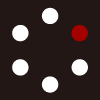
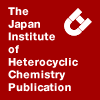
HETEROCYCLES
An International Journal for Reviews and Communications in Heterocyclic ChemistryWeb Edition ISSN: 1881-0942
Published online by The Japan Institute of Heterocyclic Chemistry
e-Journal
Full Text HTML
Received, 5th May, 2009, Accepted, 1st July, 2009, Published online, 2nd July, 2009.
DOI: 10.3987/COM-09-11746
■ Synthesis of Halogenated 4H-Pyrido[1,2-a]pyrimidin-4-ones
Annamária Molnár, Ferenc Faigl, Benjamin Podányi, Zoltán Finta, László Balázs, and István Hermecz*
R & D Directorate Preclinical Development, CHINOIN Pharmaceutical and Chemical Works Ltd., P. O. Box 110, H-1325 Budapest, Hungary
Abstract
Halogenated 4H-pyrido[1,2-a]pyrimidin-4-one were synthesized by thermal cyclization and decarboxylation of isopropylidene (2-pyridylamino)methylenemalonates, prepared from 2-aminopyridines and isopropylidene methoxymethylenemalonate formed in situ. Instead of 4H-pyrido[1,2-a]pyrimidin-4-ones, the 6-chloro and 6-bromo derivatives afforded mixtures of 7-halo-1,4-dihydro-1,8-naphthyridin-4-ones and 1-(6-halo-2-pyridyl)-3-[(6-halo-2-pyridylamino)methylene]-1,2,3,4-tetrahydropyridine-2,4-diones. The latters formed from N-(2-pyridyl)iminoketenes, the common intermediates of 4H-pyrido[1,2-a]pyrimidin-4-one and 1,8-naphthyridin-4-ones, via a “head-to-tail” [4+2] cycloaddition. 3-Halo-4H-pyrido[1,2-a]pyrimidin-4-ones were obtained from 4H-pyrido[1,2-a]pyrimidin-4-one with N-halosuccinimides. The structures of the new compounds were characterized by means of 1H NMR and 13C NMR examinations.
INTODUCTION
The pyrido[1,2-a]pyrimidine skeleton is a privileged scaffold for facile access to “drug-like” small molecules,2 which fulfill the requirements of the rule-of-five.3 In particular, 4H-pyrido[1,2-a]pyrimidin-4-ones have attracted the attention of pharmaceutical community, as they display diverse biological activities (Figure 1). The best-known 4H-pyrido[1,2-a]pyrimidin-4-one is the atypical neuroleptic risperidone 1, which was one of the drugs most widely prescribed worldwide in 2007.4 Its active metabolite, the antipsychotic paliperidone 2, was registered by the FDA in 2007 for the treatment of bipolar disorders.5 The other outstanding members include the anti-allergic pemirolast 3,6 the non-narcotic analgesic rimazolium 4,7 an anti-ulcer agent 5,8 the tranquillizer pirenperone 6,9 the anti-allergic ramastine 7,10 the antihypertensive seganserin 811 and the antidepressant lusaperidone 9.12
Transition metal-catalyzed carbon-carbon bond formation is a fundamental reaction for the functionalization of aromatic and heteroaromatic ring systems.13 To date, no systematic investigation has been reported on 4H-pyrido[1,2-a]pyrimidin-4-ones. Only a few published examples of transition metal-catalyzed carbon-carbon bond formation are to be found for 4H-pyrido[1,2-a]pyrimidin-4-ones.14
In the present paper, we report on the synthesis of different halogen derivatives of 4H-pyrido[1,2-a]pyrimidin-4-one, which are very convenient substrates for cross-couplings (e.g. the Suzuki-Miyaura, Heck, Sonogashira, and Buchwald-Hartwig reactions).
