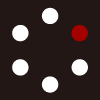
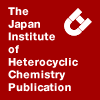
HETEROCYCLES
An International Journal for Reviews and Communications in Heterocyclic ChemistryWeb Edition ISSN: 1881-0942
Published online by The Japan Institute of Heterocyclic Chemistry
e-Journal
Full Text HTML
Received, 18th June, 2009, Accepted, 13th July, 2009, Published online, 15th July, 2009.
DOI: 10.3987/COM-09-11776
■ Crystal Structure and Intramolecular Hydrogen Bonding of a Substituted Diaminoquinoxaline
Junpei Kuwabara, Hironori Mori, and Takaki Kanbara*
Graduate School of Pure and Applied Sciences, Tsukuba Research Center for Interdisciplinary Materials Science, University of Tsukuba, 1-1-1 Ten-nodai, Tsukuba-shi, Ibaraki 305-8573, Japan
Abstract
A substituted diaminoquinoxaline, 2,3-diethyl-N5,N8-di(quinolin-8-yl)quinoxaline-5,8-diamine, was synthesized and characterized by a single-crystal X-ray diffraction study. The crystal structure of the molecule has a planar geometry with intramolecular hydrogen bonding. The hydrogen bonding is also evaluated in a solution state by spectroscopic techniques.In nature, intramolecular hydrogen bonding plays an important role in holding the secondary structure of peptides, such as α-helix and β-sheet.1 In terms of synthetic chemistry, intramolecular hydrogen bonding is a useful tool for constructing new structures.2 Although a hydrogen bond is much weaker than a covalent bond, multiple hydrogen bonds at appropriate positions provide a rigid structure for the target molecule.3 For example, intramolecular hydrogen bonding assists the construction of planar π-conjugated molecules, resulting in more enlarged π-conjugation than that in molecules without hydrogen bonding.4 It is also known that planar π-conjugated molecules are stacked in the solid state and exhibit conductivity along the stacking direction.5 To realize a new planar molecule using hydrogen bonding, we focused on a diaminoquinoxaline unit since diaminoquinoxaline derivatives have high potential for organic electronic materials6 and strong intramolecular hydrogen bonding between the amine proton and the imine nitrogen, NH···N=C.7 Hirao et al. have reported a series of diaminoquinoxaline derivatives and those small band gaps owing to donor-acceptor units.7 Herein, we report the synthesis of a substituted diaminoquinoxaline, 2,3-diethyl-N5,N8-di(quinolin-8-yl)quinoxaline-5,8-diamine, and its strong intramolecular hydrogen bonding in solid and solution states.
2,3-Diethyl-N5,N8-di(quinolin-8-yl)quinoxaline-5,8-diamine (1) was synthesized via a Pd-catalyzed C-N coupling reaction8 of 5,8-dibromo-2,3-diethylquinoxaline6a and 8-aminoquinoline (Scheme 1). The use of a bulky monodentate phosphine ligand, X-Phos,9 provides a higher yield (43%) than other phosphine ligands such as Xantphos10 (7%) and 2,2'-bis(diphenylphosphino)-1,1'-binaphthyl (BINAP) (0%). The product was characterized by 1H and 13C{1H} NMR, IR, HRMS, elemental analysis and a single-crystal X-ray diffraction study. Crystals suitable for X-ray diffraction study were grown by the slow diffusion of hexane into a CHCl3 solution of 1.
Figure 1 shows an ORTEP drawing of 1 showing its planar structure. The distances of NH···N=C (2.040-2.110 Å) are shorter than the sum of the van der Waals radii for H and N (2.75 Å), which strongly indicate intramolecular hydrogen bonding. The distances of the N-H bonds (1.012 and 1.081 Å) are relatively long compared with a normal N-H bond. The multiple hydrogen bonds are thought to elongate the N-H bond. The angles between the central quinoxaline ring and the quinoline rings are 3.19 and 5.64°, proving the planar geometry of the molecule (Figure 1b). The angles of C(2)-N(3)-C(13) and C(5)-N(4)-C(22) are 134.7(2) and 133.7(2)°, respectively. The relatively large angles are attributed to the hydrogen bonds and the steric repulsion of H(3)-H(15) and H(4)-H(21). In the crystal lattice, the planar molecules are stacked with a distance of 3.334 or 3.412 Å (Figure 1c). The distance is in the range of π-π stacking. The molecules are packed in the crystal lattice with hexagonal symmetry, which is an origin of R-3 space group. Bis(8-quinolinyl)amine has a similar bond parameter around the N-H group to that of 1 but with a longer NH···N=C distance (2.1691-2.1984 Å).11 Hirao et al. reported a diaminoquinoxaline with 4-phenylaminophenyl groups (2).7b The angles between the central quinoxaline ring and the adjacent phenyl rings are 10.29 and 23.46°, which are larger than those of 1. These results indicate that the multiple intramolecular hydrogen bonds are necessary to maintain the planar structure because of the steric repulsion.
