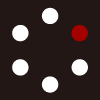
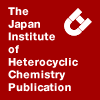
HETEROCYCLES
An International Journal for Reviews and Communications in Heterocyclic ChemistryWeb Edition ISSN: 1881-0942
Published online by The Japan Institute of Heterocyclic Chemistry
e-Journal
Full Text HTML
Received, 11th May, 2009, Accepted, 2nd June, 2009, Published online, 3rd June, 2009.
DOI: 10.3987/COM-09-11750
■ Synthesis of 2-Thioxo-2,3-dihydropyrido[3,2-e]-1,3-thiazin-4-ones by the Reaction of N-Alkyl-2-chloropyridine-3-carboxamides with Carbon Disulfide
Kazuhiro Kobayashi,* Toshihide Komatsu, and Hisatoshi Konishi
Department of Chemistry and Biotechnology, Graduate School of Engineering, Tottori University, 4-101 Koyama-minami, Tottori 680-8552, Japan
Abstract
A facile method for the preparation of 2-thioxo-2,3- dihydropyrido[3,2-e]-1,3-thiazin-4-ones by the reaction of N-alkyl-2- chloropyridine-3-carboxamides with carbon disulfide in the presence of sodium hydride as a base has been developed.We have recently reported a synthesis of 2-arylimino-2,3-dihydropyrido[3,2-e]-1,3-thiazin-4-ones by the reaction of N-substituted 2-chloropyridine-3-carboxamides with aryl isothiocyanates.1 As an extension of this study, it was anticipated that these amides would undergo addition/intramolecular substitution on treatment with carbon disulfide giving 2-thioxo-2,3-dihydropyrido[3,2-e]-1,3-thiazin-4-ones, which are of potential interest from a biological point of view as some molecules having the pyrido[3,2-e]-1,3-thiazin-4-one skeleton exhibit biological activity.2 In 1984 Koscik and Kristian reported a synthesis of 2-thioxopyrido[3,2-e]thiazin-4-one by a reaction of 2-chloropyridine-3-carbonyl isothiocyanate with sodium hydrogen sulfide.3 To the best of our knowledge, however, there have been no reports on the synthesis of its derivatives so far. In this paper we wish to report the results of our investigation, which offers a facile method for the preparation of this class of heterocycles.
The reactions for the synthesis of 3-alkyl-2-thioxo-2,3-dihydropyrido[3,2-e]-1,3-thiazin-4-ones (2) from N-alkyl-2-chloropyridine-3-carboxamides (1) were conducted as illustrated in Scheme 1. In order to investigate the generality of the present synthesis, ten amides were prepared by using three 2-chloropyridine-3-carbonyl chlorides, one of which were commercially available and others could be prepared from the respective commercially available carboxylic acids, and four commercially available primary amines (see Experimental).
To a stirred suspension of sodium hydride in DMF at room temperature was added one of the amides 1. After ceasing of evolution of hydrogen gas, carbon disulfide was added, and the mixture was stirred overnight at the same temperature. The usual aqueous workup and subsequent purification by recrystallization or column chromatography on neutral alumina gave the desired products 2; these compounds were unstable on silica gel. The yields of the products 2 are also summarized in Scheme 1.
First, the reactions using 2-chloropyridine-3-carboxamides (1a-d) were carried out. These amides reacted efficiently with carbon disulfide to give expected products (2a-d), respectively, in moderate-to-fair yields. Next, 2-chloro-6-methylpyridine-3-carboxamides (1e-g) were used. The reactions gave the expected products (2e-g), respectively. The yields were similar to those using 2a-d. Finally, 2,6-dichloropyridine-3-carboxamides (1h-j) were subjected to reaction with carbon disulfide under the same conditions as those applied to 1a-g. We found that the reactions using these amides resulted in the formation of somewhat complicated reaction mixtures and the yields of the products (2h-j) were somewhat poorer than those using the other carboxamides. This may be attributable to lability of the 6-chloro substituent under the reaction conditions. It should be noted that the use of 2-chloro-N-isopropylpyridine-3-carboxamide in the present reaction resulted in the formation of an intractable mixture of products including a considerable amount of the starting material, indicating that the presence of α-alkyl branching in the N-alkyl group decreases the reactivity toward carbon disulfide. 2-Chloro-N-phenylpyridine-3-carboxamide did not work well in the present reaction; the starting material was recovered almost quantitatively.
The formation of thioxopyridothiazinones (2) is thought to commence with addition of the amide anion (3) to carbon disulfide to afford the dithiocarbamate anion intermediate (4), which underwent intramolecular substitution at the 2-position of the pyridine ring to result in the formation of 2, as depicted in Scheme 2.
