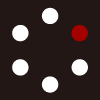
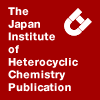
HETEROCYCLES
An International Journal for Reviews and Communications in Heterocyclic ChemistryWeb Edition ISSN: 1881-0942
Published online by The Japan Institute of Heterocyclic Chemistry
e-Journal
Full Text HTML
Received, 3rd April, 2009, Accepted, 14th May, 2009, Published online, 18th May, 2009.
DOI: 10.3987/COM-09-11725
■ Synthesis of Pyrrolo[2,3-d]pyrimidine Analogues: “Pyridine Ring” Analogues of Pemetrexed
Yun Xu, Mingfeng Yu, Yan Long, Han Wu, and Zhenmin Mao*
School of Pharmacy, Shanghai Jiaotong University , 800 Dongchuan Road, Shanghai 200240, China
Abstract
Two analogues of pemetrexed with its phenyl ring replaced with pyridine ring as novel anticancer agents were synthesized. Preliminary in vitro evaluation indicated that replacement of the phenyl moiety of pemetrexed by the pyridine ring with the 6-5 bicyclic ring system showed low cytotoxicity, that departs from the findings with antifolates bearing 6-6 bicyclic ring system.Pemetrexed (LY231514, 1) is a novel 6-5 bicyclic pyrrolo[2,3-d]pyrimidine antifolate that shows remarkable activity against a broad spectrum of solid tumors. Pemetrexed was approved as a multitargeted antifolate (MTA) by FDA in 2004 for the treatment of malignant pleural mesothelioma in combination with cisplatin and as a single-agent in the treatment of non-small-cell lung cancer.1 The clinical success of pemetrexed has generated renewed interest in the design and synthesis of antifolates that function as multi-inhibitors for folate-dependent enzymes.2, 3
Antifolates bearing classical 6-6 bicyclic ring system, such as pteridine, deazapteridine and quinazoline, could tolerate changes in phenyl group in some extent without the loss of cytotoxic potency.4 It is of interests to further explore the effect of change of phenyl ring with other ring system, such as pyridine in pemetrexed, while retain its 6-5 bicyclic pyrrolo[2,3-d]pyrimidine moiety. We describe herein the synthesis and biological activity of two close structural analogues of pemetrexed with the substitution of phenyl ring by pyridine, 2-amino-4-oxo-5-substituted-pyrrolo[2,3-d]pyrimidine (2a) and 2,4-diamino-5-substituted-pyrrolo[2,3-d]pyrimidine (2b).
Retrosynthetic analysis suggested that structures 3a, 3b and 4 were key precursors to the synthesis of target compounds 2a and 2b (Scheme 1). Taylor and Liu5 have demonstrated a synthetic approach for construction of the bicyclic pyrrolo[2,3-d]pyrimidine ring system through the coupling of aminopyrimidine and nitroolefin structure in the presence of strong base. The synthesis employs a Michael addition and a following Nef reaction. The Michael addition happens between the unsubstituted C-5 positions of 2,6-diaminopyrimidin-4(3H)-one (3a) and 2,4,6-triaminopyrimidine (3b) to nitroolefin group of 4. The adducts then are undergone Nef reaction by converting the nitro group into a aldehyde which cyclizes with the C-6 amino substituent to form the 6-5 bicyclic ring system.6
The synthesis of the requisite precursor 6-(4-nitrobut-3-enyl)nicotinic acid methyl ester (4) was started from a palladium-catalyzed coupling of methyl 6-bromonicotinate (5) with allyl alcohol to form 6-(3-oxopropyl)nicotinic acid methyl ester (6) in 91% yield,7 which underwent aldol condensation with nitromethane to give the nitro alcohol 7 in 50% yield, followed by dehydration8 through conversion of hydroxyl to methanesulfonyl ester with MsCl and subsequent elimination with triethylamine to afford the key nitroolefin intermediate 4 in 96% yield.