The IR spectrum of 1 exhibits a broad absorption band of υ(N-H) at 3231 cm-1, which is much lower than that of N,N'-diphenyl-p-phenylenediamine (3390 cm-1) (Figure 2). The lower wavenumber is further evidence for hydrogen bonding in the solid state. In a solution state, 1 has also lower wavenumber of υ(N-H) (3343 cm-1) than that of N,N'-diphenyl-p-phenylenediamine (3431 cm-1).
To evaluate the strength of the hydrogen bonding in a solution state, 1H NMR spectra of 1 were measured at different temperatures and in various solvents (Figure 3). In CDCl3 at room temperature, the signal for the amine proton is observed at 10.4 ppm, whose position is at a much lower magnetic field than the amine signals of N,N'-diphenyl-p-phenylenediamine (5.55 ppm) and 2 (7.46 and 5.71 ppm). These results clearly show the existence of strong intramolecular hydrogen bonding in a solution state. The position of the amine signal is unchanged at a higher temperature (55 °C), indicating the high stability of the hydrogen bonds (Figure 3b). Interestingly, even in polar solvents such as DMSO-d6 and acetone-d6, the amine signal appears at a similar position to that in the nonpolar solvent CDCl3 (10.5 and 10.7 ppm, respectively). The hydrogen bonds are independent of the solvent, presumably due to the high stability of the multiple hydrogen bonds and the steric hindrance around the amine moiety, preventing the interaction of the solvent with the amine group.
Compound 1 was also characterized by cyclic voltammetry and UV-vis absorption spectroscopy. Compound 1 exhibits irreversible reduction and oxidation in acetonitrile. The electrochemical reduction of 1 occurred at Epc = -2.22 V (versus Ag/Ag+), whereas two oxidation peaks were observed at Epa = 0.17 and 0.58 V, respectively; the oxidation is considered to take place mainly at the two amino groups. The absorption maximum of 1 in acetonitrile is 409 nm (ε = 11000) with a shoulder at 452 nm (Figure 4), which indicates that 1 has a higher band gap than that of 2.
To summarize the present report, the intramolecular hydrogen bonding of 1 is stable in a solid and in solution, even at a high temperature and in polar solvents.
EXPERIMENTAL
General Experimental Procedures: 5,8-Dibromo-2,3-diethylquinoxaline was synthesized according to the literature method.6a 8-Aminoquinoline and other chemicals were purchased and used as received. Dehydrated toluene was purchased from Kanto Chemical and used as a dry solvent. NMR spectra were recorded on a JEOL EX-300 or a JEOL EX-270 NMR spectrometer. IR spectra were recorded on a JASCO FT/IR-300 spectrometer. Elemental analysis was carried out with a Perkin-Elmer 2400 CHN Elemental Analyzer. UV-vis spectrum was recorded on a JASCO V-630iRM spectrophotometer. Cyclic voltammogram was recorded on BAS 1200a electrochemical analyzer. Mass spectrum was recorded on JEOL JMS-700 MStation.
Synthesis of 2,3-diethyl-N5,N8-di(quinolin-8-yl)quinoxaline-5,8-diamine (1): A mixture of Pd2(dba)3 (22.9 mg, 0.025 mmol), X-phos (42.5 mg, 0.09 mmol), 5,8-dibromo-2,3-diethylquinoxaline (172.1 mg, 0.50 mmol), 8-aminoquinoline (216.3 mg, 1.5 mmol) and NaOtBu (320.4 mg, 4.0 mmol) in toluene (5 mL) was stirred for 3 days at 100 °C under nitrogen atmosphere. After cooling to rt, the reaction mixture is neutralized with 1M HCl. The organic layer was extracted with CHCl3 and washed with water and brine. The product was isolated by column chromatography on silica gel using CHCl3 as an eluent. Analytical pure sample was obtained by crystallization from CHCl3/hexane (101.3 mg, 43%). 1H NMR (300 MHz, CDCl3): δ 1.61 (t, J = 7.3 Hz, 6H, CH3), 3.14 (q, J = 7.3 Hz, 4H, CH2), 7.27 (m, 2H, Ar), 7.45 (t, J = 4.2 Hz, 2H, Ar), 7.50 (t, J = 8.0 Hz, 2H, Ar), 7.80 (d, J = 7.8 Hz, 2H, Ar), 7.85 (s, 2H, Ar), 8.15(dd, J = 8.3, 1.7 Hz, 2H, Ar), 8.90 (dd, J = 4.2, 1.6 Hz, 2H, Ar), 10.4 (s, 2H, NH). 13C{1H} NMR (68 MHz, CDCl3): δ 11.4, 27.8, 108.1, 111.2, 116.4, 121.4, 127.3, 128.8, 130.9, 133.3, 135.9, 139.6, 147.4, 147.5, 154.7. IR (KBr, cm-1): 3231, 2967, 1578, 1539, 1439, 1383, 1143, 1130, 810, 788, 772, 739, 599. HI-FAB MS: 470.2220 (Calcd. 470.2219). Anal. Calcd for C30H26N6·0.5H2O: C, 75.13; H, 5.67; N, 17.52. Found: C, 75.10; H, 5.58; N, 17.25%.
