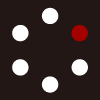
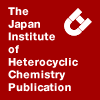
HETEROCYCLES
An International Journal for Reviews and Communications in Heterocyclic ChemistryWeb Edition ISSN: 1881-0942
Published online by The Japan Institute of Heterocyclic Chemistry
e-Journal
Full Text HTML
Received, 27th March, 2009, Accepted, 14th May, 2009, Published online, 15th May, 2009.
DOI: 10.3987/COM-09-11719
■ Flavonoid Glycosides Isolated from the Blue Flowers of Nigella damascena
Kenjiro Toki,* Norio Saito, Ai Nogami, Fumi Tatsuzawa, Atsushi Shigihara, and Toshio Honda
Laboratory of Floriculture, Faculty of Floriculture, Minami-Kyushu University, 11609 Takanabe, Koyu-gun, Miyazaki 884-0003, Japan
Abstract
Two new kaempferol glycosides (1 and 2) were isolated from the blue flowers of Nigella damascena cv ‘Ms Jekyll Blue’ along with a known anthocyanin (3). The structures of 1 and 2 were elucidated by application of chemical and spectroscopic methods. Compound (3) was a rarely observed anthocyanin in plants.INTRODUCTION
Nigella damascena (Ranunculaceae) is a common garden plant with white, pink or blue flowers. As part of our continuing investigation on flower color variation due to anthocyanins of ornamental plants in the Ranunculaceae, we have already reported the distribution of some complicated anthocyanins in the flowers of Consolida armeniaca,1 Ranunculus asiaticus,2 Delphinium hybridum3 and Anemone coronaria.4,5,6 In this study, we investigated the flavonoid pigments in the blue flowers of N. damascena. From this experiment, two new kaempferol glycosides as main flavonoids and a rare anthocyanin were isolated from the flower sepals of N. damascena. In this paper, we wish to report the structure elucidation of these compounds.
RESULTS AND DISCUSSION
Two flavonol glycosides (1 and 2) and an anthocyanin (3)7 were isolated from the flower sepals by column chromatography (CC), paper chromatography (PC) and HPLC. Acid hydrolyses of 1 and 2 yielded kaempferol, galactose, glucose and rhamnose. On the other hand, a similar hydrolysis of 3 gave petunidin, glucose, xylose and rhamnose.
Alkaline hydrolysis of 1 resulted in one deacylflavonol glycoside, which was identified as 2 by analyses of HPLC and TLC. Moreover, 3-hydroxy-3-methylglutaric acid was detected in its hydrolysate by analyses of HPLC and TLC.
The FAB mass spectrum of 1 gave its molecular ion [M+1]+ at m/z 901 in good agreement with the mass calculated for C39H49O24 (901.261), which was supposed to be composed of kaempferol with one molecule each of galactose, glucose, rhamnose (based on the results of its acid hydrolysis) and a certain acid having a molecular formula of C6H10O5. The assumed structure for the acid was finally determined to be 3-hydroxy-3-methylglutaric acid .by direct comparison with the authentic specimen purchased from TCI Co., Ltd. The elemental components of 1 were further confirmed by high resolution FAB-MS experiment. The structural analysis of 1 was performed mainly on the basis of 1H and 13C NMR spectral analyses in DMSO-d6 solution, and proton and carbon chemical shifts assigned were shown in Table 1. Six aromatic proton signals of kaempferol moiety were readily assigned. The chemical shifts of the sugar moieties were observed in the region of δ 1.14 – 5.63, where three anomeric protons were exhibited at δ 5.63 (d, J=7.6 Hz, H-1 galactose), δ 4.59 (d, J=7.7 Hz, H-1 glucose) and δ 5.53 (d, J=1.6 Hz, H-1 rhamnose). Based on the observed coupling constants (Table 1), galactose and glucose were determined to be β-pyranose