 865 unique reflections.
Typical procedure for domino Heck–C–H activation reaction (Table 3, entries 1–3).
To a solution of 1 (100 mg, 0.41 mmol) in MeCN (4 mL), iodobenzene (91 mL, 0.81 mmol), Pd(OAc)2 (9.2 mg, 0.041 mmol) and i-Pr2NEt (0.21 mL, 1.2 mmol) under an argon atmosphere, and the mixture was stirred at 80 °C for 1 h. The reaction mixture was evaporated, and CH2Cl2 was added. The mixture was washed with satd. aq. NH4Cl and brine, and extracted with CH2Cl2. The organic layer was dried over anhydrous MgSO4, filtered, and evaporated under reduced pressure to give a residue, which was purified by column chromatography (n-hexane : AcOEt = 9 : 1) to afford 4 (78 mg, 59%, E : Z = 1.0 : 3.6) as a red solid (Table 3, entry 2).
2-(2,3-Diphenyl-1H-inden-1-ylidene)propanal (4) (E/Z mixture). 1H NMR (270 MHz, CDCl3): δ 1.65 (E-isomer), 2.50 (Z-isomer) (s, 3H), 7.00–7.90 (m, 14H), 9.52 (Z-isomer), 10.9 (E-isomer) (s, 1H); 13C NMR (68 MHz, CDCl3): δ 14.1, 14.2, 121.2, 121.4, 126.2, 126.4, 126.6, 126.8, 127.3, 127.5, 127.6, 127.8, 128.0, 128.1, 128.2, 128.3, 128.5, 128.6, 128.9, 129.1, 129.2, 129.5, 129.95, 129.99, 130.1, 132.3, 133.6, 136.7, 136.8, 137.4, 137.8, 143.6, 145.9, 152.0, 192.5, 193.0; IR (film) 1659 cm-1; FAB (m/z) 323 (M+H)+; HRMS (FAB) calcd for C24H19O 323.1436 (M+H)+, found 323.1450.
2-[2-(4-Methoxyphenyl)-3-phenyl-1H-inden-1-ylidene]propanal (27).
To a solution of 1 (50 mg, 0.20 mmol) in MeCN (2 mL) were added 4-iodoanisole (95 mg, 0.41 mmol), Pd(OAc)2 (4.6 mg, 0.020 mmol) and i-Pr2NEt (0.11 mL, 0.63 mmol) under an argon atmosphere, and the mixture was stirred at 80 °C for 1 h. The reaction mixture was evaporated, and added CH2Cl2. The mixture was washed with satd. aq. NH4Cl and brine, extracted with CH2Cl2. The organic layer was dried over anhydrous MgSO4, filtered and evaporated under reduced pressure. The residue was purified by the column chromatography (n-hexane : CHCl3 = 9 : 1) to afford 27 (33 mg, 46%, E : Z = 1.0 : 3.6) as a red solid.
27 (E/Z mixture). 1H NMR (270 MHz, CDCl3): δ 1.68 (E-isomer), 2.49 (Z-isomer) (s, 3H), 3.78 (Z-isomer), 3.80 (E-isomer) (s, 3H), 6.70–7.90 (m, 13H), 9.57 (Z-isomer), 10.88 (E-isomer) (s, 1H); 13C NMR (68 MHz, CDCl3): δ 14.15, 14.18, 55.1, 113.8, 114.1, 121.1, 121.3, 121.9, 126.0, 126.38, 126.41, 126.8, 127.6, 127.7, 127.8, 128.0, 128.07, 128.13, 128.4, 128.9, 129.1, 129.2, 129.4, 129.8, 131.05, 131.12, 133.8, 136.6, 136.7, 137.4, 143.7, 145.4, 152.2, 158.9, 192.8, 193.2; IR (film) 1028, 1234, 1242, 1654 cm-1; FAB (m/z) 353 (M+H)+; HRMS (FAB) calcd for C25H21O2 353.1542 (M+H)+, found 353.1558.
4-[1-(1-Oxopropan-2-ylidene)-3-phenyl-1H-inden-2-yl]phenylmethanesulfonate (28). To a solution of 1 (50 mg, 0.20 mmol) in MeCN (2 mL) were added 4-iodophenyl methanesulfonate (121 mg, 0.41 mmol), Pd(OAc)2 (4.6 mg, 0.020 mmol) and i-Pr2NEt (0.11 mL, 0.63 mmol) under an argon atmosphere, and the mixture was stirred at 80 °C for 1 h. The reaction mixture was evaporated, and added CH2Cl2. The mixture was washed with satd. aq. NH4Cl and brine, extracted with CH2Cl2. The organic layer was dried over anhydrous MgSO4, filtered and evaporated under reduced pressure. The residue was purified by the column chromatography (n-hexane : AcOEt = 7 : 3) to afford 28 (32 mg, 39%, E : Z = 1.0 : 3.1) as a red solid.
