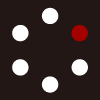
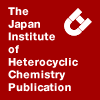
HETEROCYCLES
An International Journal for Reviews and Communications in Heterocyclic ChemistryWeb Edition ISSN: 1881-0942
Published online by The Japan Institute of Heterocyclic Chemistry
e-Journal
Full Text HTML
Received, 20th March, 2009, Accepted, 18th June, 2009, Published online, 19th June, 2009.
DOI: 10.3987/COM-09-11754
■ Flavonol Glycosides from the Native American Plant Gaura longiflora
Wen-Hui Xu, Melissa R. Jacob, Ameeta K. Agarwal, Alice M. Clark, Zong-Suo Liang, and Xing-Cong Li*
National Center for Natural Products Research, Research of Pharmaceutical Sciences, School of Pharmacy, The University of Mississippi, University , MS 38677, U.S.A.
Abstract
Phytochemical investigation of the native American plant Gaura longiflora led to the isolation of three new and eight known flavonol glycosides. The structures of the new compounds were established primarily by spectroscopic data as quercetin 3-O-(2''-O-α-L-rhamnopyranosyl-6''-O-E-p-coumaroyl)-β-D- glucopyranoside (1), kaempferol 3-O-(2''-O-α-L-rhamnopyranosyl-6''-O-E-p- coumaroyl)-β-D-glucopyranoside (2) and quercetin 3-O-(2″-O-α-L-rhamno- pyranosyl-6''-O-Z-p-coumaroyl)-β-D-glucopyranoside (3).In our search for prototype antifungal agents from natural sources, the organic extract of the native American plant Gaura longiflora Spach (Onagraceae) showed marginal antifungal activity. Preliminary fractionation failed to produce enriched antifungal activity for follow-up bioassay-guided fractionation. However, this plant is a member of the native North American genus Gaura consisting of 21 species1 that have been scarcely studied phytochemically. Two species of this small genus, G. coccomea3 and G. triangulate,3 were reported to contain flavonoids, while another three species, G. drummondii,4 G. suffulta,5 and the title plant G. longiflora5 were only investigated for the volatile constituents. The flavonoid chemistry of G. longiflora thus became our interest and a phytochemical investigation has been conducted. Herein we report the isolation and structure elucidation of three new flavonol glycosides (1−3), along with eight known compounds (4−11).
The 95% EtOH extract of the whole plant of G. longiflora was chromatographed on silica gel. The column fraction containing flavonol glycosides as determined by TLC was further chromatographed on silica gel and reversed-phase HPLC to afford 11 flavonol glycosides including three new compounds (1−3). The known compounds were identified by comparison of their ESIMS and NMR spectroscopic data with those reported in the literature as kaempferol 3-O-glucoside (4)6 kaempferol 3-O-α-rhamnopyranosyl (1→2)-β-glucopyranoside (5),6 kaempferol 3-O-rutinoside (6),6 quercetin 3-neohesperidoside (7),6 quercetin 3-rutinoside (8),6 kaempferol 3-O-(2'',6''-di-O-rhamnopyranosyl)- glucopyranoside (9),6 quercetin 3-O-(2'',6''-di-O-rhamnopyranosyl)-glucopyranoside (10),6 and quercetin 3-O-(2''-O-α-rhamnopyranosyl-6''-O-galloyl)-β-glucopyranoside (11).7