forms and rhamnose to be in the α-pyranose form. Seven proton signals of 3-hydroxy-3-methylglutaric acid moiety were observed in the region of δ 1.03 – 2.37 (Table 1). In the NOESY spectrum, the correlations between H-6 or H-8 of kaempferol and H-1 of rhamnose, and H-2 of galactose and H-1 of glucose were observed, suggesting that OH-7 of kaempferol was glycosylated with rhamnose and OH-2 of galactose was bonded with OH-1 of glucose (Figure 1). Acyl moiety at the OH-6 of galactose was presumed to be 3-hydroxy-3-methylglutaric acid on the basis of its molecular formula, and further identified by observing correlation between the C-1” of the acid and H-6a and H-6b of galactose in its HMBC spectrum. From the results of UV spectroscopic experiment, 1 (350 nm) was confirmed to be glycosylated with galactose at the OH-3 of kaempferol because of hypsochromic effect of 3-glycosylation (kaempferol 367 nm in MeOH).8 Therefore, 1 was determined to be kaempferol 3-O-[2-O-(β-glucopyranosyl)-6-O-(3-hydroxy-3-methylglutaroyl)-β-galactopyranoside]-7-O-(α-rhamno-pyranoside), which is a new flavonol glycoside in plants.9,10,11
The FAB mass spectrum of 2 gave its molecular ion [M+1]+ at m/z 757 in agreement with the mass calculated for C33H41O20 (757.219), which was composed of kaempferol with one molecule each of galactose, glucose and rhamnose. As mentioned before, 2 was identified as the same structure of deacyl compound of 1 by analysis of TLC and HPLC indicating that the structure of 2 was kaempferol 3-(2-glucosylgalactoside)-7-rhamnoside. This structure was further confirmed by analysis of its 1H and 13C NMR spectra as shown in Table 1. Observed six aromatic proton signals of kaempferol were readily assigned as shown in Table 1. The proton signals of its sugar moieties were similar to those of 1 except for H-6a and 6b (δ 3.25 – 3.45) of galactose moiety. Those signals are shifted to higher magnetic field than those (δ 3.97, dd, J=7.7 and 11.3 Hz, and δ 3.91, dd, J=4.3 and 11.3 Hz) of 1, supporting that OH-6 of galactose moiety in 2 is free from 3-hydroxy-3-methylglutaric acid. Therefore, flavonol (2) was determined to be kaempferol 3-O-[2-O-(β-glucopyranosyl)-β-galactopyranoside]-7-O-(α- rhamnopyranoside), which is also a new flavonol glycoside in plants.7,8,9
The FAB mass spectrum of 3 gave a molecular ion [M]+ at 757 m/z [calc. for C33H41O20 (757.219)], indicating that 3 is composed of petunidin with one molecule each of glucose, xylose and rhamnose. The 1H NMR spectrum (500 MHz in DCl-CD3OD, 1:9) of 3 exhibited five aromatic proton signals in addition to three methyl proton signals of petunidin.12 The anomeric protons of sugar moieties were observed at δ 5.56 (d, J=7.6 Hz, Glucose H-1), δ 4.72 (d, J=7.6 Hz, xylose H-1), and δ 4.62 (d, J=1.5 Hz, rhamnose H-1). Glucose and xylose moieties in 3 were determined to be in the β-pyranose forms and the rhamnose moiety to be in the α-pyranose form based on their observed coupling constants.
By application of NOESY experiment, the NOEs between H-1 of glucose and H-4 of petunidin, H-2 of glucose and H-1 of xylose, and H-6a and 6b of glucose and H-1 of rhamnose were observed (Fig. 2). These results suggested that OH-3 of petunidin was glycosylated with glucose, and also OH-2 and OH-6 of glucose were bonded with xylose and rhamnose, respectively. Therefore, the anthocyanin (3) was determined to be petunidin 3-(2G-xylosylrutinoside), which has been already reported in the plant of Vaccinium padifolium.7 However, its distribution is very rare and this is second report in plants.