28 (E/Z mixture). 1H NMR (270 MHz, CDCl3): δ 1.68 (E-isomer), 2.51 (Z-isomer) (s, 3H), 3.11 (Z-isomer), 3.17 (E-isomer) (s, 3H), 7.00–7.90 (m, 13H), 9.57 (s, 1H, Z-isomer), 10.88 (s, 1H, E-isomer); 13C NMR (68 MHz, CDCl3): δ 8.9, 14.2, 37.3, 38.0, 121.5, 121.6, 121.8, 122.1, 122.4, 126.5, 127.0, 127.1, 128.0, 128.2, 128.3, 128.6, 128.96, 129.02, 129.6, 131.6, 131.8, 133.2, 135.0, 136.6, 137.2, 137.4, 139.8, 140.0, 143.2, 147.0, 148.5, 151.8, 191.9, 192.8; IR (film) 1150, 1174, 1356, 1651 cm-1; FAB (m/z) 417 (M+H)+; HRMS (FAB) calcd for C25H21O4S 417.1161 (M+H)+, found 417.1165.
2-[2-(4-Nitrophenyl)-3-phenyl-1H-inden-1-ylidene]propanal (29).
To a solution of 1 (50 mg, 0.20 mmol) in MeCN (2 mL) were added 1-iodo-4-nitrobenzene (101 mg, 0.41 mmol), Pd(OAc)2 (4.6 mg, 0.020 mmol) and i-Pr2NEt (0.11 mL, 0.63 mmol) under an argon atmosphere, and the mixture was stirred at 80 °C for 1 h. The reaction mixture was evaporated, and added CH2Cl2. The mixture was washed with satd. aq. NH4Cl and brine, extracted with CH2Cl2. The organic layer was dried over anhydrous MgSO4, filtered and evaporated under reduced pressure. The residue was purified by the column chromatography (n-hexane : AcOEt = 9 : 1) to afford 29 (49 mg, 66%, E : Z = 1.0 : 4.3) as a red solid.
29 (E/Z mixture). 1H NMR (270 MHz, CDCl3): δ 1.67 (E-isomer), 2.52 (Z-isomer) (s, 3H), 7.00–8.50 (m, 13H), 9.52 (Z-isomer), 10.88 (E-isomer) (s, 1H); 13C NMR (68 MHz, CDCl3): δ 14.2, 15.2, 121.8, 121.9, 123.5, 123.8, 129.3, 126.9, 127.4, 128.3, 128.38, 128.42, 128.5, 128.8, 128.9, 129.2, 129.8, 130.8, 131.1, 132.8, 134.2, 136.6, 137.5, 143.0, 144.9, 145.0, 146.8, 148.1, 151.5, 191.4, 192.4; IR (film) 1340, 1508, 1595, 1658 cm-1; FAB (m/z) 368 (M+H)+; HRMS (FAB) calcd for C24H18NO3 368.1287 (M+H)+, found 368.1302.
2-[2-(4-Bromophenyl)-3-phenyl-1H-inden-1-ylidene]propanal (30).
To a solution of 1 (50 mg, 0.20 mmol) in MeCN (2 mL) were added 1-bromo-4-iodobenzene (115 mg, 0.41 mmol), Pd(OAc)2 (4.6 mg, 0.020 mmol) and i-Pr2NEt (0.11 mL, 0.63 mmol) under an argon atmosphere, and the mixture was stirred at 80 °C for 1 h. The reaction mixture was evaporated, and added CH2Cl2. The mixture was washed with satd. aq. NH4Cl and brine, extracted with CH2Cl2. The organic layer was dried over anhydrous MgSO4, filtered and evaporated under reduced pressure. The residue was purified by the column chromatography (n-hexane : AcOEt = 9 : 1) to afford 30 (44 mg, 55%, E : Z = 1.0 : 2.7) as a red solid.
30 (E/Z mixture). 1H NMR (270 MHz, CDCl3): δ 1.68 (E-isomer), 2.50 (Z-isomer) (s, 3H), 7.00–7.90 (m, 13H), 9.58 (Z-isomer), 10.87 (E-isomer) (s, 1H); 13C NMR (68 MHz, CDCl3): δ 14.2, 14.6, 121.4, 121.6, 121.8, 126.5, 126.9, 127.9, 128.25, 128.33, 129.0, 129.1, 129.6, 131.5, 131.8, 131.9, 133.3, 135.4, 136.67, 136.73, 137.4, 143.4, 151.8, 192.1, 192.9; IR (film) 771, 1660 cm-1; FAB (m/z) 400 (M)+; HRMS (FAB) calcd for C24H17BrO 400.0463 (M)+, found 400.0444.
2-[2-(3-Methoxyphenyl)-3-phenyl-1H-inden-1-ylidene]propanal (31).