RESULTS AND DISCUSSION
For the synthesis of 4H-pyrido[1,2-a]pyrimidin-4-ones 17, Meldrum’s acid15 10 was selected as starting material instead of dimethyl malonate, because 10 is a stronger CH acid (pKa = 7.32 ± 0.01 in DMSO16) and therefore more reactive than dimethyl malonate (pKa = 15.87 ± 0.03 in DMSO16). Another advantage is that 17 can be obtained from aminomethylenemalonates (14) in three reaction steps: cyclization, ester hydrolysis and then decarboxylation,17 whereas aminomethylenemalonates (13) give 4H-pyrido[1,2-a]pyrimidin-4-ones (17) directly in a one-pot reaction under thermal conditions18 where decarboxylation and cyclization occur during heating, at around 240-260 oC. The alternative reaction sequences are depicted in Scheme 1. Carboxylic acid derivatives of nitrogen bridgehead ring systems (e.g. 15, R = H) could be obtained from isopropylidene (2-hetarylamino)methylenemalonates even under acidic conditions (e.g. in a mixture of POCl3 and PPA at 135-140 oC,19a and in PPA19b).
The reaction between Meldrum’s acid 10 and trimethyl orthoformate smoothly yielded isopropylidene methoxymethylenemalonate (11). After the reaction mixture had been heated for 4 h on an oil bath and then evaporated to dryness in vacuo, the residue was left to react with the appropriate 2-aminopyridine in EtOH at ambient temperature over night. The precipitated aminomethylenemalonate (13) was filtered off from the cooled reaction mixture and used directly in the cyclization step without further purification (yields are shown in Table 1).
The addition of (6-unsubstituted-2-pyridyl)aminomethylenemalonates (13a-k) to preheated Ph2O at 260 oC afforded 4H-pyrido[1,2-a]pyrimidin-4-ones (17) in good yields after dilution of the cold reaction mixture with hexane and extraction with aqueous 2 N HCl, followed by adjustment of the pH of the aqueous extract to 8 with 40% NaOH solution (see Table 1). The precipitated 4H-pyrido[1,2-a]pyrimidin-4-ones were filtered off and recrystallized from EtOH. Our work-up protocol provided 15-30% better yields for 17f, 17i and 17k than in the earlier methods20,21 (50%, 62% and 44%, respectively), where Ph2O was diluted with pentane or hexane and precipitated 4H-pyrido[1,2-a]pyrimidin-4-ones were filtered off. In the case of 17k, no mp was reported previously.21
In contrast with what was reported by Ye et al.,23 who have obtained 4-oxo-4H-pyrido[1,2-a]pyrimidine-3-carboxylic acids (19) when 18 was heated under reflux in EtOH, we did not observed the formation of any 4H-pyrido[1,2-a]pyrimidine-3-carboxylic acid (19, R = H) from 13h under similar conditions, even after a longer reaction time (8 h) (Scheme 2).
The thermal cyclization of 3-(6-substituted-2-pyridyl)acrylates (20) gave 6-substituted-4H-pyrido- [1,2-a]pyrimidin-4-ones (21) or 7-substituted-1,4-dihydro-1,8-naphthyridin-4-ones (22), or their mixture, depending on the nature of the 2-substituent in the acrylates (R3) and on the reaction conditions.24 (Scheme 3) 6-Substituted-4H-pyrido[1,2-a]pyrimidin-4-ones (21) were transformed into the thermodynamically more stable 7-substituted-1,4-dihydro-1,8-naphthyridin-4-ones (22) by heating at higher temperature or for a longer period.25
De Silva et al.26 obtained 5,7-dimethyl-1,4-dihydro-1,8-naphthyridin-4-one (24) by refluxing isopropylidene [N-(4,6-dimethyl-2-pyridyl)aminomethylene]malonate (23) in Ph2O, but 4,6-dimethyl-4H-pyrido[1,2-a]pyrimidin-4-one (25) was the product when the ring closure was carried out in Dowtherm A at a lower temperature, ~240 oC.18 (Scheme 4).