Compound 1 was obtained as an amorphous yellow powder. The negative-ion high resolution ESIMS of 1 showed a pseudomolecular ion peak at m/z 755.1844, suggesting an empirical molecular formula of C36H36O18 that was in accordance with its 13C NMR spectrum displaying 36 resonance signals. The IR spectrum indicated the presence of hydroxy (3348 cm−1) and carbonyl (1655 cm−1) groups. Acid hydrolysis of 1 afforded quercetin, p-coumaric acid, and D-glucose and L-rhamnose in a ratio of 1:1. The quercetin skeleton in 1 was evident from the 1H NMR signals at δH 7.58 (2H, m, H-2', H-6'), 6.86 (1H, d, J = 9.0 Hz, H-5'), 6.27 (1H, s, H-8), 6.09 (1H, s, H-6) and 13C NMR signals at δC 117.6 (C-2'), 123.9 (C-6'), 116.3 (C-5'), 95.0 (C-8), 100.3 (C-6),8,9 while the trans-p-coumaroyl structural moiety was indicated by the 1H NMR signals at δH 7.38 (1H, d, J = 16.0 Hz, H-7''''), 6.06 (1H, d, J = 16.0 Hz, H-8''''), 7.28 (2H, d, J = 8.0 Hz, H-2'''', H-6''''), 6.82 (2H, d, J = 8.0 Hz, H-3'''', H-5''''), and the 13C NMR signals at δC 169.2 (C-9''''), 161.5 (C-4''''), 146.9 (C-7''''), 131.6 (C-2'''',6''''), 127.5 (C-1''''), 117.2 (C-3'''',5''''), 115.0 ( C-8'''').10 The two anomeric protons at δH 5.70 (1H, d, J = 7.5 Hz, H-1'') and 5.26 (1H, s, H-3'''), which correlated with two anomeric carbons at δC 100.5 (C-1'') and 103.1 (C-3'''), respectively, in the HMQC spectrum revealed the presence of β-D-glucopyranosyl and α-L-rhamnopyranosyl units. The 13C NMR signals due to the aglycone moiety of 1 were very similar to those of 10,6 indicating that the C-3 hydroxy group was glycosylated by a disaccharide moiety attached to a coumaroyl group. The negative-ion ESIMS spectrum exhibited fragmentation ions at m/z 609.1 [M − 146 (rhamnosyl or coumaroyl) − H]− and 463.1 [609.1 − 146 × 2 (rhamnosyl and coumaroyl) − H]−, indicating the rhamnosyl unit was located at the terminal position. The interglycosidic linkage between the rhamnosyl and glucosyl units and the connectivity between the p-coumaroyl group to the sugar moiety were established by the analysis of the HMBC spectrum. The three-bond long-range correlations between H-1'''(δH 5.26)and C-2'' (δC 80.5) and between H-2''(δH 3.72)and C-1''' (δC 103.1) confirmed the terminal rhamnosyl unit was linked to the C-2 hydroxy group of the inner glucosyl unit, while the correlation between H-6'' (δH 4.36) and C-9'''' (δC 169.2) indicated that the p-coumaroyl group was attached to the C-6 hydroxy group of the glucosyl unit. Thus, the structure of 1 was determined as quercetin 3-O-(2''-O-α-L-rhamnopyranosyl-6''-O-E-p-coumaroyl)-β-D-glucopyranoside.
Compound 2 produced a pseudomolecualr ion peak at m/z 739.1849 in the negative-ion high resolution ESIMS, which is 16 mass unit corresponding to an oxygen atom less than compound 1. In conjunction with the 13C NMR spectrum, its molecular formula was determined as C36H36O17. Comparison of the 1H and 13C NMR spectra of 2 with those of 1 indicated that the only difference between them is the aglycone moiety with a different substitution pattern on B-ring. The presence of kaempferol11 in 2 was supported by the 1H NMR signals at δH 7.99 (2H, d, J = 9.0 Hz, H-2', H-6'), 6.87 (2H, d, J = 8.5 Hz, H-3', H-5'), 6.27 (1H, s, H-8), 6.08 (1H, s, H-6) and 13C NMR signals of B-ring at δC 123.4 (C-1'), 132.4 (C-2', C-6'), 157.9 (C-4'), 116.4 (C-3', C-5') as well as other signals of A- and C-ring shown in Table 1. The fragmentation ion at m/z 593.1 [M − 146 (rhamnosyl or coumaroyl) − H]− in the negative-ion ESIMS spectrum also supported the same sequence of the sugar and acyl moieties as in 1. Thus, the structure of 2
