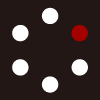
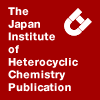
HETEROCYCLES
An International Journal for Reviews and Communications in Heterocyclic ChemistryWeb Edition ISSN: 1881-0942
Published online by The Japan Institute of Heterocyclic Chemistry
e-Journal
Full Text HTML
Received, 17th April, 2009, Accepted, 28th May, 2009, Published online, 29th May, 2009.
DOI: 10.3987/COM-09-11738
■ Liver X Receptor (LXR) Modulators with Dibenz[b,f][1,4]oxazepin-11-one, (Z)-Dibenz[b,f]azocin-6-one, and 11,12-Dihydrodibenz[b,f]azocin-6-one Skeletons
Atsushi Aoyama, Hiroshi Aoyama, Makoto Makishima, Yuichi Hashimoto, and Hiroyuki Miyachi*
Graduate School of Medicine, Dentistry and Pharmaceutical Sciences, Okayama University, 1-1-1 Tsushima-naka, Okayama 700-8530, Japan
Abstract
Conformationally restricted heterocyclic analogs of carba-T0901317 with dibenz[b,f][1,4]oxazepin-11-one, 11,12-dihydrodibenz[b,f]azocin-6-one, and (Z)-dibenz[b,f]-azocin-6-one skeletons were prepared as candidate for liver X receptor (LXR) agonists. In vitro transactivation assay revealed that the activity depends on the nature of the linking group.INTRODUCTION
Liver X receptors (LXR) are members of the nuclear receptor superfamily and are involved in the regulation of cholesterol, lipid, and glucose metabolism.1, 2 LXRs are ligand-dependent transcription factors which bind to DNA as heterodimers with retinoid X receptor (RXR). There are two subtypes, i.e., LXRα and LXRβ, which have characteristic distribution patterns. LXRα is mostly expressed in liver, adipose tissue and macrophages, while LXRβ is ubiquitously distributed. The endogenous ligands of LXRs are reported to be metabolites of cholesterol (oxysterols), as exemplified by 22(R)-hydroxycholesterol (1) and 24(S),25-epoxycholesterol (2).3 In macrophages, liver, and intestine, activation of LXRs induces the expression of genes involved in cholesterol homeostasis.4 It may be possible to prevent or even reverse atherosclerosis by modulating the expression or activity of LXRs, which are therefore an attractive potential therapeutic target. However, the beneficial pharmacological effects of LXR agonists are compromised by the LXR-mediated increase in the expression of genes that affect hepatic lipogenesis, including sterol regulatory element binding protein 1c (SREBP-1c) and fatty acid synthase (FAS). Indeed, chronic treatment of rodents with pan-full-LXR agonists, such as T0901317 (3), leads to the accumulation of hepatic triglycerides and causes steatosis.4 Recent studies indicated a distinct role of the LXRα subtype in lipogenesis, because LXRα knockout mice are unable to regulate lipid balance when they are given a high fat diet, and develop steatosis. On the other hand, LXRβ knockout mice can manage a high fat diet as effectively as can wild-type mice.5, 6 Therefore, there is great interest in subtype-selective LXR agonists, especially LXRβ-selective agonists and/or LXR modulators that have beneficial effects on cholesterol transport and inflammation, but do not induce hepatic lipogenesis.
We have studied the design and synthesis of ligands of various nuclear receptors, such as peroxisome proliferator-activated receptor (PPAR) and farnesoid X receptor (FXR), and we recently expanded our focus to include another metabolic nuclear receptor, LXR.7, 8 Previously, we have demonstrated that 5-substituted phenanthridin-6-one derivatives, which are conformationally restricted heterocyclic analogues of carba-T0901317,9 exhibit no LXR-agonistic activity, but show LXR-antagonistic activity.10 This prompted us to speculate that the nature of the LXR-modulating activity elicited by the conformationally restricted carba-T0901317 derivatives depends on the three-dimensional geometry of their two phenyl ring moieties. Here, we would like to report the results of further structural modification of carba-T0901317.