To a solution of 1 (50 mg, 0.20 mmol) in MeCN (2 mL) were added 3-iodoanisole (95 mg, 0.41 mmol), Pd(OAc)2 (4.6 mg, 0.020 mmol) and i-Pr2NEt (0.11 mL, 0.63 mmol) under an argon atmosphere, and the mixture was stirred at 80 °C for 2 h. The reaction mixture was evaporated, and added CH2Cl2. The mixture was washed with satd. aq. NH4Cl and brine, extracted with CH2Cl2. The organic layer was dried over anhydrous MgSO4, filtered and evaporated under reduced pressure. The residue was purified by the column chromatography (n-hexane : AcOEt = 9 : 1) to afford 31 (33mg, 47%, E : Z = 1.0 : 2.3) as a red solid.
31 (E/Z mixture). 1H NMR (270 MHz, CDCl3): δ 1.70 (E-isomer), 2.49 (Z-isomer) (s, 3H), 3.66 (Z-isomer), 3.71 (E-isomer) (s, 3H), 6.50–7.90 (m, 13H), 9.55 (Z-isomer), 10.88 (E-isomer) (s, 1H); 13C NMR (68 MHz, CDCl3): δ 14.1, 14.2, 55.1, 55.3, 113.0, 113.3, 113.7, 115.4, 115.6, 121.3, 121.5, 122.6, 123.0, 126.2, 126.4, 126.6, 126.8, 127.7, 127.8, 128.08, 128.14, 128.2, 128.9, 129.0, 129.1, 129.4, 129.5, 129.7, 129.8, 130.0, 130.7, 133.7, 136.6, 136.7, 137.4, 139.0, 139.4, 143.5, 151.9, 159.6, 192.7, 193.1; IR (film) 1454, 1575, 1599, 1659 cm-1; FAB (m/z) 353 (M+H)+. HRMS (FAB) calcd for C25H21O2 353.1542 (M+H)+, found 353.1506.
Preparation of 3-iodophenyl methanesulfonate for Table 3 (entry 10).
To a solution of m-iodophenol (1.0 g, 4.5 mmol) in CH2Cl2 (25 mL) were added MsCl (0.70 mL, 9.0 mmol) and Et3N (1.9 mL, 14 mmol) under an argon atmosphere at 0 °C. The mixture was stirred at room temperature for 30 min. The mixture was washed with brine. The organic layer was dried over anhydrous MgSO4, filtered and evaporated under reduce pressure to give 3-iodophenyl methanesulfonate (1.3 g, 98%) as a white solid.
3-Iodophenyl methanesulfonate. 1H NMR (270 MHz, CDCl3): δ 3.17 (s, 3H), 7.15 (t, 1H, J = 8.0 Hz, 1H), 7.29 (br d, 1H, J = 8.0 Hz), 7.60–7.73 (m, 2H); 13C NMR (68 MHz, CDCl3): δ 37.6, 93.8, 121.5, 131.0, 131.2, 136.5, 149.1; IR (film) 1331, 1576, 3034 cm-1; FAB (m/z) 299 (M+H)+; HRMS (FAB) calcd for C7H8O3IS 298.9239 (M+H)+, found 298.9239.
3-[1-(1-Oxopropan-2-ylidene)-3-phenyl-1H-inden-2-yl]phenylmethanesulfonate (32). To a solution of 1 (50 mg, 0.20 mmol) in MeCN (2 mL) were added 3-iodophenyl methanesulfonate (121 mg, 0.41 mmol), Pd(OAc)2 (4.6 mg, 0.020 mmol) and i-Pr2NEt (0.11 mL, 0.63 mmol) under an argon atmosphere, and the mixture was stirred at 80 °C for 1 h. The reaction mixture was evaporated, and added CH2Cl2. The mixture was washed with satd. aq. NH4Cl and brine, extracted with CH2Cl2. The organic layer was dried over anhydrous MgSO4, filtered and evaporated under reduced pressure. The residue was purified by the column chromatography (CHCl3) to afford 32 (52mg, 62%, E : Z = 1.0 : 2.3) as a red solid.
32 (E/Z mixture). 1H NMR (270 MHz, CDCl3): δ 1.71 (E-isomer), 2.51 (Z-isomer) (s, 3H), 2.80 (Z-isomer), 2.86 (E-isomer) (s, 3H), 7.00–8.00 (m, 13H), 9.60 (Z-isomer), 10.88 (E-isomer) (s, 1H); 13C NMR (68 MHz, CDCl3): δ 14.2, 14.7, 36.7, 36.9, 121.1, 121.2, 121.5, 121.6, 123.1, 123.6, 126.5, 126.6, 126.9, 127.1, 127.9, 128.1, 128.3, 128.4, 129.0, 129.1, 129.3, 129.7, 129.9, 130.3, 133.3, 134.8, 136.5, 137.5, 140.0, 143.1, 147.2, 148.5, 149.2, 149.5, 151.4, 192.0, 192.7; IR (film) 829, 1338, 1655 cm-1; FAB (m/z) 417 (M+H)+; HRMS (FAB) calcd for C25H21O4S 417.1161 (M+H)+, found 417.1161.