was established as kaempferol 3-O-(2''-O-α-L-rhamnopyranosyl-6''-O-E-p-coumaroyl)-β-D-glucopyranoside. It is noted that SciFinder® has incorrectly assigned this structure to a compound (CA registry # 78510-18-6) isolated from Castanea sativa whose correct structure is kaempferol 3-O-(2''-O-E-p-coumaroyl-6''-O-α-L-rhamnopyranosyl-O-E-p-coumaroyl)-β-D-glucopyranoside.12
Compound 3 was isolated in limited quantity and a reasonable 13C NMR spectrum was not obtained. However, the close similarities of its UV, IR, ESIMS, and 1H NMR spectra to those of compound 1 prompted us to conduct the structure elucidation of this compound. First, the key structural information derived from the negative-ion high resolution ESIMS that showed a pseudomolecular ion peak at m/z 755.1758 indicating the same molecular formula C36H36O18 as for 1. Secondly, the presence of quercetin and glucopyranosyl and rhamnopyranosyl units is confirmed by the close resemblance of the 1H NMR signals due to the aglycone and sugar moieties to those of 1 (Table 1). The negative-ion ESIMS spectrum also exhibited fragmentation ions at m/z 609.1 [M − 146 (rhamnosyl or coumaroyl) − H]− and 463.1 [M − 146 × 2 (rhamnosyl and coumaroyl) − H]− similar to those of 1, indicating the rhamnosyl unit was located at the terminal position. The only difference between the two compounds is that a cis-p-coumaroyl group is present in 3, as evident by the two typical doublets at δH 5.42 (H-8'''') and 6.61 (H-7'''') with coupling constants of 13 Hz,13 instead of a trans-p-coumaroyl group in 1. The slightly upfield shifts of the anomeric proton, C-5 proton, and one of the C-6 geminal protons of the glucopyranosyl unit resulted from the substitution of the cis-p-coumaroyl group in 3 when compared with 1 is consistent with those reported in the literature.14 Thus, the structure of compound 3 is quercetin 3-O-(2''-O-α-L-rhamnopyranosyl-6''-O-Z-p-coumaroyl)-β-D-glucopyranoside.
EXPERIMENTAL
General Procedure
Optical rotations were measured with an Autopol IV polarimeter. UV was obtained on an HP 8453 diode array spectrophotometer. IR spectra were recorded using a Thermo Nicolet IR 300 FT/IR spectrometer.
The 1D and 2D NMR (COSY, HMQC, HMBC) spectra using standard pulse programs were recorded at room temperature on a Bruker Avance DRX 500 FT spectrometer operating at 500 (1H) or 125 (13C) MHz or a Varion Inova 600 spectrometer operating at 600 (1H) MHz. The chemical shift values are relative to the internal standard TMS. ESIMS data were obtained on an Agilent Series 1100 SL mass spectrometer. Column chromatography was performed using normal phase silica gel (J. T. Baker, 40 µm) and reversed-phase silica gel (RP-18, J. T. Baker, 40 µm). Semi-preparative HPLC was conducted on a C18 column (Gemini, 250 × 10 mm, 5 μm) with UV detection at 254 nm. Analytical HPLC was performed on a C18 column (Gemini, 4.6 × 150 mm, 5 μm) with diode array detector at 250 nm. TLC was carried out on silica gel sheets (Alugram® Sil G/UV254, Macherey-Nagel, Germany) and reversed-phase plates (RP-18 F254S, Merck, Germany). Visualization: UV at 254 nm or spraying with 10% H2SO4 followed by heating.
Plant Material
The whole plant of Gaura longiflora Spach was collected by G. Walters, S. Bodine & Huong 690 in Cuivre River State Park, Lincoln County, Missouri, USA (coordinates: 39º03'56"N 090º55'58"W) on August 21, 2001, and identified by Nancy Parker. A voucher specimen (MO 5288289) is deposited in the Herbarium of Missouri Botanical Garden, MO, USA.
