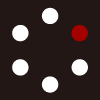
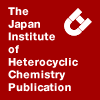
HETEROCYCLES
An International Journal for Reviews and Communications in Heterocyclic ChemistryWeb Edition ISSN: 1881-0942
Published online by The Japan Institute of Heterocyclic Chemistry
e-Journal
Full Text HTML
Received, 9th June, 2009, Accepted, 29th July, 2009, Published online, 31st July, 2009.
DOI: 10.3987/COM-09-11773
■ Two New Sesquiterpene Glucosides from Gymnaster koraiensis
Il Kyun Lee, Ki Hyun Kim, Shi Yong Ryu, and Kang Ro Lee*
Natural Products Laboratory, College of Pharmacy, Sungkyunkwan University, 300 Chunchun-dong, Jangan-ku, Wuwon 440-746, Korea
Abstract
Two new sesquiterpene glucosides, 1(R),4β-dihydroxy-trans-eudesm-6-ene-1-O-β-D-glucopyranoside (1) and 1(R),4β-dihydroxy-trans-eudesm-7-ene-1-O-β-D-glucopyranoside (2), together with six other known compounds, were isolated from the flowers of Gymnaster koraiensis (Nakai) Kitamura (Compositae). The identification and structural elucidation of these compounds were based on 1D- and 2D-NMR spectral data analysis. The absolute configurations of 1 and 2 were determined by a convenient Mosher ester procedure carried out in NMR tube.INTRODUCTION
Gymnaster koraiensis (Nakai) Kitamura (Compositae) is widely distributed in the north of Korea. This indigenous herb is used as a folk medicine for antitussive and antibacterial activities.1 Previous phytochemical studies on this plant showed the presence of polyacetylenes, polyacetylene glucosides and benzofurans.2-5 Column chromatographic purification of the BuOH-soluble fraction of the EtOH extract of the flowers of this source led to the isolation of two new sesquiterpene glucosides (1-2), together with six other known compounds (3-8). The structures of the known compounds were determined to be oplopanone-8-O-β-D-glucopyranoside (3),6,7 3(R)-8(E)-decene-4,6-diyne-1,3-diol-1-O-β-D-glucopyrano side (4),4,8 8(E)-decene-4,6-diyne-1-O-β-D-glucopyranoside (5),4 8(E)-decene-4,6-diyne-1-O-β-D- apinofuranosyl-(1′′→6′)-β-D-glucopyranoside (6),8 eugenyl-O-β-D-glucopyranoside (7)9 and 2-phenylethyl-O-β-D-glucopyranoside (8)10 by comparing their spectroscopic data with those in published literature. The known compounds (3, 5-8) were reported from this source for the first time.
RESULTS AND DISCUSSION
Compound 1 was obtained as a colorless gum, whose molecular formula was determined to be C21H36O7 from the [M + Na]+ peak at m/z 423.2362 (calcd. for C21H36O7Na : 423.2359) in the positive-ion HR-FABMS. The IR spectrum indicated that 1 possessed hydroxyl (3416 cm-1) and C=C double bond (1650 cm-1) functional groups. In the 13C-NMR (including DEPT) spectra, 21 carbon signals appeared, which included four methyl carbons at δC = 29.6, 22.2, 21.9 and 13.0, four methylene carbons at δC = 40.2, 36.5, 24.2 and 23.9, two methine carbons at δC = 51.9 and 36.7, one oxygenated methine carbon at δC = 85.8, two olefinic carbons δC = 145.3 and 118.2, one oxygenated quaternary carbon at δC = 71.7 and, one quaternary carbon at δC = 38.9, including six signals assignable to the glucose moiety (δC = 102.2, 78.4, 77.9, 75.3, 72.1, 63.2), were observed. The NMR data were very similar except for the glucose part to those of 1β,4β-dihydroxy-trans-eudesm-6-ene, which was isolated from Pulicaria paludosa.11 The only difference was the chemical shift at C-1 (δH = 3.44, dd, J = 12.0, 4.5 Hz ; δC = 85.8 in 1; δH = 3.35, dd, J = 11.6, 4.0 Hz ; δC = 80.0 in 1β,4β-dihydroxy-trans-eudesm-6-ene). The downfield shift at C-1 implied that 1 was glycosylated at C-1.12 The sugar moiety appeared at δH = 4.33 (d, J = 7.5 Hz), 3.85 (dd, J = 11.5, 2.5 Hz), 3.67 (dd, J = 11.5, 5.5 Hz), 3.36 (m), 3.30 (m), 3.24 (m), 3.16 (dd, J = 9.1, 7.5 Hz) in the 1H-NMR