From the 6-chloro and 6-bromo derivatives of aminomethylenemalonates (13l, m) in boiling Ph2O we expected to obtain 7-halo-1,4-dihydro-1,8-naphthyridin-4-ones (27, X = Cl, Br), as the Cl and Br atoms exhibit similar bulkiness27 to that of the Me group. From the reaction mixtures besides the expected 1,8-naphthyridines 27, we could isolated by-products, too (Scheme 5). In both cases LC-MS investigations have indicated a dimer formation with brutto formula C16H10N4O2X2. (At the thermal cyclization of 13a dimer formation could not be detetected by LC-MS investigations.) Naphthyridines 27 and dimers 29 could be separated by fractional crystallization from EtOH. Dimers exhibited lower solubility, than naphthyridines. According to our knowledge, these are the first cases when dimeric products were detected and isolated in the case of the thermal cyclization of (6-substituted 2-pyridylamino)methylenemalonates.
Dimers 29 would be formed in a “head-to-tail” [4+2] cyclodimerization of the iminoketene 26, which would probably take place in a pseudopericyclic manner, initiated by the attack of an imino lone pair on the carbonyl carbon of a second molecule of iminoketene (Scheme 6). The initial dimer (28) thus formed would tautomerizes by 1,3 hydrogen shift to the isolated product 29. A similar dimer formation was earlier described by Wentrup et al. in the case of 2-pyridylketene.28 1H and 13C NMR data are consistent with the dimer structure 29, and have indicated that the products are present as a 1:2 E-Z isomeric mixtures in DMSO-d6 (see Figure 2).
3-Chloro-, 3-bromo- and 3-iodo-4H-pyrido[1,2-a]pyrimidin-4-ones (30) were obtained from 17a in 76-86% yields by reaction with the respective N-halosuccinimide in CCl4 (Scheme 7).
CONCLUSION
In conclusion, a productive “one-pot” synthesis was extended for the preparation of halogenated
4H-pyrido[1,2-a]pyrimidin-4-one (17b-k) from Meldrum’s acid, trimethyl orthoformate and the appropriate 2-aminopyridine via methoxymethylenemalonates (13). From the 6-chloro and 6-bromo derivatives 13m, l a mixture of 7-halo-1,4-dihydro-1,8-naphthyridin-4-ones (27) and, in the first case, tetrahydropyridine-2,4-diones 29 were obtained. The latters formed in a “head-to-tail” [4+2] cyclodimerization from the common intermediates 26 of 4H-pyrido[1,2-a]pyrimidin-4-ones and 1,8-naphthyridin-4-ones. The 3-halo derivatives 30 were obtained from 4H-pyrido[1,2-a]- pyrimidin-4-one (17a) with N-halosuccinimides. The structures of the new compounds were characterized by means of 1H and 13C NMR spectroscopy.
EXPERIMENTAL
Melting points are uncorrected and yields were not maximized 1H and 13C NMR spectra were recorded in DMSO-d6 on a Bruker DRX instrument at 400 and 100 MHz, respectively). Coupling constants are reported in Hz and chemical shifts in ppm (δ, ppm) downfield from TMS, which was used as internal standard. The following abbreviations are used: singlet (s), doublet (d), triplet (t), broad (br). LC-MS investigations were carried out by an Alliance 2695 HPLC system (Waters Co.) equipped with a quaternary pump, an auto-sampler, Waters 2996 PDA detector and a Waters ZQ-2000 single quadruple mass spectrometer with ESCi source. Chromatographic separation was carried out at 30 °C on an Acquity UPLC BEH C18 column (2.1 × 50 mm, 1.7 μm). The mobile phases consisted of 0.05% CF3CO2H in H2O (A) and 0.03% CF3CO2H acid in MeCN (B) using a gradient elution of 5–5% (v/v) B at 0–0.5 min; 5–95% B at 0.5–4.5 min; 95–95% B at 4.5–6.0 min. The flow rate was 0.5 mL/min, and the injection volume was 0.5 μL.