2-[2-(3-Nitrophenyl)-3-phenyl-1H-inden-1-ylidene]propanal (33).
To a solution of 1 (50 mg, 0.20 mmol) in MeCN (2 mL) were added 1-iodo-3-nitrobenzene (101 mg, 0.41 mmol), Pd(OAc)2 (4.6 mg, 0.020 mmol) and i-Pr2NEt (0.11 mL, 0.63 mmol) under an argon atmosphere, and the mixture was stirred at 80 °C for 1 h. The reaction mixture was evaporated, and added CH2Cl2. The mixture was washed with satd. aq. NH4Cl and brine, extracted with CH2Cl2. The organic layer was dried over anhydrous MgSO4, filtered and evaporated under reduced pressure. The residue was purified by the column chromatography (n-hexane : AcOEt = 9 : 1) to afford 33 (35mg, 47%, E : Z = 1.0 : 2.5) as a red solid.
33 (E/Z mixture). 1H NMR (270 MHz, CDCl3): δ 1.66 (E-isomer), 2.53 (Z-isomer) (s, 3H), 7.10–8.20 (m, 13H), 9.57 (Z-isomer), 10.89 (E-isomer) (s, 1H); 13C NMR (68 MHz, CDCl3): δ 14.2, 15.0, 121.8, 121.9, 122.4, 124.7, 125.1, 126.6, 126.9, 127.0, 127.4, 128.2, 128.36, 128.43, 128.5, 128.9, 129.0, 129.2, 129.3, 129.6, 129.8, 132.7, 132.8, 133.9, 135.9, 136.3, 136.5, 137.5, 139.7, 143.0, 148.1, 148.3, 151.5, 191.4, 192.5; IR (film) 1232, 1346, 1528 1655 cm-1; FAB (m/z) 368 (M+H)+; HRMS (FAB) calcd for C24H18NO3 368.1287 (M+H)+, found 368.1301.
2-[6-Methyl-2-(4-nitrophenyl)-3-p-tolyl-1H-inden-1-ylidene]propanal (34).
To a solution of 10 (55 mg, 0.20 mmol) in MeCN (2 mL) were added 1-iodo-4-nitrobenzene (100 mg, 0.40 mmol), Pd(OAc)2 (4.5 mg, 0.020 mmol) and i-Pr2NEt (0.10 mL, 0.57 mmol) under an argon atmosphere, and the mixture was stirred at 80 °C for 1 h. The reaction mixture was evaporated, and added CH2Cl2. The mixture was washed with satd. aq. NH4Cl and brine, extracted with CH2Cl2. The organic layer was dried over anhydrous MgSO4, filtered and evaporated under reduced pressure. The residue was purified by the column chromatography (n-hexane : AcOEt = 9 : 1) to afford 34 (39 mg, 49%, E : Z = 1.0 : 2.4) as a red crystal.
34 (E/Z mixture). Mp 202–204 °C; 1H NMR (500 MHz, CDCl3): δ 1.65 (E-isomer), 2.50 (Z-isomer) (s, 3H), 2.32 (E-isomer), 2.33 (Z-isomer) (s, 3H), 2.42 (E-isomer), 2.47 (Z-isomer) (s, 3H), 6.98 (Z-isomer), 7.02 (E-isomer) (d, 2H, J = 7.9 Hz), 7.06–7.12 (E/Z isomer) (m, 2H), 7.13–7.19 (E/Z isomer) (m, 2H), 7.32 (Z-isomer, Hb), 7.35 (E-isomer, Hd) (d, 2H, J = 8.5 Hz), 7.43 (E-isomer, Hc), 7.72 (Z-isomer, Ha) (s, 1H), 8.11 (Z-isomer), 8.14 (E-isomer) (d, 2H, J = 8.5 Hz), 9.51 (Z-isomer), 10.86 (E-isomer) (s, 1H); 13C NMR (68 MHz, CDCl3): δ 14.2, 15.1, 21.3, 21.8, 21.9, 121.6, 121.8, 123.5, 123.8, 127.7, 128.2, 128.7, 128.9, 129.1, 129.2, 129.6, 129.8, 129.9, 130.2, 130.9, 131.2, 133.2, 135.0, 136.7, 136.9, 137.1, 137.4, 138.2, 138.4, 138.5, 140.3, 140.6, 144.9, 145.5, 146.7, 148.2, 151.9, 191.5, 192.7; FAB (m/z) 396 (M+H)+; HRMS (FAB) calcd for C26H22NO3 396.1599 (M+H)+, found 396.1592; Crystallographic data: C26H21NO3, M = 395.46, triclinic, P-1 (#2), T = 298 K, a = 10.7108(14) Å, b = 11.2338(13) Å, c = 10.2696(12) Å, α = 96.444(10)°, β = 110.963(9)°, γ = 110.197(9)°, V = 1043.0(2) Å3, Z = 2, Dc = 1.259 g/cm3, λ (CuKα) = 1.54178 Å, R = 0.1183, wR2[F2] = 0.3072 for 833 unique reflections. CCDC 609878.