spectrum and at δC = 102.2, 78.4, 77.9, 75.3, 72.1, 63.2 in the 13C-NMR spectrum, suggesting the presence of D-glucose moiety. The coupling constant (J = 7.5 Hz) of the anomeric proton of D-glucose moiety indicated it to be the β-form.13 The position of D-glucose moiety was reconfirmed by an HMBC experiment, in which long-range correlation was observed between the H-1′ (δH = 4.33, d, J = 7.5 Hz) and C-1 (δC = 85.8) (Figure 2). Thus, the structure of 1 was supposed to be 1β,4β- dihydroxy-trans- eudesm-6-ene-1-O-β-D-glucopyranoside. The relative stereochemistry was confirmed by NOESY spectrum. The correlations of H-5 with H-1 and H-14 (not with C-15) were observed in the NOESY experiment (Figure 2). In addition, enzymatic hydrolysis of 1 with β-glucosidase (emulsin) yielded 1β,4β-dihydroxy-trans-eudesm-6-ene (1a, C15H26O2, [α]D25: - 12.0°), whose 1H-NMR and MS spectra were in good agreement with values reported previously,11,14 and D-glucose ([α]D25: + 50.4 ° (c 0.05, H2O). Determination of the absolute configuration at C-1 of 1 was examined with the convenient Mosher’s method.15 Compound 1a, obtained by enzyme hydrolysis of 1, was treated with (S)-(+)- and (R)-(-)-α-methoxy-α-trifluromethylphenylacetic acid (MTPA) chlorides to give (R)- and (S)-MTPA esters (1b and 1c, respectively). As shown in Figure 4, the H-2, 3 and 14 of the (S)-MTPA ester (1c) resonated at lower field than those of the (R)-MTPA ester (1b), while the H-8, 9 and 15 of 1s were observed at higher field compared to those of 1b. Consequently, the absolute configuration at C-1 in 1 was to be R-form. Thus, the structure of 1 was determined to be 1(R),4β-dihydroxy-trans-eudesm-6-ene-1-O-β-D-glucopyranoside.
Compound 2 was obtained as a colorless gum, whose molecular formula was determined to be C21H36O7 from the [M + Na]+ peak at m/z 423.2358 (calcd. for C21H36O7Na : 423.2359) in the positive-ion HR-FABMS. The IR spectrum indicated that 2 possessed a hydroxyl group at 3386 cm-1 and a C=C double bond at 1649 cm-1. The NMR spectra of 2 were very similar to those of compound 1. In the 13C-NMR spectrum of 2, two olefinic carbon signals observed at δC= 145.3 and 118.2 in 1 were slightly shifted upfield to δC = 142.9 and 118.0 in 2, respectively. Furthermore, the coupling pattern of an olefinic proton in the 1H-NMR spectrum was different (δH = 5.54, br. s in 1; δH = 5.53, br. d, J = 5.7 Hz in 2).16 These observations suggested that the structure of 2 was 1β,4β-dihydroxy-trans-eudesm-7-ene-1-O-β-D-glucopyranoside. Analysis of the 1H-1H COSY, HMQC and HMBC spectra permitted the assignment of all proton and carbon signals for 2 the location of the double bond, and the glycosyl linkage (Figure 3). Enzymatic hydrolysis of 2 with β-glucosidase (Emulsin) of 2 yielded 1β,4β-dihydroxy-trans-eudesm-7-ene (2a, C15H26O2, [α]D25: - 35.0°), whose 1H-NMR and MS spectra were in good agreement with values reported previously,11,17 and D-glucose ([α]D25: + 53.2 ° (c 0.05, H2O). The relative stereochemistry was confirmed by NOESY spectrum (Figure 3). Determination of the absolute configuration at C-1 of 2 was examined with the convenient Mosher’s method.15 Compound 2a, obtained by enzyme hydrolysis of 2, was treated with (S)-(+)- and (R)-(-)-α-methoxy-α-trifluromethylphenylacetic acid (MTPA) chlorides to give (R)- and (S)-MTPA esters (2b and 2c, respectively). As shown in Figure 4, the H-2, 3 and 14 of the (S)-MTPA ester (2c) resonated at lower field than those of the (R)-MTPA ester (2b), while the H-8, 9 and 15 of 1s were observed at higher field compared to those of 2b. Consequently, the absolute configuration at C-1 in 2 was to be R. Therefore, the structure of 2 was determined to be 1(R), 4β-dihydroxy-trans-eudesm-7-ene-1-O-β-D-glucopyranoside.