Synthesis of Isopropylidene (2-Pyridylamino)methylenemalonate (13). General Method. A 1:2 mixture of Meldrum’s acid (17.6 g, 0.12 mol) and trimethyl orthoformate (30 mL, 0.25 mol) was heated under reflux for 4 h, and the reaction mixture was then evaporated to dryness at 100 mbar. The residue 11 was dissolved in EtOH (200 mL) and a 2-aminopyridine (0.1 mol) was added, and the reaction mixture was stirred at ambient temperature overnight. The precipitated 13 was filtered off, washed with EtOH and used in cyclization reaction without further purification. Yields and mp are listed in Table 1, and the results of the elemental analysis are listed in Table 5.
Synthesis of 4H-Pyrido[1,2-a]pyrimidin-4-ones (17). General Method. Isopropylidene (2-pyridylamino)methylenemalonate (13) (20 g) was added to Ph2O (180 g) preheated to 260 oC. The reaction mixture was heated at 260 oC for 10 min, then quickly cooled to room temperature, diluted with n-hexane (200 mL) and extracted with 2 N HCl. The pH of the separated aqueous phase was adjusted to 8 with 40% aqueous NaOH, and the precipitated 4H-pyrido[1,2-a]pyrimidin-4-one (17) was filtered off, washed with water, and recrystallized from EtOH. Yields and mp are given in Table 1, and results of the elemental analysis are listed in Table 5.
Cyclization of Isopropylidene (6-halo-2-pyridylamino)methylenemalonates (27). 6-Halo derivative 13l, or m (2 g) was added to Ph2O (40 g) preheated at 260 oC, the reaction mixture was heated at 260 °C for ~2 min, then quickly cooled to room temperature and diluted with n-hexane (80 mL). The precipitated crystals were filtered off, and 27 and 29 were separated by fractional crystallization from EtOH.
7-Chloro-1,4-dihydro-1,8-naphthyridin-4-one (27a). Yield and mp are given in Table 2. 1H NMR δ 12.34 (brd, 1H, J = 5.9 Hz, NH), 8.44 (d, 1H, J = 8.3 Hz, 5-H), 7.94 (dd, 1H, J = 7.6 Hz and 5.9 Hz, 2-H), 7.44 (d, 1H, J = 8.3 Hz, 6-H), 6.12 (d, 1H, J = 7.6 Hz, 3-H); 13C NMR δ 111.0 (C-3), 119.5 (C-4a), 120.3 (C-5), 138.7 (C-6), 140.8 (C-7), 150.4 (C-8a), 152.9 (C-2), 177,1 (C-4). Anal. Calcd for C8H5ClN2O: C, 53.21; H, 2.79; N, 15.51. Found: C 53.34; H 2.63; N 15.70
7-Bromo-1,4-dihydro-1,8-naphthyridin-4-one (27b). Yield and mp are given in Table 2. Anal. Calcd for C8H5BrN2O: C, 42.70; H, 2.24; N 12.45. Found: C, 42.58; H, 2.31; N, 12,56.
1-(6-Chloro-2-pyridyl)-3-[(6-chloro-2-pyridylamino)methylene]-1,2,3,4-tetrahydropyridine-2,4-dione (29a). Yield 8%, its purity was cca 95%, mp > 300 °C. HRMS m/z: (ESI+) calcd for C16H10Cl2N4O2 Found: 361.0246.
1-(6-Bromo-2-pyridyl)-3-[(6-bromo-2-pyridylamino)methylene]-1,2,3,4-tetrahydropyridine-2,4-dione (29b). Yield cca 6%, its purity was cca 80%, mp > 300 °C. HRMS m/z: (ESI+) calcd for C16H10Br2N4O2 . Found: 448.9253.