Michael addition of 2,6-diaminopyrimidin-4(3H)-one (3a) to the nitroolefin group of 4 was proceeded at 50 °C to yield the adduct 8a. The crucial intramolecular cyclization of 8a to the pyrrolo[2,3-d]pyrimidine 9a was achieved by stirring with NaOH at 25 °C for 2 h followed by addition into HCl at -5 °C for 3 h, the mixture was neutralized by addition of NaOH and then acidified with AcOH in excellent overall yield. This procedure includes a three-step conversion: a Nef reaction which transform the nitro group to aldehyde with treatment of NaOH and subsequent HCl, followed by a intramolecular condensation between the aldehyde and the 6-amino group in acidic condition and a final aromatization.5 This one-pot operation efficiently resulted in not only the formation of the desired 6-5 bicyclic ring system, but also a necessary saponification of the carbonyl eater to carboxylic acid for the later coupling with glutamate in this case.
The synthesis of penultimate 10a was accomplished by coupling of the carboxylic acid 9a to glutamate with the activation of the carboxylic acid by 2,4-dimethoxy-6-chloro-1,3,5-triazine and N-methylmorpholine.9 Final hydrolysis of 10a with 1N NaOH followed by acidification with AcOH yielded the target compound 2a. The 4-amino substituted target compound 2b was synthesized from 2,4,6-triaminopyrimidine (3b) by procedures similar to those of 2a.
In vitro cell culture screening of target compounds 2a and 2b, however, shown that both target compounds were not active against many cancer cells with low cytotoxicity (>20 μg/mL). This result might indicate that the pyridine substitution of phenyl ring in antifolates bearing the 6-5 bicyclic ring system is not well tolerated, that departs from the findings with antifolates bearing 6-6 bicyclic ring system.
EXPERIMENTAL
General. 1H NMR and 13C NMR were recorded on a Varian-300 instrument. The chemical shift values were expressed in ppm (parts per million) relative to tetramethylsilane (TMS) as internal standard. Mass spectra were obtained on Agilent 1100 series LC-MSD and HP 1100 LC-MS spectrometers. Melting points were determined on a RY-2 microscopic melting point apparatus and were uncorrected. Column chromatography was carried out with silica gel (200-300 mesh).
6-(3-Oxopropyl)nicotinic acid methyl ester (6). A mixture of methyl 6-bromonicotinate 5 (1.1 g, 5.0 mmol), allyl alcohol (0.5 mL, 7.5 mmol), NaHCO3 (1.1 g, 10.0 mmol), Pd(OAc)2 (56.1 mg, 0.25 mmol), (n-Bu)4NBr (1.9 g , 6.0 mmol) in DMF (10 mL) was stirred for 72 h at 25 °C under nitrogen. The reaction mixture was filtered, and the filtrate was poured into H2O and extracted with hexane (5×20 mL). The combined extracts were dried over anhydrous Na2SO4 and concentrated in vacuo. The residue was purified by column chromatography (silica gel/hexanes: EtOAc = 10: 1) to give 0.88 g (91%) of 6 as a clear oil. MS (ESI): m/z 194 [M+H]+. 1H NMR (300 MHz, CDCl3) δ: 9.82 (s, 1H, CHO), 8.81 (d, 1H, J=2.4Hz, 2-H), 7.97 (dd, 1H, J=2.4, 8.4Hz, 4-H), 7.26 (d, 1H, J=8.4Hz, 5-H), 3.90 (s, 3H, CH3), 3.01 (t, 2H, J=7.5Hz, 6-CH2CH2CHO), 2.81 (t, 2H, J=7.5Hz, 6-CH2CH2CHO). 13C NMR (75 MHz, CDCl3) δ: 200.9, 167.1, 161.0, 146.0, 130.1, 128.6, 128.5, 52.2, 45.0, 28.3. HRMS: Calcd for C10H11NO3: 193.0739; found: 193.0737.