Extraction and Isolation
Powdered, air-dried whole plant of G. longiflora (98 g) was extracted with 95% EtOH (1 L × 3) at room temparature for 24 h. Removal of the solvent by evaporation in vacuo yielded a residue (7.45 g), which was directly subjected to silica gel column chromatography using a stepwise gradient elution of CHCl3/MeOH (100:1 to 100:15) and finally with MeOH to afford 12 pooled fractions (A−N) according to TLC. The most polar fraction N (3.01 g) containing flavonoid glycosides was further subjected to RP-18 column chromatography using a stepwise gradient elution of MeOH/H2O (10−80%) and finally with MeOH to give 9 pooled fractions. Fraction 7 (168 mg) was chromatographed on a semi-preparative C18 reversed-phase HPLC column using 20% MeCN/H2O (5 mL/min at 254 nm) to give compounds 4 (1.6 mg, tR = 10.50 min), 5 (4.0 mg, tR = 9.48 min), 7 (5.3 mg, tR = 6.90 min), 8 (18 mg, tR = 8.27 min), 9 (4.5 mg, tR = 6.46 min), 10 (9.4 mg, tR = 5.00 min), and 11 (6.7 mg, tR = 6.10 min). Similarly, fraction 8 (55 mg) was chromatographed on a semi-preparative C18 reversed-phase HPLC column using 30% MeCN/H2O (5 mL/min at 254 nm) to give compounds 1 (6.1 mg, tR = 5.40 min), 2 (2.8 mg, tR = 6.82 min), 3 (2.5 mg, tR = 6.04 min), and 6 (8.4 mg, tR = 3.60 min).
Compound 1, amorphous yellow powder; [α]25D −18.6 (c 0.7, MeOH); UV λmax (MeOH) 315 (ε 4819), 265 (ε 4378); IR (neat) νmax: 3348, 1655, 1603, 1514, 1448, 1358, 1263, 1171, 1054, 932, 812 cm−1; NMR data (MeOH-d4), Table 1; ESIMS m/z 755.1844 (calcd for [C36H36O18 − H] −, 755.1829).
Compound 2, amorphous yellow powder; [α]25D −10.9 (c 0.11, MeOH); UV λmax (MeOH) 315 (ε 3926), 265 (ε 3751); IR (KBr) νmax : 3347, 1653, 1541, 1457, 1054 cm−1; NMR data (MeOH-d4), Table 1; ESIMS m/z 739.1849 (calcd for [C36H36O17 − H] −, 739.1880).
Compound 3, amorphous yellow powder; [α]25D −2.4 (c 0.9, MeOH); UV λmax (MeOH) 315 (ε 4535), 265 (ε 5779); IR (neat) νmax: 3350, 1647, 1513, 1362, 1271, 1202, 1072 cm−1; NMR data (MeOH-d4), Table 1; ESIMS m/z 755.1758 (calcd for [C36H36O18 − H] −, 755.1829), 791.1508 (calcd for [C36H36O18 + Cl] −, 791.1596).