In conclusion, we have demonstrated that 3-alkyl-2-thioxo-2,3-dihydropyrido[3,2-e]-1,3-thiazin-4-ones
could be prepared efficiently using the one-pot addition/intramolecular substitution sequence between N-alkyl-2-chloropyridine-3-carboxamides and carbon disulfide. The present method must be useful in organic synthesis, because it is operationally very simple and the starting materials are readily available.
EXPERIMENTAL
General. All melting points were obtained on a Laboratory Devices MEL-TEMP II melting apparatus and are uncorrected. IR spectra were determined with a Shimadzu FTIR-8300 spectrophotometer. The 1H NMR spectra were determined in CDCl3 using TMS as an internal reference with a JEOL ECP500 FT NMR spectrometer operating at 500 MHz. The 13C NMR spectra were determined in CDCl3 using TMS as an internal reference with a JEOL ECP500 FT NMR spectrometer operating at 125 MHz. Low-resolution MS spectra (EI, 70 eV) were measured by a JEOL JMS AX505 HA spectrometer. Thin-layer chromatography (TLC) was carried out on Merck Alumina 60 Neutral F254 or Merck Kieselgel 60 PF254. Column chromatography was carried out on Merck Alumina, Activated, Neutral, Activity I or Merck Kieselgel 60 (0.063–0.200 mm). All of the organic solvents used in this study were dried over appropriate drying agents and distilled prior to use.
Starting Materials. 2-Chloropyridine-3-carboxamides 1 were prepared by treating the respective 2-chloropyridine-3-carbonyl chlorides with primary amines. 2-Chloropyridine-3-carbonyl chloride was commercially available, and 2-chloro-6-methyl-3-carbonylchloride4 and 2,6-dichloropyridine-3-carbonyl chloride5 were prepared according to the appropriate reported procedures. Compounds 1a,6 1b,7 1c,7 1d,8 1e-g,9 and 1h10 are known compounds. Physical and spectral data for new compounds follow. 2,6-Dichloro-N-ethylpyridine-3-carboxamide (1i): a white solid; mp 89–91 ˚C (hexane–THF); IR (KBr) 3269, 1643 cm–1; 1H NMR δ 1.28 (t, J = 7.3 Hz, 3H), 3.50–3.56 (m, 2H), 6.48 (br s, 1H), 7.37 (d, J = 7.8 Hz, 1H), 8.11 (d, J = 7.8 Hz, 1H). Anal. Calcd for C8H8Cl2N2O: C, 43,86; H, 3.68; N, 12.79. Found: C, 43.82; H, 3.75; N, 12.63. 2,6-Dichloro-N-propylpyridine-3-carboxamide (1j): a white solid; mp 90–94 (hexane–THF); IR (KBr) 3260, 1647 cm–1; 1H NMR δ 1.02 (t, J = 7.3 Hz, 3H), 1.67 (sext, J = 7.3 Hz, 2H), 3.45 (q, J = 7.3 Hz, 2H), 6.53 (br s, 1H), 7.37 (d, J = 7.8 Hz, 1H), 8.10 (d, J = 7.8 Hz, 1H). Anal. Calcd for C9H10Cl2N2O: C, 46.37; H, 4.32; N, 12.02. Found: C, 46.17; H, 4.40; N, 11.87. All other chemicals used in this study were commercially available.
Typical Procedure for the Preparation of Thioxopyridothiazinones (2). 3-Methyl-2-thioxo-2,3- dihydropyrido[3,2-e]-1,3-thiazine-4-one (2a). A stirred suspension of NaH (60% in oil; 1.1 mmol, 42 mg) in DMF (2 mL) at rt was added a solution of 1a (0.16 g, 0.96 mmol) in DMF (1 mL) dropwise. After 15 min, CS2 (0.11 g, 1.4 mmol) was added, and the mixture was allowed to stir overnight at the same temperature. Saturated aqueous NH4Cl (10 mL) was added, and organic materials were extracted with Et2O three times (10 mL each). The combined extracts were washed with brine and dried over anhydrous Na2SO4. After evaporation of the solvent, the residual solid was recrystallized from pentane to give 2a (0.13 g, 64%); a yellow solid; mp 145–148 ˚C (pentane); IR (KBr) 1680, 1410, 1103 cm–1; 1H NMR δ 3.94 (s, 3H), 7.39 (dd, J = 7.8, 4.8 Hz, 1H), 8.57 (dd, J = 7.8, 1.8 Hz, 1H), 8.74 (dd, J = 4.8, 1.8 Hz, 1H); MS m/z 210 (M+, 100). Anal. Calcd for C8H6N2OS2: C, 45.69; H, 2.88; N, 13.32. Found: C, 45.68; H, 2.78; N, 13.20.