6-(3-Hydroxy-4-nitrobutyl)nicotinic acid methyl ester (7). To a solution of 6 (0.55 g, 2.85 mmol) in MeOH (5 mL) at 25 °C was added nitromethane (0.21 g, 3.44 mmol) followed by addition of sodium hydroxide (6 mg, 0.15 mmol) in MeOH (1 mL) at 0 °C. The mixture was stirred at 35 °C for 48 h. Then the mixture was concentrated in vacuo. The residue was purified by column chromatography (silica gel/hexanes: EtOAc = 5: 1) to give 0.36 g (50%) of 7 as a light yellow solid, mp 65~67 °C. MS (ESI): m/z 255 [M+H]+. 1H NMR (300 MHz, CDCl3) δ: 8.82 (d, 1H, J=2.4Hz, 2-H), 7.99 (dd, 1H, J=2.4, 7.8Hz, 4-H), 7.29 (d, 1H, J=7.8Hz, 5-H), 4.36 (d, 2H, J=6.6Hz, CH2NO2), 4.25 (m, 1H, CHOH), 3.92 (s, 3H, CH3), 2.88 (m, 2H, 6-CH2CH2), 2.50~3.00 (br s, 1H, OH), 1.86 (m, 2H, 6-CH2CH2). 13C NMR (75 MHz, CDCl3) δ: 167.3, 161.9, 146.4, 130.2, 128.7, 128.6, 80.7, 67.8, 52.3, 34.9, 31.6. HRMS: Calcd for C11H14N2O5: 254.0903; found: 254.0910.
6-(4-Nitrobut-3-enyl)nicotinic acid methyl ester (4). To a solution of 7 (0.36 g, 1.42 mmol) in dry CH2Cl2 (5 mL) at 0 °C was added methanesulfonyl chloride (0.15 mL, 1.92 mmol) followed by addition triethylamine (0.4 mL, 2.84 mmol). After 3 h, the mixture was warmed to 25 °C, and poured into H2O (10 mL) and extracted with CH2Cl2 (2×10 mL). The combined extracts were dried over anhydrous Na2SO4 and concentrated. The residue was purified by column chromatography (silica gel/hexanes: EtOAc = 10: 1) to give 0.32 g (96%) of 4 as a white solid, mp 69~71 °C. MS (ESI): m/z 237 [M+H]+. 1H NMR (300 MHz, CDCl3) δ: 8.82 (d, 1H, J=2.4Hz, 2-H), 7.99 (dd, 1H, J=2.4, 8.4Hz, 4-H), 7.26 (m, 2H, 5-H, CHCHNO2), 6.96 (d, 1H, J=13.5Hz, CHCHNO2), 3.91 (s, 3H, CH3), 2.90 (t, 2H, J=7.5Hz, 6-CH2CH2), 2.62 (q, 2H, J=7.5Hz, 6-CH2CH2). 13C NMR (75 MHz, CDCl3) δ: 167.1, 160.2, 145.1, 141.0, 140.4, 130.3, 128.9, 128.6, 52.4, 34.1, 29.9. HRMS: Calcd for C11H12N2O4: 236.0797; found: 236.0798.
6-[3-(2,4-Diamino-6-oxo-1,6-dihydropyrimidin-5-yl)-4-nitrobutyl]nicotinic acid methyl ester (8a). A mixture of 4 (0.30g, 1.27 mmol) and 2,6-diaminopyrimidin-4(3H)-one 3a (0.20 g, 1.59 mmol) in H2O (7.5 mL) and EtOAc (7.5 mL) was stirred at 50 °C for 24 h. The reaction mixture was poured into EtOAc (50 mL) and washed with H2O (2×10 mL). The combined extracts were dried over anhydrous Na2SO4 and concentrated. The residue was purified by column chromatography (silica gel/CH2Cl2: MeOH = 20: 1) to give 0.30 g (65%) of 8a as a yellow solid, mp 216~218 °C. MS (ESI): m/z 363 [M+H]+. 1H NMR (300 MHz, DMSO-d6) δ: 9.76 (s, 1H, 3-H), 8.66 (d, 1H, J=2.4Hz, pyridine 2-H), 7.83 (dd, 1H, J=2.4, 8.4Hz, pyridine 4-H), 7.25 (d, 1H, J=8.4Hz, pyridine 5-H), 6.03 (s, 2H, 6-NH2), 5.93 (s, 2H, 2-NH2), 5.00, 4.75 (2m, 2H, CH2NO2), 3.81 (s, 3H, CH3), 3.40 (m, 1H, 5-CHCH2CH2), 2.63, 2.50 (2m, 2H, 5-CHCH2CH2), 2.13, 1.70 (2m, 2H, 5-CHCH2CH2). 13C NMR (75 MHz, DMSO-d6) δ: 166.9, 163.6, 162.6, 162.5, 154.2, 148.9, 129.9, 129.1, 127.8, 112.6, 78.3, 52.6, 35.7, 33.7, 31.9. HRMS: Calcd for C15H18N6O5: 362.1339; found: 362.1342.