RESULTS AND DISCUSSION
In order to investigate the effects of the different degrees of conformational restriction, we planned to synthesize dibenz[b,f][1,4]oxazepin-11-one 5, 11,12-dihydrodibenz[b,f]azocin-6-one 6, and (Z)-dibenz[b,f]azocin-6-one 7 derivatives with one-atom and two-atom linkers.
We planned to synthesize compound 5 by the intramolecular aromatic nucleophilic substitution reaction of a substituted phenol derivative as the key step.11 As shown in Scheme 1, Friedel-Crafts type reaction of 2-methoxyaniline with hexafluoroacetone hydrate proceeded at the para-position of the amino group to afford 1,1,1,3,3,3-hexafluoropropan-2-ol derivative 8, although the yield (41%) was moderate. Compound 8 was amidated with 2-fluorobenzoyl chloride in the presence of triethylamine (95% yield), and subsequent demethylation of the methoxy group with BBr3 afforded quantitatively the key intermediate phenol derivative 10. The intramolecular aromatic nucleophilic substitution reaction of compound 10 proceeded smoothly by use of K2CO3 as the base in DMF to afford the dibenz[b,f][1,4]oxazepin-11-one skeleton in 81% yield. N-Ethylation of compound 11 afforded the target dibenz[b,f][1,4]oxazepin-11-one derivative 5 in 42% yield.12
The preparation of 11,12-dihydrodibenz[b,f]azocin-6-one derivative 6 is summarized in Scheme 2. We planned to construct this ring system by the intramolecular lactamation reaction of an amino-substituted benzoic acid derivative as the key step.13 Wittig reaction of 2-nitrobenzyltriphenylphosphonium bromide (12), which was prepared from 2-nitrobenzyl bromide and triphenylphosphine in toluene (93% yield), and ethyl 2-formylbenzoate (13), which was prepared by the ethyl esterification of 2-formylbenzoic acid in 98% yield, in the presence of sodium ethoxide in dichlomethane afforded stilbene derivative 14 in 60% yield as a mixture of stereoisomers. Compound 14 was hydrogenated (15), friedel-crafts type alkylated (16), hydrolysed with NaOH afforded the precursor acid derivative 17. The intramolecular lactamation of 17 was proceeded EDCI-HOBt-DIPEA system in CH2Cl2 afforded 11,12-dihydrodibenz[b,f]azocin-6-one derivative 18 although the yield was poor (20% yield). In the absence of HOBt or with the use of carbodiimidazole as the coupling reagent, we could obtain the lactam 18 in a trace amount. N-Ethylation of compound 18 as described for compound 11 afforded the target 11,12-dihydrodibenz[b,f]azocin-6- one derivative 6 in 63% yield.14
The preparation of (Z)-dibenz[b,f]azocin-6-one derivativ e 7 is summarized in scheme 3. We planned to construct this ring system by means of intramolecular Stille coupling reaction of a vinyl-substituted aromatic iodide derivative as the key step.15 The Stille coupling of 2-(4-amino-3-bromophenyl)-1,1,1,3,3,3-hexafluoropropan-2-ol with tributyl(vinyl)tin in the presence of Pd(PPh3)4 in toluene afforded 2-vinylaniline derivative 19 in 75% yield. Compound 19 was amidated with 2-iodobenzoyl chloride in the presence of triethylamine to afford compound 20 in 73% yield. The hydroxyl group of compound 20 was protected with 2-methoxyethoxymethyl chloride in the presence of DBU as a base in DMF to afford the MEM- derivative 21 in 81% yield. The N-ethylation of compound 21 as described for compound 11 afforded the N-ethyl derivative 22 in 56% yield. The intramolecular coupling reaction of compound 22 proceeded smoothly with the use of Pd(OAc)2 and PPh3 in the presence of AcONa as the base in DMF to afford the (Z)-dibenz[b,f]azocin-6-one skeleton in quantitative yield. The MEM group was deprotected with TiCl4-DCM to afford the target (Z)-dibenz[b,f]azocin-6-one derivative 7 in 63% yield.16
The values of in vitro transactivation activity of the compounds in the present series are summarized in Table 1, along with that of carba-T0901317 analogue 4 as the positive control, and that of our previously reported phenanthridin-6-one LXR antagonist. Compound 4 exhibited potent pan LXR-agonistic activities, i.e., its EC50 values for LXRα and LXRβ are 1.80 and 0.25 μM, respectively.