3-Ethyl-2-thioxo-2,3-dihydropyrido[3,2-e]-1,3-thiazine-4-one (2b): a yellow solid; mp 128–131 ˚C (hexane–Et2O); IR (KBr) 1682, 1412, 1107 cm–1; 1H NMR δ 1.36 (t, J = 7.3 Hz, 3H), 4.75 (q, J = 7.3 Hz, 2H), 7.38 (dd, J = 8.2, 4.6 Hz, 1H), 8.55 (dd, J = 8.2, 1.4 Hz, 1H), 8.72 (dd, J = 4.6, 1.4 Hz, 1H); 13C NMR δ 11.77, 43.02, 121.09, 122.67, 139.33, 154.38, 157.22, 160.55, 192.63; MS m/z 224 (M+, 100). Anal. Calcd for C9H8N2OS2: C, 48.19; H, 3.59; N, 12.49. Found: C, 48.13; H, 3.78; N, 12.35.
3-Propyl-2-thioxo-2,3-dihydropyrido[3,2-e]-1,3-thiazine-4-one (2c): a yellow oil; Rf 0.71 (hexane–THF, 2:1); IR (neat) 1682, 1410, 1115 cm–1; 1H NMR δ 1.00 (t, J = 7.3 Hz, 3H), 1.80 (sext, J = 7.3 Hz, 2H), 4.62 (t, J = 7.3 Hz, 2H), 7.38 (dd, J = 7.8, 4.6 Hz, 1H), 8.54 (dd, J = 7.8, 1.8 Hz, 1H), 8.72 (dd, J = 4.6, 1.8 Hz, 1H); MS m/z 236 (M+, 100). Anal. Calcd for C10H10N2OS2: C, 50.40; H, 4.23; N, 11.75. Found: C, 50.29; H, 4.25; N, 11.66.
3-Phenylmethyl-2-thioxo-2,3-dihydropyrido[3,2-e]-1,3-thiazine-4-one (2d): a yellow solid; mp 108–112 ˚C (pentane); IR (KBr) 1674, 1412, 1155 cm–1; 1H NMR δ 5.94 (s, 2H), 7.27 (t, J = 7.3 Hz, 1H), 7.32 (dd, J = 7.8, 7.3 Hz, 2H), 7.38 (dd, J = 8.2, 4.6 Hz, 1H), 7.45 (d, J = 7.8 Hz, 2H), 8.54 (dd, J = 8.2, 1.8 Hz, 1H), 8.73 (dd, J = 4.6, 1.8 Hz, 1H); MS m/z 286 (M+, 100). Anal. Calcd for C14H10N2OS2: C, 58.72; H, 3.52; N, 9.78. Found: C, 58.89; H, 3.69; N, 9.62.
3,7-Dimethyl-2-thioxo-2,3-dihydropyrido[3,2-e]-1,3-thiazine-4-one (2e): a yellow solid; mp 144–148 ˚C (pentane); IR (KBr) 1684, 1356, 1107 cm–1; 1H NMR δ 2.64 (s, 3H), 3.93 (s, 3H), 7.22 (d, J = 8.2 Hz, 1H), 8.42 (d, J = 8.2 Hz, 1H); 13C NMR δ 24.80, 34.88, 118.32, 122.79, 139.36, 156.65, 161.24, 165.21, 193.19; MS m/z 224 (M+, 100). Anal. Calcd for C9H8N2OS2: C, 48.19; H, 3.59; N, 12.49. Found: C, 48.34; H, 3.68; N, 12.31.
3-Ethyl-7-methyl-2-thioxo-2,3-dihydropyrido[3,2-e]-1,3-thiazine-4-one (2f): a yellow solid; mp 72–75 ˚C (pentane–Et2O); IR (KBr) 1688, 1352, 1109 cm–1; 1H NMR δ 1.35 (t, J = 7.3 Hz, 3H), 2.62 (s, 3H), 4.74 (q, J = 7.3 Hz, 2H), 7.21 (d, J = 8.2 Hz, 1H), 8.40 (d, J = 8.2 Hz, 1H); MS m/z 238 (M+, 100). Anal. Calcd for C10H10N2OS2: C, 50.40; H, 4.23; N, 11.75. Found: C, 50.29; H, 4.06; N, 11.65.
7-Methyl-3-propyl-2-thioxo-2,3-dihydropyrido[3,2-e]-1,3-thiazine-4-one (2g): a yellow solid; mp 49–52 ˚C (cyclohexane); IR (KBr) 1681, 1354, 1118 cm–1; 1H NMR δ 0.99 (t, J = 7.3 Hz, 3H), 1.79 (sext, J = 7.3 Hz, 2H), 2.62 (s, 3H), 4.61 (t, J = 7.3 Hz, 2H), 7.21 (d, J = 8.2 Hz, 1H), 8.40 (d, J = 8.2 Hz, 1H); MS m/z 252 (M+, 100). Anal. Calcd for C11H12N2OS2: C, 52.35; H, 4.79; N, 11.10. Found: C, 52.21; H, 4.84; N, 11.01.