6-[2-(2-Amino-4-oxo-4,7-dihydro-3H-pyrrolo[2,3-d]pyrimidin-5-yl)ethyl]nicotinic acid (9a). A mixture of 8a (0.10 g, 0.28 mmol) in 2 N NaOH (2.5 mL) was stirred at 25 °C for 2 h and then was slowly added into 2.5 N HCl (3 mL) at -5 °C. After 3 h, the pH of the reaction mixture was adjusted to 7 with 2 N NaOH. The mixture was warmed to 25 °C, and stirred for another 1 h and added AcOH to adjust the pH to 3. The solid was filtered, washed with H2O, EtOAc and dried under vacuum to give 9a (0.80 g, 95%) as a green solid without further purification for the next reaction, mp >300 °C. MS (ESI): m/z 300 [M+H]+. 1H NMR (300 MHz, DMSO-d6) δ: 12.40~13.20 (br s, 1H, COOH), 10.60 (s, 1H, 7-H), 10.13 (s, 1H, 3-H), 8.65 (d, 1H, J=2.4Hz, pyridine 2-H), 7.82 (dd, 1H, J=2.4, 8.4Hz, pyridine 4-H), 7.30 (d, 1H, J=8.4Hz, pyridine 5-H), 6.30 (s, 1H, 6-H), 5.98 (s, 2H, 2-NH2), 2.97 (t, 2H, J=6.9Hz, 5-CH2CH2), 2.83 (t, 2H, J=6.9Hz, 5-CH2CH2). 13C NMR (75 MHz, DMSO-d6) δ: 168.0, 159.9, 152.9, 152.0, 149.8, 148.5, 129.9, 129.2, 128.9, 118.3, 114.1, 99.4, 37.0, 28.6. HRMS: Calcd for C14H13N5O3: 299.1018; found: 299.1012.
N-{6-[2-(2-Amino-4-oxo-4,7-dihydro-3H-pyrrolo[2,3-d]pyrimidin-5-yl)ethyl]nicotinoyl}-L-glutamic acid diethyl ester (10a). To a suspension of acid 9a (50 mg, 0.17 mmol) in DMF (5 mL) was added N-methylmorpholine (20 µL, 0.18 mmol) followed by 2-chloro-4,6-dimethoxy-1,3,5–triazine (32 mg, 0.18 mmol), and the solution was stirred at 25 °C for 2 h. Another portion of N-methylmorpholine (20 µL, 0.18 mmol) was added to the solution followed by diethyl L-glutamate hydrochloride (44 mg, 0.18 mmol), and the mixture was stirred at 25 °C for 4 h. The solvent was removed under reduced pressure, and the residue was purified by column chromatography (silica gel/CH2Cl2: MeOH = 20: 1) to give 10a (73 mg , 89%) as a white solid, mp 137~139 °C. MS (ESI): m/z 485 [M+H]+. 1H NMR (300 MHz, DMSO-d6) δ: 10.59 (s, 1H, 7-H), 10.13 (s, 1H, 3-H), 8.61 (d, 2H, J=6.9Hz, CONH, pyridine 2-H), 7.76 (dd, 1H, J=2.4, 8.4Hz, pyridine 4-H), 7.27 (d, 1H, J=8.4Hz, pyridine 5-H), 6.29 (d, 1H, 6-H), 5.99 (s, 2H, 2-NH2), 4.40 (m, 1H, glutamate α-CH), 4.08, 4.03 (2q, 4H, J=6.9Hz, 2CH2CH3), 2.96 (m, 2H, 5-CH2CH2), 2.84 (m, 2H, 5-CH2CH2), 2.42 (t, 2H, J=7.5Hz, glutamate γ-CH2), 2.04 (m, 2H, glutamate β-CH2), 1.16 (m, 6H, 2CH2CH3). 13C NMR (75 MHz, DMSO-d6) δ: 173.3, 172.9, 167.7, 156.1, 155.2, 151.9, 151.1, 146.5, 132.3, 129.5, 128.4, 117.7, 116.2, 99.4, 61.6, 61.0, 53.1, 36.4, 31.3, 28.1, 26.8, 15.1. HRMS: Calcd for C23H28N6O6: 484.2070; found: 484.2069.