Synthesis of 3-Halo-4H-pyrido[1,2-a]pyrimidin-4-ones (30). The respective N-halosuccinimide (0.11 mol) was added to a solution of 4H-pyrido[1,2-a]pyrimidin-4-one 17a (14.6 g, 0.1 mol) in CCl4 (200 mL), and the mixture was heated under reflux for 30 min, then filtered hot and cooled to ambient temperature, and the precipitated yellow crystals were filtered off and recrystallized from EtOH.
3-Chloro-4H-pyrido[1,2-a]pyrimidin-4-one (28a). Yield 86%, mp 159-160 °C. Anal. Calcd for C8H5ClN2O: C, 53.21; H, 2.79; N, 15.51. Found: C, 53.45; H, 2.86; N, 15.35.
3-Bromo-4H-pyrido[1,2-a]pyrimidin-4-one (28b). Yield 76%, mp 137-138 oC, lit.,29 mp 133-134 oC.
3-Iodo-4H-pyrido[1,2-a]pyrimidin-4-one (28c). Yield 86%, mp 142 °C. Anal. Calcd for C8H5IN2O: C, 35.32; H, 1.85; N, 10.30. Found: C, 35.40; H, 1.69; N, 10.42.
ACKNOWLEDGMENTS
We are grateful Dr. Durham David for the linguistic improvement of the manuscript. One of the authors (A.M.) expresses her thanks for support through a sanofi-aventis fellowship.
References
1. Nitrogen Bridgehead Compounds Part 94. Part 93: Á. Kiss, J. Kökösi, R. Rotter, and I. Hermecz, Tetrahedron, 2000, 56, 7987. CrossRef
2. I. Hermecz and Z. Mészáros, Med. Res. Rev., 1988, 8, 203. CrossRef
3. C. A. Lipinski, F. Lombardo, B. W. Dominy, and P. J. Feeney, Adv. Drug Deliv. Rev., 1997, 23, 3. CrossRef
4. (a) C. Fenton and L. J. Scott, CNS Drug, 2005, 19, 429; (b) idem., Pharmaceut. Exec., 2008, (5), 74.
5. (a) P. Revill, N. Serradell, and J. Bolós, Drug Fut., 2006, 31, 579; CrossRef (b) L. P. H. Yang and G. L. Plosker, CNS Drug, 2007, 21, 417.