7-Chloro-3-methyl-2-thioxo-2,3-dihydropyrido[3,2-e]-1,3-thiazine-4-one (2h): a yellow solid; mp 68–71 ˚C (cyclohexane); IR (KBr) 1684, 1348, 1101 cm–1; 1H NMR δ 3.92 (s, 3H), 7.37 (d, J = 8.2 Hz, 1H), 8.47 (d, J = 8.2 Hz, 1H); MS m/z 244 (M+, 100). Anal. Calcd for C8H5ClN2OS2: C, 39.26; H, 2.06; N, 11.45. Found: C, 39.10; H, 2.15; N, 11.46.
7-Chloro-3-ethyl-2-thioxo-2,3-dihydropyrido[3,2-e]-1,3-thiazine-4-one (2i): a yellow solid; mp 63–67 ˚C (pentane); IR (KBr) 1682, 1352, 1109 cm–1; 1H NMR δ 1.34 (t, J = 7.3 Hz, 3H), 4.72 (q, J = 7.3 Hz, 2H), 7.35 (d, J = 8.2 Hz, 1H), 8.46 (d, J = 8.2 Hz, 1H); MS m/z 258 (M+, 100). Anal. Calcd for C9H7ClN2OS2: C, 41.78; H, 2.73; N, 10.83. Found: C, 41.69; H, 2.80; N, 10.92.
7-Chloro-3-propyl-2-thioxo-2,3-dihydropyrido[3,2-e]-1,3-thiazine-4-one (2j): a yellow solid; mp 58–61 ˚C (pentane); IR (KBr) 1690, 1348, 1115 cm–1; 1H NMR δ 1.00 (t, J = 7.3 Hz, 3H), 1.78 (sext, J = 7.3 Hz, 2H), 4.59 (t, J = 7.3 Hz, 2H), 7.35 (d, J = 8.2 Hz, 1H), 8.45 (d, J = 8.2 Hz, 1H); 13C NMR δ 11.14, 19.74, 48.98, 119.82, 123.42, 141.60, 156.63, 157.69, 160.08, 191.65; MS m/z 272 (M+, 85), 171 (100). Anal. Calcd for C10H9ClN2OS2: C, 44.03; H, 3.33; N, 10.27. Found: C, 43.98; H, 3.39; N, 10.39.
ACKNOWLEDGEMENTS
We gratefully acknowledge Mrs. Miyuki Tanmatsu of this university for determining mass spectra and performing combustion analyses.
References
1. K. Kobayashi, T. Komatsu, D. Nakamura, and H. Konishi, Heterocycles, 2009, 78, 1041. CrossRef
2. (a) T. Zawisza, W. Malinka, and T. Jakobiec, Acta Pol. Pharm., 1981, 38, 145; (b) K. Ohno, R. Tsutsumi, N. Matsumoto, H. Yamashita, Y. Amada, J. Shishikura, H. Inami, S. Yatsugi, M. Okada, S. Sakamoto, and T. Yamaguchi, J. Pharmacol. Exp. Ther., 2003, 306, 66; CrossRef (c) H. Yamashita, K. Ohno, Y. Amada, H. Hattori, Y. Ozawa-Funatsu, T. Toya, H. Inami, J. Shishikura, M. Okada, and T. Yamaguchi, J. Pharmacol. Exp. Ther., 2004, 308, 127; CrossRef (d) H. Yamashita, K. Ohno, H. Imai, J. Shishikura, S. Sakamoto, M. Okada, and T. Yamaguchi, Eur. J. Pharmacol., 2004, 494, 147. See also pertinent references cited therein. CrossRef
3. D. Koscik and P. Kristian, Chem. Zve., 1984, 38, 111.
4. P. Schell, M. P. Richards, K, Hanson, S. C. Berk, and G. M. Makara, J. Comb. Chem., 2005, 7, 96. CrossRef
5. F. Mutterer and C. D. Weis, Helv. Chim. Acta, 1976, 59, 222. CrossRef
6. A. D. Dunn and R. Norrie, Z. Chem., 1988, 28, 248.
7. L. Thunus and C. L. Lapiere, Eur. J. Med. Chem., 1974, 9, 55.
8. R. Ferraccioli, D. Carenzi, O. Rombola, and M. Catellani, Org. Lett., 2004, 6, 4759. CrossRef
9. N. I. Shramm, N. A. Podushkina, V. S. Zalesov, and M. E. Konshin, Khim.-Farmatsevticheskii Zh., 1981, 15, 35.
10. Y. Hirokawa, T. Horikawa, and S. Kato, Chem. Pharm. Bull., 2000, 48, 1847.