N-{6-[2-(2-Amino-4-oxo-4,7-dihydro-3H-pyrrolo[2,3-d]pyrimidin-5-yl)ethyl]nicotinoyl}-L-glutamic acid (2a). To a solution of 10a (50 mg, 0.10 mmol) in THF (5 mL) was added 1 N NaOH (2 mL). The mixture was stirred at 25 °C for 3 h. The solvent was evaporated under reduced pressure, and the residual solution was acidified with AcOH. The precipitate was collected by filtration, washed with H2O, EtOAc, and Et2O, and dried under reduced pressure to give 2a (30 mg, 70%) as a green solid, mp 190~192 °C. MS (ESI): m/z 429 [M+H]+. 1H NMR (300 MHz, DMSO-d6) δ: 10.58 (s, 1H, 7-H), 10.13 (s, 1H, 3-H), 8.58 (d, 1H, J=2.4Hz, pyridine 2-H), 8.41 (d, 1H, J=8.1Hz, CONH), 7.75 (dd, 1H, J=2.4, 8.4Hz, pyridine 4-H), 7.26 (d, 1H, J=8.4Hz, pyridine 5-H), 6.28 (d, 1H, 6-H), 5.98(s, 2H, 2-NH2), 4.35 (m, 1H, glutamate α-CH), 2.94 (m, 2H, 5-CH2CH2), 2.83 (m, 2H, 5-CH2CH2), 2.32 (t, 2H, J=7.8Hz, glutamate γ-CH2), 1.96 (m, 2H, glutamate β-CH2). 13C NMR (75 MHz, DMSO-d6) δ: 174.8, 174.2, 166.8, 160.0, 152.9, 146.7, 132.2, 128.9, 127.9, 118.3, 114.1, 99.4, 52.8, 36.8, 31.7, 28.7, 27.2. HRMS: Calcd for C19H20N6O6: 428.1444; found: 428.1450.
6-[4-Nitro-3-(2,4,6-triaminopyrimidin-5-yl)butyl]nicotinic acid methyl ester (8b). Prepared from 4 (0.56 g, 2.36 mmol) and pyrimidine-2,4,6-triamine 3b (0.36 g, 2.88 mmol) as described for the preparation of 8a, obtained 8b (0.56 g, 66%) as a yellow solid, mp 89~91 °C. MS (ESI): m/z 362 [M+H]+. 1H NMR (300 MHz, DMSO-d6) δ: 8.86 (d, 1H, J=2.4Hz, pyridine 2-H), 7.83 (dd, 1H, J=2.4, 8.4Hz, pyridine 4-H), 7.27 (d, 1H, J=8.4Hz, pyridine 5-H), 5.64 (br s, 4H, 4-NH2, 6-NH2), 5.36 (s, 2H, 2-NH2), 4.84 (d, 2H, J=7.5Hz, CH2NO2), 3.79 (s, 3H, CH3), 3.61 (m, 1H, 5-CHCH2CH2), 2.62, 2.48 (2m, 2H, 5-CHCH2CH2), 2.00, 1.84 (2m, 2H, 5-CHCH2CH2). 13C NMR (75 MHz, DMSO-d6) δ: 166.9, 164.2, 161.6, 148.7, 129.9, 129.2, 127.9, 83.3, 77.6, 52.6, 34.7, 33.7, 31.6. HRMS: Calcd for C15H19N7O4: 361.1499; found: 361.1506.