EXPERIMENTAL DETAILS
General. Optical rotations were measured on a Jasco P-1020 polarimeter in MeOH and H2O. IR spectra were recorded on a Bruker IFS-66/S FT-IR spectrometer. FAB and HR-FAB mass spectra were obtained on a JEOL JMS700 mass spectrometer. NMR spectra, including NOESY, DEPT and HMBC experiments, were recorded on a Varian UNITY INOVA 500 NMR spectrometer operating at 500 MHz (1H) and 125 MHz (13C), respectively, with chemical shifts given in ppm (δ) using TMS as an internal standard. Preparative HPLC was conducted using a Gilson 306 pump with Shodex refractive index detector. Silica gel 60 (Merck, 70-230 mesh and 230-400 mesh) and RP-C18 silica gel (Merck, 230-400 mesh) were used for column chromatography. Merck precoated Silica gel F254 plates and RP-18 F254s plates were used for TLC. Spots were detected on TLC under UV light or by heating after spraying with 10% H2SO4 in C2H5OH (v/v). The packing material for molecular sieve column chromatography was Sephadex LH-20 (Pharmacia Co.). Low pressure liquid chromatography was carried out over a Merck Lichroprep Lobar®-A Si 60 (240×10 mm) or a Lichroprep Lobar®-A RP-18 (240×10 mm) column with a FMI QSY-0 pump (ISCO).
Plant material. The flower parts of Gymnaster koraiensis (Nakai) Kitamura (Compositae) (5 kg) were collected at Pyeongchang in Gangwon province, Korea in August 2006 and identified by Prof. Kang Ro Lee. A voucher specimen of the plant (SKK-07-006) was deposited at the College of Pharmacy in Sungkyunkwan University.
Extraction and isolation. The half dried flower parts of G. koraiensis (5 kg) were extracted with EtOH three times at room temperature. The resultant EtOH extracts (250 g) were suspended in distilled water (800 mL Χ 3) and then successively partitioned with n-hexane, CHCl3, EtOAc and n-BuOH, yielding 27 g, 7 g and 85 g, respectively. The n-BuOH soluble fraction (85 g) was chromatographed on a Diaion HP-20, eluting with a gradient solvent system of water and MeOH to give two subfractions. Fraction B (48 g) silica gel (230-400 mesh, 350 g), was eluted with a gradient solvent system of MeOH/H2O (1:1, 3.5:1 and 1:0). According to TLC analysis, nine crude fractions (fr. BA-BI) were collected. Fr. BB (6.8 g) was further chromatographed on a CHCl3/MeOH/Water (35:10:1–10:5:1) to give nine fractions (BB1 – BB9). Fr. BB2 was eluted with a gradient solvent system of CHCl3/MeOH/Water (35:10:1) to give four subfractions (fr. BB21 – BB24). Fr. BB23 (540 mg) was column chromatography on a RP-C18 silica gel (230-400 mesh, 100 g), using a solvent system of 50% MeOH, and purified by preparative normal-phase HPLC with a solvent system of CHCl3/MeOH (6:1) to yield 1 (4 mg) and 2 (25 mg). Fr. BB22 (140 mg) was purified by Lobar®-A RP-18 (240×10 mm) column (25% MeOH), and further purified by preparative normal-phase HPLC, using a solvent system of CHCl3/MeOH (7:1) to yield 3 (19 mg). Fr. BA (2.7 g) silica gel (230-400 mesh 100 g) was eluted with a solvent system of MeOH/H2O (13:1). According to TLC analysis, seven fractions (fr. BA1-BA7) were collected. Fr. BA6 (220 mg) was further purified by preparative reversed-phase HPLC, using a solvent system of 55% MeOH to yield 7 (15 mg). Fr. BA7 (200 mg) was further purified by preparative normal-phase HPLC, using a solvent system of CHCl3/MeOH (7:1) to yield 4 (15 mg) and 8 (43 mg). Fr. BB24 (420 mg) was purified by Lobar®-A RP-18 (240×10 mm) column (55% MeOH), and further purified by preparative normal-phase HPLC, using a solvent system of CHCl3/MeOH (4:1) to yield 5 (138 mg). Fr. BB27 (1.5 g) was resolved by column chromatography on a silica gel (230-400 mesh, 100 g), eluting with a gradient solvent system of CHCl3/MeOH (6:1 and 4:1) to give three fractions (fr. BB271 – BB273). Fr. BB273 (200 mg) was purified by preparative normal-phase HPLC, using a solvent system of CHCl3/MeOH (4:1) to yield 6 (11 mg).