2-[2-(1H-Indol-5-yl)-3-phenyl-inden-1-ylidene]-propionaldehyde (35).
To a solution of 1 (200 mg, 0.81 mmol) in MeCN (8 mL) were added 5-iodoindole (394 mg, 1.62 mmol), Pd(OAc)2 (18 mg, 0.080 mmol) and i-Pr2NEt (0.42 mL, 2.43 mmol) under an argon atmosphere, and the mixture was stirred at 80 °C for 3 h. The reaction mixture was evaporated, and added CH2Cl2. The mixture was washed with satd. aq. NH4Cl and brine, extracted with CH2Cl2. The organic layer was dried over anhydrous MgSO4, filtered and evaporated under reduce pressure. The residue was purified by the column chromatography (n-hexane : AcOEt = 98 : 2 to 93 : 7) to afford 35 (209 mg, 71%, E : Z = 1.0 : 4.7) as a red solid.
35 (E/Z mixture). 1H NMR (500 MHz, CDCl3): δ 1.59 (E-isomer), 2.50 (Z-isomer) (s, 3H), 6.30–8.30 (m, 15H), 9.38 (Z-isomer), 10.89 (E-isomer) (s, 1H); 13C NMR (68 MHz, CDCl3): δ 14.0, 14.2, 102.8, 102.9, 111.0, 111.4, 121.0, 121.3, 122.15, 122.24, 124.2, 124.3, 124.5, 124.8, 125.9, 126.2, 126.4, 126.8, 127.4, 127.6, 127.9, 128.0, 128.1, 128.8, 129.0, 129.2, 129.3, 129.4, 135.1, 137.4, 144.0, 193.1, 193.5; IR (film) 765, 1647, 3356 cm-1; FAB (m/z) 362 (M+H)+; HRMS (FAB) calcd for C26H20NO 362.1545 (M+H)+, found 362.1540.
2-[2-(1-Methyl-1H-indol-5-yl)-3-phenyl-inden-1-ylidene]-propionaldehyde (36).
To a solution of 1 (39 mg, 0.16 mmol) in MeCN (2 mL) were added 1-methyl-5-iodoindole (81 mg, 0.32 mmol), Pd(OAc)2 (4.5 mg, 0.02 mmol) and i-Pr2NEt (0.08 mL, 0.47 mmol) under an argon atmosphere, and the mixture was stirred at 80 °C for 4 h. The reaction mixture was evaporated, and added CH2Cl2. The mixture was washed with satd. aq. NH4Cl and brine, extracted with CH2Cl2. The organic layer was dried over anhydrous MgSO4, filtered and evaporated under reduce pressure. The residue was purified by the column chromatography (n-hexane : AcOEt = 98 : 2) to afford 36 (18 mg, 30%, E : Z = 1.0 : 3.0) as a red solid.
36 (E/Z mixture). 1H NMR (500 MHz, CDCl3): δ 1.58 (E-isomer), 2.50 (Z-isomer) (s, 3H), 3.77 (Z-isomer), 3.78 (E-isomer) (s, 3H), 6.30–8.00 (m, 14H), 9.34 (Z-isomer), 10.90 (E-isomer) (s, 1H); 13C NMR (125 MHz, CDCl3): δ 14.0, 14.2, 32.9, 101.1, 101.2, 109.2, 121.0, 121.3, 122.4, 123.7, 123.8, 126.2, 126.3, 126.8, 127.4, 127.5, 128.0, 128.1, 128.4, 128.6, 128.8, 129.15, 129.24, 129.3, 129.4, 134.2, 136.0, 136.9, 137.4, 138.3, 144.0, 144.8, 152.9, 193.0, 193.5; FAB (m/z) 375 (M)+; HRMS (FAB) calcd for C24H19O 375.1623 (M)+, found 375.1609.
2-[2-(1-Tosyl-1H-indol-5-yl)-3-phenyl-inden-1-ylidene]-propionaldehyde (37).
To a solution of 1 (25 mg, 0.10 mmol) in MeCN (1 mL) were added 1-tosyl-5-iodoindole (80 mg, 0.20 mmol), Pd(OAc)2 (2 mg, 0.01 mmol) and i-Pr2NEt (0.05 mL, 0.30 mmol) under an argon atmosphere, and the mixture was stirred at 80 °C for 21 h. The reaction mixture was evaporated, and added CH2Cl2. The mixture was washed with satd. aq. NH4Cl and brine, extracted with CH2Cl2. The organic layer was dried over anhydrous MgSO4, filtered and evaporated under reduced pressure. The residue was purified by the column chromatography (n-hexane : AcOEt = 99 : 1 to 90 : 10) to afford 37 (20 mg, 39%, E : Z = 1.0 : 7.0) as an orange solid.