6. D. G. Shulman, L. Amdahl, C. Washington, and A. Graves, Clin. Ther., 2003, 25, 1096. CrossRef
7. S. Fürst, K. Gyires, and J. Knoll, Arzneim. Forsch., 1988, 38, 552.
8. S. Matsutani and Y. Mizushima, Eur. Pat. Appl., 329126, 1989 (Chem. Abstr., 1990, 112, 98557).
9. R. L. Smith, R. J. Barrett, and E. J. Sanders-Bush, J. Pharmacol. Exp. Ther., 1995, 275, 1050.
10. F. Awouters, J. Vermeire, F. Smeyers, P. Vermote, R. van Beek, and C. J. E. Niemegeers, Drug. Dev. Res., 1986, 8, 95. CrossRef
11. A. Petterson, K. Gradin, T. Hedner, and B. Persson, Naunyn-Schiedeberg’s Arch. Pharmacol., 1985, 329, 394. CrossRef
12. L. E. J. Kennis, F. P. Bischoff, C. J. Mertens, C. J. Love, F. A. F. Van den Keybus, S. Pieters, M. Braeken, A. A. H. P. Megens, and J. E. Leysen, Bioorg. Med. Chem. Lett., 2002, 10, 71. CrossRef
13. (a) F. Alonso, I. P. Beletskaya, and M. Yus, Tetrahedron, 2008, 64, 3047; CrossRef (b) R. Chinchilla and C. Nájera, Chem. Rev., 2007, 107, 874; CrossRef (c) L. Yin and J. Liebscher, Chem. Rev., 2007, 107, 133; CrossRef (d) I. J. S. Fairlamb, Chem. Soc. Rev., 2007, 36, 1036; CrossRef (e) B. H. Yang and S. L. Buchwald, J. Organomet. Chem., 1999, 576, 125 (1999) and references cited therein. CrossRef
14. (a) K. Yoshida, K. Nakayama, Y. Yokomizo, M. Ohtsuka, M. Takemura, K. Hoshino, H. Kanda, K. Namba, H. Nitanai, J. Z. Zhang, V. J. Lee, and W. J. Watkins, Bioorg. Med. Chem., 2006, 14, 8506; CrossRef (b) K. Yoshida, K. Nakayama, N. Kuru, S. Kobayashi, M. Ohtsuka, M. Takemura, K. Hoshino, H. Kanda, J. Z. Zhang, V. J. Lee, and W. J. Watkins, Bioorg. Med. Chem., 2006, 14, 1993; CrossRef (c) Z. A. Knight, G. G. Chiang, P. J. Alaimo, D. M. Kenski, C. H. Ho, K. Coan, R. T. Abraham, and K. M. Shokat, Bioorg. Med. Chem., 2004, 12, 4749; CrossRef (d) A. M. Venkatesan, J. I. Levin, J. S. Baker, P. S. Chan, T. Bailey, and J. Coupet, Bioorg. Med. Chem. Lett., 1994, 4, 183; CrossRef (e) M. J. O’Mahony, P. J. West, S. D. Lindell, and J. A. Macritchie, Brit Patent 2,307,177, 1997 (Chem. Abstr., 1997, 127, 132147); (f) A. D. Robertson, S. Jackson, V. Kenche, C. Yaip, H. Parbaharan, and P. Thompson, PCT Int. Appl. WO 01/053,266, 2001 (Chem. Abstr., 2001, 135, 122509); (g) G. L. Bundy, F. L. Ciske, M. J. Genin, S. E. Heasley, S. D. Larsen, B. H. Lee, P. D. May, J. R. Palmer, M. E. Schnute, V. E. Vaillancourt, A. Thorarensen, A. J. Wolf, N. A. Wicnienski, and D. Wilhite, PCT Int. Appl. WO 02/04,444, 2002 (Chem. Abstr., 2002, 136, 118476); (h) H.-J. Zhou, A. McDonald, G. Bergnes, D. J. Morgans Jr., J. C. Chabala, S. D. Knigth, and D. Dhanak, PCT Int. Appl. WO 04/064,741, 2004 (Chem. Abstr., 2004, 141, 174179); (i) W. Wang, R. N. Constantine, L. M. Lagniton, S. Pecchi, M. T. Burger, and M. C. Desai, PCT Int. Appl. WO 04/113,335, 2004 (Chem. Abstr., 2005, 142, 93843); (j) W. Wang, R. N. Constantine, and L. M. Lagniton, U.S. Patent 02/28,002, 2002 (Chem. Abstr., 2005, 143, 387057); (k) E. D. Jones, J. A. V. Coates, D. I. Rhodes, J. J. Deadman, N. A. Degraff, L. J. Winfield, N. Thienthong, W. Issa, N. Choi, and K. Macfarlane, PCT Int. Appl. WO 08/077,188, 2008 (Chem. Abstr., 2008, 149, 128851); (l) S. Liu, J. Fu, R. Kamboj, Q. Jia, M. Wood, S. Chowdhury, and J. Sun, PCT Int. Appl. WO 08/097,991, 2008 (Chem. Abstr., 2008, 149, 268064); (m) A.