1(R),4β-Dihydroxy-trans-eudesm-6-ene-1-O-β-D-glucopyranoside (1). Colorless gum. [α]D25 - 51.5° (c 0.05, MeOH); IR (KBr) νmax cm-1: 3416, 2961, 1650, 1057, 1004; FABMS m/z (rel. int.) = 423 ([M + Na]+); HR-FABMS m/z = 423.2362 [M + Na]+ (calcd for C21H36O7Na:423.2359); 1H- NMR: see Table 1.; 13C-NMR (CD3OD, 125 MHz): δ 145.3 (C-7), 118.2 (C-6), 102.2 (C-1′), 85.8 (C-1), 78.4 (C-3′), 77.9 (C-5′), 75.3 (C-2′), 72.1 (C-4′), 71.7 (C-4), 63.2 (C-6′), 51.9 (C-5), 40.2 (C-3), 38.9 (C-10), 36.7 (C-11), 36.5 (C-9), 29.6 (C-14), 24.2 (C-2), 23.9 (C-8), 22.2 (C-13), 21.9 (C-12), 13.0 (C-15).
1(R),4β-Dihydroxy-trans-eudesm-7-ene-1-O-β-D-glucopyranoside (2). Colorless gum. [α]D25 - 43.7 ° (c 0.2, MeOH); IR (KBr) νmax cm-1: 3386, 2960, 1649, 1372, 1076, 1024; FABMS m/z (rel. int.) = 423 ([M + Na]+); HR-FABMS m/z = 423.2358 [M + Na]+ (calcd. for C21H36O7Na:423.2359); 1H- NMR: see Table 1.; 13C-NMR (CD3OD, 125 MHz): δ 142.9 (C-7), 118.0 (C-8), 101.9 (C-1′), 86.9 (C-1), 79.6 (C-3′), 77.9 (C-5′), 75.3 (C-2′), 72.1 (C-4′), 71.6 (C-4), 63.2 (C-6′), 48.5 (C-5), 42.0 (C-9), 40.3 (C-3), 38.4 (C-10), 36.5 (C-11), 30.0 (C-14), 24.3 (C-6), 23.9 (C-2), 22.4 (C-13), 21.8 (C-12), 13.2 (C-15).
Enzymatic hydrolysis of 1 and 2 using β-glucosidase. Compound 1 (2.0 mg) with 2 mL of H2O and 4 mg of β-glucosidase18,19 (Emulsin) was shaken for 7 days at 36 °C. The H2O solution was then extracted with EtOAc three times, and the EtOAc extract was evaporated in vacuo. The EtOAc extract (2.0 mg) was purified using Silica HPLC (CHCl3:MeOH = 9:1) to afford aglycone 1a (1.5 mg) as a colorless gum [α]D25 - 12.0 ° (c 0.05, CHCl3), 1H-NMR (CDCl3, 500 MHz): see Table 1. Compound 2 (3.0 mg) was treated by the same method. The EtOAc extract (2.0 mg) was purified using silica HPLC (CHCl3:MeOH = 9:1) to afford aglycone 2a (1.5 mg) as a colorless gum [α]D25 - 35.0 ° (c 0.1, CHCl3), 1H-NMR (CDCl3, 500 MHz): see Table 1. The sugar in the water layer was identified as D-glucose by co-TLC (EtOAc:MeOH:H2O = 9:3:1, Rf value : 0.2, 1a : 0.5 mg, 2a : 0.5 mg) with a D-glucose standard (Aldrich Co., USA).