37 (E/Z mixture). 1H NMR (500 MHz, CDCl3): δ 1.47 (E-isomer), 2.48 (Z-isomer) (s, 3H), 2.37 (E-isomer), 2.38 (Z-isomer) (s, 3H), 6.50–8.00 (m, 18H), 9.37 (Z-isomer), 10.87 (E-isomer) (s, 1H); 13C NMR (125 MHz, CDCl3): δ 14.1, 21.6, 109.1, 109.3, 113.8, 121.3, 122.7, 126.5, 126.6, 126.8, 127.1, 127.6, 128.1, 129.1, 129.5, 129.8, 130.0, 130.9, 132.9, 133.6, 134.1, 135.1, 137.3, 143.6, 145.1, 146.2, 152.4, 192.4; IR (film) 763, 1117, 1165, 1363, 1657 cm-1; FAB (m/z) 516 (M+H)+; HRMS (FAB) calcd for C33H26NO3S 516.1633 (M+H)+, found 516.1642.
2-(3-Phenyl-2-thiophen-2-yl-inden-1-ylidene)-propionaldehyde (38).
To a solution of 1 (50 mg, 0.20 mmol) in MeCN (2 mL) were added 2-iodothiophene (0.04 mL, 0.40 mmol), Pd(OAc)2 (4.6 mg, 0.02 mmol) and i-Pr2NEt (0.11 mL, 0.60 mmol) under an argon atmosphere, and the mixture was stirred at 80 °C for 1.3 h. The reaction mixture was evaporated, and added CH2Cl2. The mixture was washed with satd. aq. NH4Cl and brine, extracted with CH2Cl2. The organic layer was dried over anhydrous MgSO4, filtered and evaporated under reduced pressure. The residue was purified by the column chromatography (n-hexane : AcOEt = 98 : 2 to 95 : 5) to afford 38 (53 mg, 80%, E : Z = 1.0 : 5.9) as a red solid.
38 (E/Z mixture). 1H NMR (270 MHz, CDCl3): δ 1.81 (E-isomer), 2.51 (Z-isomer) (s, 3H), 6.70–8.00 (m, 12H), 9.59 (Z-isomer), 10.87 (E-isomer) (s, 1H); 13C NMR (125 MHz, CDCl3): δ 13.3, 14.3, 121.7, 121.9, 126.4, 126.6, 126.7, 126.9, 127.0, 127.1, 127.3, 127.5, 128.06, 128.14, 128.2, 128.3, 128.5, 128.7, 128.8, 128.9, 128.95, 129.03, 129.1, 129.2, 129.5, 129.7, 130.0, 130.4, 132.4, 133.5, 136.8, 138.0, 138.4, 143.3, 147.8, 151.8, 192.4, 193.1; IR (film) 1659, 2916, 3067 cm-1; FAB (m/z) 329 (M+H)+; HRMS (FAB) calcd for C22H17OS 329.1000 (M+H)+, found 329.1023.
Typical procedure for domino cyclization–allylation reaction (Table 3, entry 2).
1 (40 mg, 0.16 mmol) was dissolved in dry toluene (2 mL). MeMgBr (0.84 M THF solution) (0.21 mL, 1.1 eq) was added to the solution at –78 °C under an Ar atmosphere. After stirring at –78 °C for 1 h, the mixture was warmed to rt. Then Pd(OAc)2 (3.6 mg, 0.1 eq) and allyl bromide (42 µL) were added to the mixture and stirred for 14 h. the mixture was filtered and evaporated under reduced pressure. The residue was purified by silica gel column chromatography (n-hexane/EtOAc = 20/1) to afford 5 (21 mg, 42%) as colorless clear oil.
3-Allyl-2-benzhydryl-4,5-dimethylfuran (5): 1H NMR (270 MHz, CDCl3) δ 1.83 (s, 3H), 2.14 (s, 3H), 2.37 (d, J = 5.9 Hz, 1H), 4.92-4.97 (m, 2H), 5.38 (s, 1H), 5.75 (m, 1H), 7.12-7.27 (m, 10H); 13C NMR (68 MHz, CDCl3) δ –8.4, 11.6, 28.1, 48.5, 114.8, 115.0, 118.8, 126.3, 128.2, 128.9, 136.5, 142.4, 146.0, 148.0; IR (film) 743, 752, 910, 1031, 1116, 1597, 2920 cm-1; FAB (m/z) 302 (M)+, HRMS m/z calcd. for C22H22O; 302.1671 (M)+, found: 302.1628.
ACKNOWLEDGEMENTS
This work was financially supported by the 21st Century COE Program.