-R. Li, M. G. Johnson, J. Liu, X. Chen, X. Du, J. T. Mihalic, J. Deignan, D. J. Gustin, J. Duquette, Z. Fu, L. Zhu, A. P. Marcus, P. Bergeron, L. R. McGee, J. Danao, B. Lemon, T. Carabeo, T. Sullivan, J. Ma, L. Tang, G. Tonn, T. L. Collins, and J. C. Medina, Bioorg. Med. Chem. Lett., 2008, 18, 688. CrossRef
15. (a) A. S. Ivanov, Chem. Soc. Rev., 2008, 37, 789; CrossRef (b) A. E.-A. M. Gaber and H. McNab, Synthesis, 2001, 2059; CrossRef (c) I. Hermecz, G. Keresztúri, and L.Vasvári-Debreczy, Adv. Heterocycl. Chem., 1992, 54, 108; and 252; (d) B.-C. Chen, Heterocycles, 1991, 32, 529; CrossRef (e) H. McNab, Chem. Soc. Rev., 1991, 7, 345. CrossRef
16. (a) E. M. Arnett and J. A. Harrelson Jr., J. Am. Chem Soc., 1987, 109, 809; CrossRef (b) X. Wang and K. N. Houk, J. Am. Chem Soc., 1988, 110, 1870. CrossRef
17. R. Adams and I. J. Pachter, J. Am. Chem Soc., 1952, 74, 5491. CrossRef
18. G. Y. Lesher, U.S. Patent 3,907,798, 1975 (Chem. Abstr., 1976, 84, 49967).
19. (a) I. Hermecz, Á. Horváth, L. Vasvári-Debreczy, and Z. Mészáros, Synthesis, 1984, 152; CrossRef (b) R. Westwood, P. Miller, I. R. Ager, and D. P. Kay, Eur. Pat. Appl. 86.723, 1983 (Chem. Abstr., 1984, 100, 6552).
20. I. Ravina, D. Zicane, M. Petrova, E. Gudriniece, and U. Kalejs, Chem. Heterocycl. Compd., 2002, 38, 836. CrossRef
21. W.-C. Lee, L. Sun, F. Shan, C. Chuaqui, M. Cornebise, T. W. Pontz, M. Carter, J. Singh, P. A. Boriaci-Sjodin, L. Ling, and R. C. Setter, PCT WO 04/072033, 2004 (Chem. Abstr., 2004, 141, 225504).
22. N. Katagiri, R. Niwa, and T. Kato, Heterocycles, 1983, 20, 597. CrossRef
23. F.-C Ye, B.-C. Chen, and X. Huang, Synthesis, 1989, 317. CrossRef
24. (a) G. R. Lappin, J. Am. Chem. Soc., 1948, 70, 3348; CrossRef (b) I. Hermecz, Z. Mészáros, L. Vasvári- Debreczy, Á. Horváth, G. Horváth, and M. Pongor-Csákvári, J. Chem. Soc., Perkin Trans. 1, 1977, 789. CrossRef
25. (a) Z. Mészáros and I. Hermecz, Tetrahedron Lett., 1975, 16, 1019; CrossRef (b) I. Hermecz, Z. Mészáros, K. Simon, L. Szabó, and Z. Pál, J. Chem. Soc., Perkin Trans. 1, 1984, 1795; CrossRef (c) I. Hermecz, L. Vasvári-Debreczy, and K. Simon, J. Chem. Soc., Perkin Trans. 2, 1988, 1287. CrossRef
26. L. E. da Silva, A. C. Joussef, R. J. Nunes, and K. N. de Oliveira, Synth. Commun., 2008, 38, 15. CrossRef
27. (a) A. Bondi, J. Phys. Chem., 1964, 68, 441; CrossRef (b) A. Verloop, W. Hoogenstraaten, and J. Tipker, in Drug Design; ed. by E. J. Ariëns; Academic Press: New York, 1976; Vol. 7, pp. 165-207.
28. A. Khun, C. Plüg, and C. Wentrup, J. Am. Chem. Soc., 2000, 122, 1945. CrossRef
29. R. Adams and I. J. Pachter, J. Am. Chem. Soc., 1954, 76, 1845 CrossRef