Preparation of the (R)- and (S)-MTPA Ester Derivatives of 1a and 2a by a Convenient Mosher Ester.15 Compounds 1a (0.7 mg) and 2a (0.7 mg) in deuterated pyrindine-d5 (1.0 mL) was transferred into clean NMR tube. (S)-(+)-α-methoxy-α-trifluromethylphenylacetic acid (MTPA) chlorides (10 µL) was added into the NMR tube immediately under a N2 gas stream, and then the NMR tube was permitted to stand at room temperature. After overnight, the reaction was completed to afford the (R)-MTPA ester derivatives (1b and 2b) of 1a and 2a, respectively. In manner described for 1b and 2b, (S)-MTPA ester derivatives (1c and 2c) of 1a and 2a were obtained. The 1H-NMR spectra of 1b, 2b, 1c and 2c were measured with the reaction NMR tubes directly.
1b. (500 MHz, pyridine-d5): δ 5.719 (1H, br s, H-6), 5.040 (1H, dd, J = 11.5, 4.0 Hz, H-1), 2.135 (1H, q, J = 7.0 Hz, H-11), 2.064 (1H, m, H-9a), 2.045 (1H, m, H-8a), 2.025 (1H, m, H-8b), 1.998 (1H, m, H-2a), 1.830 (1H, m, H-5), 1.690 (1H, m, H-3a), 1.638 (1H, m, H-2b), 1.529 (1H, m, H-3b), 1.485 (1H, m, H-9b), 1.373 (3H, s, H-14), 1.340 (3H, s, H-15), 1.914 (3H, d, J = 7.0 Hz, H-12), 0.908 (3H, d, J = 7.0 Hz, H-13).
1c. (500 MHz, pyridine-d5): δ 5.709 (1H, br s, H-6), 5.040 (1H, dd, J = 11.5, 4.0 Hz, H-1), 2.133 (1H, q, J = 7.0 Hz, H-11), 2.110 (1H, m, H-9a), 2.044 (1H, m, H-8a), 2.012 (1H, m, H-8b), 2.010 (1H, m, H-2a), 1.800 (1H, m, H-5), 1.714 (1H, m, H-3a), 1.642 (1H, m, H-2b), 1.536 (1H, m, H-3b), 1.457 (1H, m, H-9b), 1.376 (3H, s, H-14), 1.310 (3H, s, H-15), 0.907 (3H, s, J = 7.0 Hz, H-12), 0.888 (3H, d, J = 7.0 Hz, H-13).
2b. (500 MHz, pyridine-d5): δ 5.289 (1H, br s, H-8), 4.999 (1H, dd, J = 12.5, 3.5 Hz, H-1), 2.496 (1H, m, 9a), 2.496 (1H, m, H-6a), 2.268 (1H, qd, J = 13.5, 3.5, H-2a), 2.144 (1H, q, J = 7.0 Hz, H-11), 2.098 (1H, m, H-6b), 2.053 (1H, m, H-9b), 1.893 (1H, dt, J = 13.5, 3.5 Hz, H-3a), 1.846 (1H, dq, J = 13.5, 3.5 Hz, H-2b), 1.567 (1H, td, J = 13.5, 3.5 Hz, H-3b), 1.375 (1H, dd, J = 12.3, 4.5 Hz, H-5), 1.341 (3H, s, H-14), 1.279 (3H, s, H-15), 0.959 (3H, d, J = 7.0 Hz, H-12), 0.956 (3H, d, J = 7.0 Hz, H-13).