Acid Hydrolysis of Compound 1
A solution of compound 1 (3.1 mg) in 1 M HCl/dioxane (1:1, 2 mL) was refluxed at 80 °C for 3 h. After cooling, the reaction mixture was diluted with H2O (2 mL) and extracted with CHCl3 (3 mL × 3). The CHCl3 extract was analyzed by TLC (silica gel, CHCl3–MeOH–AcOH–H2O, 70:30:10:5). Quercetin (Rf = 0.69) and p-coumaric acid (Rf = 0.87) were detected by comparison with authentic samples. The aqueous layer was neutralized by passing through an Amberlite MB-150 column eluting with H2O. The eluent was concentrated to dryness to yield a sugar residue. The sugar residue was analyzed by TLC (silica gel, CHCl3–MeOH–AcOH–H2O, 70:30:10:5) in comparison with standard samples. Glucose (Rf = 0.10) and rhamnose (Rf = 0.26) were detected. Determination of the absolute configuration of the sugars followed a recently reported procedure.15 Briefly, the sugar residue (about 1 mg) and L-cysteine methyl ester (1 mg) was dissolved in pyridine (0.2 mL) and heated at 60 °C for 1 h, and then o-tolyl isothiocyanate (1 mg) was added to the mixture and heated at 60 °C for another 1 h. The reaction mixture (10 µL) was analyzed by analytical HPLC using a gradient elution of 15 to 85% aqueous MeCN containing 0.1% acetic acid at a flow rate of 1 mL/min over 35 minute-run. D-Glucose (tR = 17.15 min) and L-rhamnose (tR = 19.62 min) were identified by comparing their retention times with those of the authentic samples, while L-glucose showed a different retention time at 16.90 min. D-rhamnose was not included in the experiment. However, the method was sufficient to differentiate the D/L-form of rhamnose based on the reported retention times in the literature.15
ACKNOWLEDGMENTS
The authors thank Dr. Bharathi Avula for recording HR-ESI-MS spectra, Mr. Frank T. Wiggers for the assistance in obtaining NMR spectra, Mr. John Hester for repository management, and Dr. Wei Wang and Dr. Yanhong Wang for HPLC analysis. This work was supported by the NIH, NIAID, Division of AIDS, Grant No. AI 027094, the USDA Agricultural Research Service Specific Cooperative Agreement No. 58-6408-2-0009, and the China Scholarship Council.
References
1. G. D. Hoggard, P. J. Kores, M. Molvray, and R. K. Hoggard, Am. J. Bot., 2004, 91, 139. CrossRef
2. G. Z. Howard, T. J. Mabry, and P. H. Raven, Phytochemistry, 1972, 11, 289. CrossRef
3. R. A. Hilsenbeck, D. A. Levin, T. J. Mabry, and P. H. Raven, Phytochemistry, 1984, 23, 1077. CrossRef
4. R. Teranishi, R. G. Buttery, S. Kint, G. Takeoka, P. D. Lingren, J. R. Raulston, and T. N. Shaver, J. Essent. Oil Res., 1991, 3, 287.
5. Kint, R. Teranishi, P. D. Lingren, T. N. Shaver, and J. R. Raulston, J. Essent. Oil Res., 1993, 5, 201.
6. K. Kazuna, N. Noda, and M. Suzuki, Phytochemistry, 2003, 62, 229. CrossRef
7. M. S. Marzouk, F. A. Moharram, E. G. Haggag, M. T. Ibrahim, and O. A. Badary, Phytother. Res., 2006, 20, 200. CrossRef
8. H. J. Kim, E. H. Woo, C. G. Shin, and H. Park, J. Nat. Prod., 1998, 61, 145. CrossRef
9. A. A. Hussein, I. Barberena, M. Correa, P. D. Coley, P. N. Solis, and M. P. Gupta, J. Nat. Prod., 2005, 68, 231. CrossRef
10. A. Itoh, T. Tanahashi, N. Nagakura, Y. Takenaka, C. C. Chen, and J. Pelletier, J. Nat. Prod., 2004, 67, 427. CrossRef
11. G. Fico, A. Braca, A. R. Bilia, F. Tomè, and I. Morelli, J. Nat. Prod., 2000, 63, 1563. CrossRef
12. G. Romussi, L. Mosti, and G. Bignardi, Liebigs Ann. Chem., 1981, 761. CrossRef
13. H. Liu, J. Orjala, O. Sticher, and T. Rali, J. Nat. Prod., 1999, 62, 70. CrossRef
14. S. Tsukamoto, K. Tomise, M. Aburatani, H. Onuki, H. Hirorta, E. Ishiharajima, and T. Ohta, J. Nat. Prod., 2004, 67, 1839. CrossRef
15. T. Tanaka, T. Nakashima, T. Ueda, K. Tomii, and I. Kouno, Chem. Pharm. Bull., 2007, 55, 899. CrossRef