6-[2-(2,4-Diamino-7H-pyrrolo[2,3-d]pyrimidin-5-yl)ethyl]nicotinic acid (9b). Prepared from 8b (0.25 g, 0.69 mmol) as described for the preparation of 9a, yielded 9b (0.20 g, 97%) as a gray solid, mp > 300 °C. MS (ESI): m/z 299 [M+H]+. 1H NMR (300 MHz, DMSO-d6) δ: 11.41 (s, 1H, 7-H), 8.64 (d, 1H, J=2.4Hz, pyridine 2-H), 7.80 (dd, 1H, J=2.4, 8.4Hz, pyridine 4-H), 7.73 (br s, 2H, 4-NH2), 7.32 (d, 1H, J=8.4Hz, pyridine 5-H), 7.06 (s, 2H, 2-NH2), 6.59 (s, 1H, 6-H), 2.98 (t, 2H, J=7.2Hz, 5-CH2CH2), 2.90 (t, 2H, J=7.2Hz, 5-CH2CH2). 13C NMR (75 MHz, DMSO-d6) δ: 168.0, 154.4, 152.6, 150.1, 147.4, 129.9, 129.4, 129.1, 118.1, 116.5, 94.5, 35.9, 27.3. HRMS: Calcd for C14H14N6O2: 298.1178; found: 298.1175.
N-{6-[2-(2,4-Diamino-7H-pyrrolo[2,3-d]pyrimidin-5-yl)ethyl]nicotinoyl}-L-glutamic acid diethyl ester (10b). The acid 9b (0.22 g, 0.74 mmol) was condensed with diethyl L-glutamate hydrochloride as described for the preparation of 10a to give 10b (0.33 g, 93%) as a light yellow solid, mp 102~104 °C. MS (ESI): m/z 484 [M+H]+. 1H NMR (300 MHz, DMSO-d6) δ: 11.10 (s, 1H, 7-H), 8.69(m, 2H, CONH, pyridine 2-H), 7.86 (dd, 1H, J=2.4, 8.4Hz, pyridine 4-H), 7.34 (d, 1H, J=8.4Hz, pyridine 5-H), 7.22 (br s, 2H, 4-NH2), 6.54 (br s, 3H, 6-H, 2-NH2), 4.42 (m, 1H, glutamate α-CH), 4.10, 4.04 (2q, 4H, J=6.9Hz, 2CH2CH3), 3.03 (m, 2H, 5-CH2CH2), 2.92 (m, 2H, 5-CH2CH2), 2.43 (t, 2H, J=7.5Hz, glutamate γ-CH2), 2.05 (m, 2H, glutamate β-CH2), 1.17 (m, 6H, 2CH2CH3). 13C NMR (75 MHz, DMSO-d6) δ: 172.9, 172.5, 167.3, 155.7, 154.8, 151.5, 150.7, 146.1, 131.9, 129.1, 128.0, 117.3, 115.8, 95.0, 61.2, 60.6, 52.7, 36.0, 30.9, 27.7, 26.4, 14.8. HRMS: Calcd for C23H29N7O5: 483.2230; found: 483.2234.
N-{6-[2-(2,4-Diamino-7H-pyrrolo[2,3-d]pyrimidin-5-yl)ethyl]nicotinoyl}-L-glutamic acid (2b). Hydrolysis of 10b (0.10 g, 0.2 mmol) as described above for the preparation of 2a, gave 2b (58 mg, 68%) as a light yellow solid, mp 253~255 °C. MS (ESI): m/z 428 [M+H]+. 1H NMR (300 MHz, DMSO-d6) δ: 10.46 (s, 1H, 7-H), 8.60 (d, 1H, J=2.4Hz, pyridine 2-H), 8.36 (d, 2H, J=7.5Hz, CONH), 7.77 (dd, 1H, J=2.4, 8.4Hz, pyridine 4-H), 7.32 (d, 1H, J=8.4Hz, pyridine 5-H), 6.38 (s, 1H, 6-H), 6.20 (s, 2H, 4-NH2), 5.54(br s, 2H, 2- NH2), 4.35 (m, 1H, glutamate α-CH), 3.30~4.90 (br s, 2H, 2COOH), 2.95 (m, 4H, 5-CH2CH2), 2.32 (t, 2H, J=6.9Hz, glutamate γ-CH2), 2.00 (m, 2H, glutamate β-CH2). 13C NMR (75 MHz, DMSO-d6) δ: 175.0, 174.5, 166.7, 159.5, 158.3, 154.4, 146.1, 132.3, 129.0, 127.9, 115.5, 114.5, 96.0, 53.0, 36.5, 31.8, 28.3, 27.3. HRMS: Calcd for C19H21N7O5: 427.1604; found: 427.1612.