As previously described, the directly linked carba-T0901317 analogue, the phenanthridin-6-one derivative, did not exhibit LXR-agonistic activity, but did exhibit LXR-antagonistic activity. However, the compound with the one-atom (oxygen) elongation linker, dibenz[b,f][1,4]oxazepin-11-one derivative 5, exhibited LXR-agonistic activity. It is of interest to note that it exhibited a decreased micromolar order EC50 value for LXRβ as compared to compound 4, but still exhibited full agonistic activity (the intrinsic activity of 5 is 73% of that of T0901317). In the case of LXRα, it exhibited decreased potency and efficacy, showing weak partial agonistic activity (32% of the activity of 10 μM T0901317).
(Z)-Dibenz[b,f]azocin-6-one derivative 7, with the ethylidene linker (two-atom elongation linker), also exhibited LXR-agonistic activity. It exhibited a more potent, sub-micromolar order EC50 value for LXRβ as compared to 5 with the one-atom elongation linker, and showed full agonistic activity (the intrinsic activity of 7 is 75% of that of T0901317). It also exhibited weak partial agonistic activity towards LXRα (67% of the activity of 10 μM T0901317). The degree of partial agonism of compound 7 is greater than that of compound 5.
In contrast to the compounds with the oxygen linker and the ethylidene linker, the compound with the ethylene linker (two-atom elongation linker), 11,12-dihydro-dibenz[b,f]azocin-6-one derivative 6, exhibited full LXR-agonistic activity towards both subtypes. In the case of LXRβ, it exhibited comparable EC50 value to the positive control, carba-T analogue 4. In the case of LXRα, it also exhibited agonistic activity comparable to or somewhat superior to that of carba-T analogue 4. These structure-activity relationship results clearly indicated the importance of the nature and/or length of the bridging linker for both potency and efficacy in the present series of compounds.
In summary, we have developed LXR ligands with various degrees of intrinsic activity. We are planning to conduct an X-ray crystallographic analysis in combination with molecular modeling studies to understand the reason why these conformationally restricted carba-T analogues exhibit characteristic activities towards LXRs. We are also planning an in vivo pharmacological evaluation of a representative compound as a candidate antimetabolic syndrome agent.
ACKNOWLEDGEMENTS
The work described in this paper was partially supported by a Grant-in-aid for Scientific Research from The Ministry of Education, Culture, Sports, Science and Technology, Japan.
References
1. P. T. Meinke, H. B. Wood, and J. W. Szewczyk, Ann. Rep. Med. Chem., 2006, 41, 99. CrossRef
2. N. Mitro, P. A. Mak, L. Vatgas, C. Godio, E. Hampton, V. Molteni, A. Kreusch, and E. Saez, Nature, 2007, 445, 219. CrossRef