CONCLUDING REMARKS
At present, it was proved that petunidin 3-(2G-xylosylrutinoside) (3) was a major anthocyanin in the blue flowers of Nigella damascene, and new kaempferol glycosides (1 and 2) were also present in this flowers. Both flavonols might be considered to take part in keeping the stability and bluing of their flower color such as the typical co-pigments for 3 in this plant. The detail functional study of both flavonols will be reported elswhere. From the chemotaxonomical point of view in the Ranunculaceae, there are several glycosylation patterns classified by the types of di-saccharides at the OH-3 of anthocyanidin. The 2G-xylosylrutinoside in this plant is comparable to 2-xylosylglucoside in Anemone,13,14 Aquilegia15 and Ranunculus,2 and rutinoside in Aconitum16 and Delphinium.3,17,18 Furthermore, two other patterns were also reported in this family, such as 2-glucosylgalactoside in Pulsatilla19 and Anemone,5,6 and 2-xylosylgalactoside in Anemone.4 As the similar patterns of flavonol glycosides by di- or tri-saccharides at 3 or 7-OH in this family, there are ten patterns such as rutinoside in Aconitum20 and Ranunculus,21 sophoroside in Aconitum,22,23,24 Ranunculus,21 Delphinium24,25 and Helleborus,26,27 gentiobioside in Aconitum,20 sambubioside in Helleborus,26,27 arabinosyl(1-6)glucoside in Thalictrum,28 arabinosyl(1-2)glucoside in Helleborus,29 glucosyl(1-3)- rhamnoside in Aconitum,23,30 rhamnosyl(1-6)galactoside in Aconitum,31 glucosyl(1-2)galactosyl(1-2)- glucoside in Nigella32 and 3R-glucosylrutinoside in Aconitum.31 It is noteworthy that the glucosyl(1-2)galactoside pattern in this study is a new pattern in the Ranunculaceae, and also kaempferol 3-glucosyl(1-2)galactoside-7-rhamnoside is a new glycoside pattern of these kaempferol glycosides in these plants.
EXPERIMENTAL
General procedures
TLC was carried out on plastic sheets or glass plate coated cellulose (Merck) using twelve mobile phases: BAW (n-BuOH-HOAc-H2O, 4:1:5), BuHCl (n-BuOH-2N HCl, 1:1), HOAc-HCl (HOAc-HCl-H2O, 15:3:82), 1% HCl for anthocyanins, BAW, H2O, 15% HOAc, PhOH (PhOH-H2O, 4:1) for flavonols, BAW, i-PrOH-H2O (4:1), i-PrOH-n-BuOH-H2O (7:1:2), PhOH for sugars and BAW, H2O, 6%HOAc for phenolic acids, BAW, EAW (EtOAc-HOAc-H2O, 3:1:1), EWN (EtOH-H2O-NH4OH, 16:3:1) with UV light, bromocresol green and aniline hydrogen phthalate spray reagent.33 The identification of flavonoids was carried out by standard procedures.33 Analytical HPLC was performed on Hitachi 6200 system, using an Inertsil ODS-2 (4.6 x 250 mm) column at 35 °C with a flow rate of 0.8 mL/min and monitoring at 520 nm and 360 nm. The eluant was applied as a linear gradient elution for 40 min from 25% to 85% solvent B (1.5% H3PO4, 20% HOAc, 25% MeCN in H2O) in solvent A (1.5% H3PO4 in H2O). UV-Vis spectra were recorded on UV-Vis spectrophotometer (MPS-2450, Shimadzu) in 0.1% HCl-MeOH for anthocyanin and in MeOH for flavonols. FAB mass spectra were obtained in the positive ion mode using the magic bullet (5:1 mixture of dithiothreitol and dithioerythritol) as a matrix. NMR spectra were acquired at 500 MHz for 1H spectra and 125.78 MHz for 13C spectra in DMSO-d6 for flavonols and DCl-CD3OD (9:1) for anthocyanin. Chemical shifts are reported relative to a TMS internal standard (δ), and coupling constants are in Hz.