Measurement of cytotoxicity (in vitro cell culture screening).
Cell culture: The target compounds 2a and 2b were evaluated for their cytotoxic activity against L1210 and A549 cells respectively.4
Methods10: The cell viability was estimated by the MTT method. Cells were plated into 96-well tissue culture plates at a density of 4~5×103 cells/well and incubated for 24 h under hydroxic conditions. They were treated with the target compounds and incubated for another 48 h. Twenty microliters MTT (5 μg/mL in PBS) was added to the culture medium. After 4 h, the blue reaction product was yielded. The medium was removed and the residual product was dissolved with DMSO (100 μL). The absorbance was measured with Thermo Multiskan MK3 at 570nm.
ACKNOWLEDGEMENTS
We would thank to the funds of Shanghai Science and Technology Committee for the financial support (042319231). We are grateful to School of Chemistry and Chemical Engineering, Shanghai Jiaotong University, Shanghai Institute of Planned Parenthood Research and the Instrumental Analysis Center of East China University of Science and Technology for providing all the spectral data.
References
1. C. Shih, V. J. Chen, L. S. Gossett, and S. B. Gates, Cancer Res., 1997, 57, 1116; L. G. Mendelsohn, C. Shih, V. J. Chen, and L. L. Habeck, Semin. Oncol., 1999, 26, 42.
2. A. Gangjee, H. D. Jain, J. J. McGuire, and R. L. Kisliuk, J. Med. Chem., 2004, 47, 6730; CrossRef A. Gangjee, Y. B. Qiu, W. Li, and R. L. Kisliuk, J. Med. Chem., 2008, 51, 5789. CrossRef
3. G. J. Zhu, Z. L. Liu, Y. Xu, and Z. M. Mao, Heterocycles, 2008, 75, 1631. CrossRef
4. J. A. Montaomery, J. R. Piper, R. D. Elliott, and C. Temple, J. Med. Chem., 1979, 22, 862; CrossRef P. R. Marsham, L. R. Hughes, A. L. Jackman, and A. J. Hayter, J. Med. Chem., 1991, 34, 1594; CrossRef P. R. Marsham, A. L. Jackman A. J. Hayter, and M. R. Daw, J. Med. Chem., 1991, 34, 2209; CrossRef J. R. Piper, J. I. Degraw, W. T. Colwell, and C. A. Johnson, J. Med. Chem., 1997, 40, 377. CrossRef
5. E. C. Taylor and B. Liu, Tetrahedron Lett., 1999, 40, 4023; CrossRef E. C. Taylor and B. Liu, J. Org. Chem., 2003, 68, 9938. CrossRef
6. A. D. Broom, J. L. Shim and G. L. Anderson, J. Org. Chem., 1976, 41, 1095; CrossRef G. L. Anderson, J. Heterocycl. Chem., 1985, 22, 1469; CrossRef M. J. Koen, J. E. Gready, J. Org. Chem., 1993, 58, 1104; CrossRef E. C. Taylor, J. E. Dowling, T. Schrader, and B. Bhatia, Tetrahedron, 1998, 54, 9507. CrossRef
7. E. C. Taylor, G. Paul, and M. Patel, J. Org. Chem., 1992, 57, 3218. CrossRef
8. J. Melton and J. E. Mcmurry, J. Org. Chem., 1975, 40, 2138. CrossRef
9. E. C. Taylor and Z. M. Mao, J. Org. Chem., 1996, 61, 7973. CrossRef
10. F. M. Freimoser, G. A. Jakob, M. Aebi, and U. Tuor, Appl. Environ. Microbiol., 1999, 65, 3727