References
1. For examples of synthetic, physiological, and structural studies of cumulene, see the following references and references cited therein (a) R. Kuhn and K. Wallenfels, Ber., 1938, 71, 783; (b) R. Kuhn and H. Zahn, Chem. Ber., 1951, 84, 566; CrossRef (c) T. Kawase, S. Muro, H. Kurata, and M. Oda, J. Chem. Soc., Chem. Commun., 1992, 778; CrossRef (d) H. Kurata, S. Muro, T. Enomoto, T. Kawase, and M. Oda, Bull. Chem. Soc. Jpn., 2007, 80, 349. CrossRef
2. (a) I. Saito, K. Yamaguchi, R. Nagata, and E. Murahashi, Tetrahedron Lett., 1990, 31, 7469; CrossRef (b) A. G. Myers and P. S. Dragovich, J. Am. Chem. Soc., 1993, 115, 7021; CrossRef (c) A. L. Smith and K. C. Nicolaou, J. Med. Chem., 1996, 39, 2103. CrossRef
3. For a review on synthetic applications of allenes, see: S. Ma, Chem. Rev., 2005, 105, 2829. CrossRef
4. For examples of reactions of symmetrically substituted cumulenes, see: (a) A. Roedig and G. Zaby, Chem. Ber., 1980, 113, 3342; CrossRef (b) J. K. Crandall, D. M. Coppert, T. Schuster, and F. Lin, J. Am. Chem. Soc., 1992, 114, 5998; CrossRef (c) N. Suzaki, M. Nishiura, and Y. Wakatsuki, Science, 2002, 295, 660; CrossRef (d) N. Suzaki, Y. Fukuda, C. E. Kim, H. Takahara, M. Iwasaki, M. Saburi, M. Nishiura, and Y. Wakatsuki, Chem. Lett., 2003, 32, 16; CrossRef (e) P. H. Liu, L. Li, J. A. Webb, Y. Zhang, and N. S. Goroff, Org. Lett., 2004, 6, 2081; CrossRef (f) F. A. Akkerman and D. Lentz, Angew. Chem. Int. Ed., 2007, 46, 4902; CrossRef (g) N. Suzuki, D. Hashizume, H. Koshino, and T. Chihara, Angew. Chem. Int. Ed., 2008, 47, 5198; CrossRef (h) N. Islam, T. Ooi, T. Iwasawa, M. Nishiuchi, and Y. Kawamura, Chem. Commun., 2009, 574. CrossRef
5. For examples of the reaction of unsymmetrically substituted cumulenes, see: (a) J. G. Garcia, B. Ramos, L. M. Pratt, and A. Rodríguez, Tetrahedron Lett., 1995, 36, 7391; CrossRef (b) J. M. Aurrecoechea, E. Pérez, and M. Solay, J. Org. Chem., 2001, 66, 564. See also ref. 4a. CrossRef