Plant materials
Seeds of N. damascene cv ‘Ms Jekyll Blue’ were purchased from Fukukaen Seed Co., Ltd, Nagoya. The
plants were grown in the green house of Minami-Kyushu University. The fresh flower sepals were collected in April – June, and dried at 45 °C, and stored in desiccators until needs.
The chromaticity value of the fresh flowers of this cultivar, b/a = -3.5 by SE-2000 Spectro Color Meter (Nippon Denshoku Industries Co., Ltd.).
Isolation of flavonols and anthocyanin
The dried flowers (48 g) were extracted with 5% HOAc (10 L) at rt. Flavonoids in the extract were absorbed on a Diaion HP-20 column, and after re-extracted with MeOH-HOAc-H2O (75:5:25), the eluant was concentrated into a small volume. The concentrated extract was fractionated with Sephadex LH-20 CC using MeOH-HOAc-H2O (6:1:12). The fractions were further purified with PC (n-BuOH-HOAc-H2O, 4:1:2 and 15% HOAc) and prep. HPLC. Prep. HPLC was performed on a Hitachi 6200 system using an Inertsil ODS-2 column (20 x 250 mm) with HOAc solvent. Three major pigments, 1 (550 mg) and 2 (69 mg) as pale yellow powders, 3 (57 mg) as a dark red powder were obtained
Kaempferol 3-[2-(glucosyl)-6-(3-hydroxy-3-methylglutaroyl)-galactoside]-7-rhamnoside (1).
Pale yellow powder, TLC (Rf values x 100); BAW 42, 15% HOAC 86, H2O 73, PhOH 71, UV dark brown, UV/NH3 yellow, UV (λmax nm); MeOH 351, 266, +NaOMe 396, 266, +AlCl3 363, 274, +AlCl3/HCl 362, 275, +NaOAc 351, 267, +NaOAc/H3BO3 350, 267, HPLC (Rt min) 21.1, HR-FAB MS calc. for C39H49O24 [M+1]+ 901.2614, found 901.2602.
Kaempferol 3-(2-glucosylgalactoside)-7-rhamnoside (2).
Pale yellow powder, TLC (Rf values x 100); BAW 42, 15% HOAC 80, H2O 81, PhOH 73, UV dark brown, UV/NH3 yellow, UV (λmax nm); MeOH 351, 266, +NaOMe 397, 266, +AlCl3 365, 275, +AlCl3/HCl 365, 275, +NaOAc 352, 266, +NaOAc/H3BO3 351, 266, HPLC (Rt min) 19.2, HR-FAB MS calc. for C33H41O20 [M+1]+ 757.2191, found 757.2090.
Petunidin 3-(2G-xylosylrutinoside) (3).
Dark red powder, TLC (Rf values x 100); BAW 51, BuHCl 42, 1% HCl 68, HOAc-HCl 91, UV-Vis (in 0.1% HCl-MeOH, λmax nm); 540, 273, E440/Emax (%) 23, +AlCl3 +shift, HPLC (Rt min) 16.4, FAB MS m/z 757. 1996 [M]+ (calc. for C33H41O20 757.2191).
References
1. N. Saito, K. Toki, S. Özden, and T. Honda, Phytochemistry, 1996, 41, 1599. CrossRef
2. K. Toki, M. Takeuchi, N. Saito, and T. Honda, Phytochemistry, 1996, 42, 1055. CrossRef
3. N. Saito, K. Toki, A. Suga, and T. Honda, Phytochemistry, 1998, 49, 881. CrossRef
4. K. Toki, N. Saito, A. Shigihara, and T. Honda, Phytochemistry, 2001, 56, 711. CrossRef
5. N. Saito, K. Toki, and T. Honda, Phytochemistry, 2002, 60, 365. CrossRef
6. K. Toki, N. Saito, A. Shigihara, and T. Honda, Heterocycles, 2003, 60, 345. CrossRef
7. L. Cabrita, N. A. Froystein, and O. M. Andersen, Food Chem., 2000, 69, 33. CrossRef
8. T. J. Mabry, K. R. Markham, and M. B. Thomas, “The Systematic Identification of Flavonoids”, Springer-Verlag, Berlin, 1970.