2c. (500 MHz, pyridine-d5): δ 5.272 (1H, br s, H-8), 4.999 (1H, dd, J = 12.5, 3.5 Hz, H-1), 2.467 (1H, m, 9a), 2.472 (1H, m, H-6a), 2.277 (1H, qd, J = 13.5, 3.5, H-2a), 2.132 (1H, q, J = 7.0 Hz, H-11), 2.077 (1H, m, H-6b), 2.040 (1H, m, H-9b), 1.905 (1H, dt, J = 13.5, 3.5 Hz, H-3a), 1.866 (1H, dq, J = 13.5, 3.5 Hz, H-2b), 1.589 (1H, td, J = 13.5, 3.5 Hz, H-3b), 1.360 (1H, dd, J = 12.3, 4.5 Hz, H-5), 1.352 (3H, s, H-14),
1.247 (3H, s, H-15), 0.939 (3H, d, J = 7.0 Hz, H-12), 0.932 (3H, d, J = 7.0 Hz, H-13).
ACKNOWLEDGEMENTS
The authors would like to thank Mr. Do Kyun Kim, Dr. Eun Jung Bang and Dr. Jung Ju Seo at Korea Basic Science Institute for the measurements of NMR and MS spectra.
References
1. D. K. Ahn, Illustrated Book of Korean Medicinal Herbs, Koy-Hak Publishing Co., Korea, 1998, 607.
2. T. Nguyen, X. F. Cai, Q. Shen, S. I. Lee, E. J. Lee, K. Y. Park, K. H. Bae, and Y. H. Kim, Chem. Pharm. Bull., 2005, 53, 1194. CrossRef
3. T. Nguyen, P. V. Kien, X. F. Cai, Q. Shen, K. H. Bae, and Y. H. Kim, Arch. Pharm. Res., 2004, 27, 1106. CrossRef
4. J. Y. Park, B. S. Min, Y. J. Park, H. Y. Kim, H. K. Lee, and K. H. Bae, Chem. Pharm. Bull., 2002, 50, 685. CrossRef
5. H. J. Jung, B. S. Min, Y. J. Park, J. Y. Kim, Y. H. Lee, H. K. Lee, and K. H. Bae, J. Nat. Prod., 2002, 65, 897. CrossRef
6. M. J. Carvalho, L. M. Carvalho, A. M. Ferreira, and A. M. S. Silva, Nat. Prod. Res., 2003, 17, 445. CrossRef
7. L. S. Gan, Z. J. Zhan, S. P. Yang, and J. M. Yue, J. Asian. Nat. Prod. Res., 2006, 8, 589. CrossRef
8. C. Z. Wang, and D. Q. Yu, Phytochemistry, 1998, 48, 711. CrossRef
9. B. C. Yu, M. C. Yang, K.H. Lee, K. H. Kim, S. U. Choi, and K. R. Lee, Arch. Pharm, Res., 2007, 30, 1471. CrossRef
10. W. Schwab and P. Schreier, Phytochemistry, 1988, 27, 1813. CrossRef
11. A. S. Feliciano, M. Medarde, M. Gordaliza, E. D. Olmo, and J. M. M. D. Carral, Phytochemistry, 1989, 28, 2717. CrossRef
12. T. J. Ha, J. H. Lee, S. W. Hwang, J. Lee, N. S. Kang, K, Y, Oark, D. Y. Sah, K. H. Park, and M. S. Yang, Agric. Chem. Biotechnol., 2006, 49, 16.
13. S. J. Perkins, L. N. Johnson, and D. C. Phillips, Carbohydr. Res., 1977, 59, 19. CrossRef
14. T. Miyase, H. Ozaki, and A. Ueno, Chem. Pharm. Bull., 1991, 39, 937.
15. B. N. Su, E. J. Park, Z. H. Mbwambo, B. D. Santarsiero, A. D. Mesecar, H. H. S. Fong, J. M. Pezzuto. And A. D. Kinghorn, J. Nat. Prod. 2002, 65, 1278. CrossRef
16. H. J. Zhang, G. T. Tan, B. D. Santarsiero, A. D. Mesecar, N. V. Hung, N. M. Cuong, D. D. Soejarto, J. M. Pezzuto, and H. S. Fong, J. Nat. Prod., 2003, 66, 609. CrossRef
17. W. C. Su, J. M. Fang, and Y. S. Cheng, Phytochemistry, 1995, 39, 603. CrossRef
18. A. S. Feliciano, M. Medarde, M. Gordaliza, E. D. Olmo, and J. M. M. D. Carral, Phytochemistry, 1989, 28, 2717. CrossRef
19. Q. Ye, G. Qin, and W. Zhao, Phytochemistry, 2002, 61, 885 CrossRef