3. B. A. Janowski, P. J. Willy, T. R. Devi, J. R. Falck, and D. J. Mangelsdorf, Nature,1996, 383, 728. CrossRef
4. J. R. Schultz, H. Tu, A. Luk, J. J. Repa, J. C. Medina, L. Li, S. Schwendner, S. Wang, M. Thoolen, D. J. Mangelsdorf, K. D. Lustig, and B. Shan, Genes Dev., 2000, 14, 2831. CrossRef
5. S. Alberti, G. Schuster, P. Parini, D. Feltkamp D, U. Diczfalusy, M. Rudling, B. Angelin, I. Björkhem, S. Pettersson, and J. A. Gustafsson, J. Clin. Invest., 2001, 107, 565. CrossRef
6. E. M. Quinet, D. A. Savio, A. R. Halpern, L. Chen, G. U. Schuster, J. A. Gustafsson, M. D. Basso, and P. Nambi, Mol. Pharmacol., 2006, 70, 1340. CrossRef
7. T. Noguchi-Yachide, A. Aoyama, M. Makishima, H. Miyachi, and Y. Hashimoto, Bioorg. Med. Chem. Lett., 2007, 17, 3957. CrossRef
8. T. Noguchi-Yachide, H. Miyachi, A. Aoyama, M. Makishima, H. Aoyama, and Y. Hashimoto, Chem. Pharm. Bull., 2007, 55, 1750. CrossRef
9. L. Li, J. Liu, L. Zhu, S. Cutler, H. Hasegawa, B. Shan, and J. C. Medina, Bioorg. Med. Chem. Lett., 2006, 16, 1638. CrossRef
10. A. Aoyama, H. Aoyama, K. Dodo, M. Makishima, Y. Hashimoto, and H. Miyachi, Heterocycles, 2008, 76, 137. CrossRef
11. Rogier A. Smits, Herman D. Lim, Bart Stegink, Remko A. Bakker, Iwan J. P. de Esch, and Rob Leurs, J. Med. Chem., 2006, 49, 4512. CrossRef
12. 10-Ethyl-7-(1,1,1,3,3,3-hexafluoro-2-hydroxypropan-2-yl)-dibenz[b,f][1,4]oxazepin-11(10H)-one (5). mp 235—237 ℃; 1H NMR (500 MHz, d6–DMSO) δ 8.95 (s, 1H), 7.72 (dd, J = 7.8, 2.0 Hz, 1H), 7.68 (d, J = 8.3 Hz, 1H), 7.64 (d, J = 2.0 Hz, 1H), 7.58 (dd, J = 8.3, 7.3 Hz, 1H), 7.54 (d, J = 7.8 Hz, 1H), 7.42 (d, J = 7.8 Hz, 1H), 7.31 (dd, J = 7.8, 7.3 Hz, 1H), 4.09 (q, J = 6.8 Hz, 2H), 1.25 (t, J = 6.8 Hz, 3H); HRMS (FAB) calcd for C18H14F6NO3 406.0878; found: 406.0858 (M+H)+.
13. F. Yokokawa and T. Shioiri, J. Org. Chem., 1998, 63, 8638. CrossRef
14. 5-Ethyl-2-(1,1,1,3,3,3-hexafluoro-2-hydroxypropan-2-yl)-11,12-dihydrodibenz[b,f]azocin-6-one (6). mp 255—257 ℃; 1H NMR (500 MHz, CDCL3) δ 7.43–7.38 (m, 2H), 7.15 (dd, J = 7.3, 1.2 Hz, 1H), 7.11–7.02 (m, 3H), 6.88 (dd, J = 7.3, 1.2 Hz, 1H), 4.34–4.26 (m, 1H), 4.02 (s, 1H), 3.65–3.57 (m, 1H), 3.46–3.39 (m, 1H), 3.36–3.28 (m, 1H), 2.98–2.89 (m, 2H), 1.24 (t, J = 7.3 Hz, 3H); HRMS (FAB) calcd for C20H18F6NO2 418.1242; found: 418.1220 (M+H)+.
15. J. K. Stille, Angew. Chem., Int. Ed. Engl., 1986, 25, 508. CrossRef
16. (Z)-5-Ethyl-2-(1,1,1,3,3,3-hexafluoro-2-hydroxypropan-2-yl)-dibenz[b,f]azocin-6-one (7). mp 260—262 ℃; 1H NMR (500 MHz, CDCl3) δ 7.90 (dd, J = 7.9, 1.8 Hz, 1H), 7.63–7.60 (m, 2H), 7.45 (ddd, J = 7.9, 7.9, 1.8 Hz, 1H), 7.40–7.36 (m, 2H), 7.25 (dd, J = 7.9, 1.8 Hz, 1H), 5.52 (s, 1H), 5.48 (s, 1H), 4.40–4.32 (m, 1H), 3.98–3.90 (m, 1H), 3.61 (s, 1H), 1.31 (t, J = 7.3 Hz, 3H) ; HRMS (FAB) calcd for C20H16F6NO2 416.1085; found: 416.1060 (M+H)+.