9. J. B. Harborne, and H. Baxter, “The Handbook of Natural Flavonoids” John Wiley & Sons. Chichester, 1999. CrossRef
10. C. A. Williams, Flavone and Flavonol O-Glycosides. In: Andersen, O. M., Markham, K. R. (Eds.). “Flavonoids: Chemistry Biochemistry and Applications”, CRC Press, Boca Raton, 2006. p.749. CrossRef
11. N. C. Veitch and R. J. Grayer, Flavonoids and their glycosides, including anthocyanins. Nat. Prod. Rep., 2008, 25, 555. CrossRef
12. T. Ando, N. Saito, F. Tatsuzawa, T. Kakefuda, K. Yamakage, E. Ohtani, M. Koshi-ishi, Y. Matsusake, H. Kokubun, H. Watanabe, T. Tsukamoto, Y. Ueda, G. Hashimoto, E. Marchesi, K. Asakura, R. Hara, and H. Seki, Biochem. Syst. Ecology, 1999, 27, 623. CrossRef
13. J. B. Harborne, “Comparative Biochemistry of the Flavonoids”, Acad. Press, London and New York, 1967.
14. J. Raynaud and G. Netain, Ann. Pharm. Franc, 1971, 29, 449.
15. R. J. Taylor, Bull. Torrey Bot. Club., 1984, 111, 462. CrossRef
16. K. Takeda, S. Sato, H. Kobayashi, Y. Kanaitsuka, M. Ueno, T. Kinoshita, H. Zazeki, and T. Fujimori, Phytochemistry, 1994, 36, 613. CrossRef
17. T. Kondo, K. Oki, K. Yoshida, and T. Goto, Chem. Lett., 1990, 137. CrossRef
18. T. Goto, K. Suzuki, K. Yoshida, K. Oki, M. Ueda, M. Isobe, and T. Goto, Tetrahedron Lett., 1991, 32, 6375. CrossRef
19. K. Yoshitama, A. Saeki, T. Iwata, N. Ishikura, and S. Yahara, Phytochemistry, 1998, 47, 105. CrossRef
20. D. A. Young and R. W. Sterner, Phytochemistry, 1981, 20, 2055. CrossRef
21. K. Gluchoff-Fiasson, I. L. Fiasson, and H. Watson, Phytochemistry, 1997, 45, 1063. CrossRef
22. G. Fico, A. Braca, A. R. Billa, F. Tomé, and I. Morelli, Planta Med., 2001, 67, 287. CrossRef
23. A. Braca, G. Fico, I. Morelli, F. de Simone, F. Tomé, and N. de Tommasi, J. Ethnopharmacology, 2003, 86, 63. CrossRef
24. J. C. Luis, F. Valdés, R. Martin, A. J. Carmona, and J. G. Diaz, Fitoterapia, 2006, 77, 469. CrossRef
25. M. J. Warnock, Y. L. Liu, and T. J. Mabry, Phytochemistry, 1983, 22, 1834. CrossRef
26. J. B. Harborne, Phytochemistry, 1965, 4, 107. CrossRef
27. K. Egger and M. Keil, Ber. dt. Bot. Ges., 1965, 78, 153.
28. G. Guangyao, C. Sibao, Y. Junshan, and X. Peigen, Fitoterapia, 2000, 71, 627. CrossRef
29. J. M. Prieto, T. Siciliano, and A. Braca, Fitoterapia, 2006, 77, 203. CrossRef
30. G. Fico, A. Braca, N. de Tommasi, F. Tomé, and I. Morelli, Phytochemistry, 2001, 57, 543. CrossRef
31. C. Mariani, A. Braca, S. Vitalini, N. de Tommasi, F. Visioli, and G. Fico, Phytochemistry, 2008, 69, 1220. CrossRef
32. I. Merfort, Phytochemistry, 1997, 46, 359. CrossRef
33. J. B. Harborne, “Phytochemical Methods”, Second ed., Chapman and Hall, London, 1984. CrossRef