6. A. B. Flynn and W. W. Ogilvie, Chem. Rev., 2007, 107, 4698. CrossRef
7. A. S. Levenson and V. C. Jordan, Eur. J. Cancer, 1999, 35, 1628. CrossRef
8. X. Wang, B. Ramos, and A. Rodriguez, Tetrahedron Lett., 1994, 35, 6977. CrossRef
9. T. Asakawa, T. Furuta, M. Iinuma, S. Fujii, K. Tanaka, and T. Kan, Chem. Lett., 2006, 512. CrossRef
10. T. Furuta, T. Asakawa, M. Iinuma, S. Fujii, K. Tanaka, and T. Kan, Chem. Commun., 2006, 3648. CrossRef
11. K. T. Sprott and E. J. Corey, Org. Lett., 2003, 5, 2465. CrossRef
12. S. Danishefsky and T. Kitahara, J. Am. Chem. Soc., 1974, 96. 7807. CrossRef
13. The configurations of each isomer have not been optimized.
14. K. Nishide, S. Ichihashi, H. Kimura, T. Katoh, and M. Node, Tetrahedron Lett., 2001, 42, 9237. CrossRef
15. R. F. Heck, Org. React., 1989, 27, 345.
16. For recent examples of palladium catalyzed indene synthesis, see: (a) F. Teplý, I. G. Stará, I. Starý, A. Kollárovič, D. Šaman, and P. Fiedler, Tetrahedron, 2002, 58, 9007; CrossRef (b) D. Zhang, E. K. Yum, Z. Liu, and R. C. Larock, Org. Lett., 2005, 7, 4963; CrossRef (c) H. Tsukamoto, T. Ueno, and Y. Kondo, J. Am. Chem. Soc., 2006, 128, 1406; CrossRef (d) L.-N. Guo, X.-H. Duan, H.-P. Bi, X.-Y. Liu, and Y.-M. Liang, J. Org. Chem., 2007, 72, 1538; CrossRef (e) J. Hitce and O. Baudoin, Adv. Synth. Catal., 2007, 349, 2054. CrossRef
17. (a) A. E. Shilov and G. B. Shul’pin, Chem. Rev., 1997, 97, 2879; CrossRef (b) S. Ma and Z. Gu, Angew. Chem. Int. Ed., 2005, 44, 7512; CrossRef (c) K. Godula and D. Sames, Science, 2006, 312, 67; CrossRef (d) L.-C. Campeau and K. Fagnou, Chem. Commun., 2006, 1253; CrossRef (e) D. Alberico, M. E. Scott, and M. Lautens, Chem. Rev., 2007, 107, 174; CrossRef (f) B.-J. Li, S.-D. Yang, and Z.-J. Shi, Synlett, 2008, 949. CrossRef
18. G. Dyker, S. Borowski, G. Henkel, A. Kellner, I. Dix, and P. G. Jones, Tetrahedron Lett., 2000, 41, 8259. CrossRef
19. For examples of domino carbopalladation/C–H activation reactions, see: (a) R. Grigg, P. Fretwell, C. Meerholtz, and V. Sridharan, Tetrahedron, 1994, 50, 359; CrossRef (b) R. Grigg, V. Loganathan, and V. Sridharan, Tetrahedron Lett., 1996, 37, 3399; CrossRef (c) S. M. Abdur Rahman, M. Sonoda, K. Itahashi, and Y. Tobe, Org. Lett., 2003, 5, 3411; CrossRef (d) H. Ohno, M. Yamamoto, M. Iuchi, and T. Tanaka, Angew. Chem. Int. Ed., 2005, 44, 5103; CrossRef (e) L. F. Tietze and F. Lotz, Eur. J. Org. Chem., 2006, 4676; CrossRef (f) A. Pinto, L. Neuville, P. Retailleau, and J. Zhu, Org. Lett., 2006, 8, 4927; CrossRef (g) A. Pinto, L. Neuville, and J. Zhu, Angew. Chem. Int. Ed., 2007, 46, 3291; CrossRef (h) M. B. Bertrand and J. P. Wolfe, Org. Lett., 2007, 9, 3073; CrossRef (i) R. T. Ruck, M. A. Huffman, M. M. Kim, M. Shevlin, W. V. Kandur, and I. W. Davies, Angew. Chem. Int. Ed., 2008, 47, 4711. CrossRef
20. The similar Pd-catalyzed cyclization reactions of in situ generated cumulene alcohol have been reported, see: (a) J. M. Aurrecoechea and E. Pérez, Tetrahedron Lett., 2001, 42, 3839; CrossRef (b) J. M. Aurrecoechea and E. Pérez, Tetrahedron, 2004, 60, 4139. CrossRef
21. The similar Pd(II)-catalyzed cyclizations and allylation of allenes including dehalopalladation process have been proposed, see, (a) S. Ma and W. Gao, J. Org. Chem., 2002, 67, 6104; CrossRef (b) B. Alcaide, P. Almendros, T. Martínez del Campo, E. Soriano, and J. L. Marco-Contelles, Chem. Eur. J., 2009, 15, 1909. CrossRef
22. For reviews on highly substituted furan, see: (a) X. L. Hou, H. Y. Cheung, T. Y. Hon, P. L. Kwan, T. H. Lo, S. Y. Tong, and H. N. C. Wong, Tetrahedron, 1998, 54, 1955; CrossRef (b) B. A. Keay, Chem. Soc. Rev., 1999, 28, 209; CrossRef (c) S. F. Kirsch, Org. Biomol. Chem., 2006, 4, 2076. CrossRef
23. For recent examples of tetrasubstituted furan synthesis, see: (a) A. W. Sromek, A. V. Kel’in, and V. Gevorgyan, Angew. Chem. Int. Ed., 2004, 43, 2280; CrossRef (b) M. H. Suhre, M. Reif, and S. F. Kirsch, Org. Lett., 2005, 7, 3925; CrossRef (c) T. Yao, X. Zhang, and R. C. Larock, J. Org. Chem., 2005, 70, 7679; CrossRef (d) Y. Liu and S. Zhou, Org. Lett., 2005, 7, 4609; CrossRef (e) R. Sanz, D. Miguel, A. Martínez, J. M. Álvarez-Gutiérrez, and F. Rodríguez, Org. Lett., 2007, 9, 727; CrossRef (f) T. Schwier, A. W. Sromek, D. M. L. Yap, D. Chernyak, and V. Gevorgyan, J. Am. Chem. Soc., 2007, 129, 9868; CrossRef (g) Y. Xiao and J. Zhang, Angew. Chem. Int. Ed., 2008, 47, 1903; CrossRef (h) X. Feng, Z. Tan, D. Chen, Y. Shen, C.-C. Guo, J. Xiang, and C. Zhu, Tetrahedron Lett., 2008, 49, 4110; CrossRef (i) M. Yoshida, M. Al-Amin, and K. Shishido, Synthesis, 2009, 2454. CrossRef