X-Ray Crystallography: Crystal of 1 suitable for X-ray diffraction study was obtained by recrystallization from CHCl3/hexane and mounted in glass capillary tube. The data for 1 were collected on a Rigaku Saturn CCD area detector. Calculations were carried out by using a program package CrystalStructure for Windows. The structure was solved by direct method and expanded using Fourier techniques. A full-matrix least-squares refinement was used for non-hydrogen atoms with anisotoropic thermal parameters. H1 and H2 were determined by difference Fourier map and refined isotropically. Other hydrogen atoms were placed at the calculated positions and were included in the structure calculation without further refinement of the parameters. Crystal data of 1: C30H26N6; Crystal size 0.3 x 0.1 x 0.1 mm; Mr, 470.58; trigonal; space group R-3 (No. 148); a 37.569(5), c 8.647(1) Å; V 10570(2) Å3; Z 18; µ (Mo-Kα) 0.815 cm-1; F (000) 4464; Dcalcd 1.331 g cm-3; unique reflections (2θ < 55°) 5345; number of variables 354; R 0.038 (I>2(I)); R 0.117 (All reflections); Rw 0.025 (All reflections); GOF 0.899. Crystallographic data have been deposited at the CCDC, 12 Union Road, Cambridge CB21EZ, UK and copies can be obtained on request, free of charge, by quoting the publication citation and the deposition number 734947.
ACKNOWLEDGEMENTS
This work was partly supported by Grant-in-Aid for Young Scientists (Start-up) (20850003) and Grant-in-Aid for Scientific Research (C) (20550105). The authors are grateful to the Chemical Analysis Center of University of Tsukuba for measurement of elemental analysis.
References
1. J. J. Dannenberg, Adv. Protein Chem., 2005, 72, 227. CrossRef
2. a) Z. Li, J. Hou, and C. Li, Acc. Chem. Res., 2008, 41, 1343; CrossRef b) B. Gong, Acc. Chem. Res., 2008, 41, 1376; CrossRef c) J. Garric, J.-M. Leger, and I. Huc, Angew. Chem. Int. Ed., 2005, 44, 1954; CrossRef d) C. Li, G. Wang, H. Yi, X. Jiang, Z. Li, and R. Wang, Org. Lett., 2007, 9, 1979. CrossRef
3. a) W. Zhang and J. S. Moore, Angew. Chem., Int. Ed., 2006, 45, 4416; CrossRef b) Z. Li, J. Hou, C. Li, and H. Yi, Chem. Asian J., 2006, 1, 766; CrossRef c) L. Yuan, W. Feng, K. Yamato, A. R. Sanford, D. Xu, H, Guo, and B. Gong, J. Am. Chem. Soc., 2004, 126, 11120; CrossRef d) F. Li, Q. Gan, L. Xue, Z. Wanga, and H. Jiang, Tetrahedron Lett., 2009, 50, 2367. CrossRef
4. a) D. A. P. Delnoye, R. P. Sijbesma, J. A. J. M. Vekemans, and E. W. Meijer, J. Am. Chem. Soc., 1996, 118, 8717; CrossRef b) M. Vetrichelvan and S. Valiyaveettil, Chem. Eur. J., 2005, 11, 5889; CrossRef c) Y. Tian and M. Kertesz, Macromolecules, 2009, 42, 2309. CrossRef
5. G. Saito and Y. Yoshida, Bull. Chem. Soc. Jpn., 2007, 80, 1. CrossRef
6. a) T. Kanbara and T. Yamamoto, Macromolecules, 1993, 26, 3464; CrossRef b) T. Yamamoto, K. Sugiyama, T. Kushida, T. Inoue, and T. Kanbara, J. Am. Chem. Soc., 1996, 118, 3930; CrossRef c) T. Yamamoto, Z. Zhou, T. Kanbara, M. Shimura, K. Kizu, T. Maruyama, Y. Nakamura, T. Fukuda, B. Lee, N. Ooba, S. Tomaru, T. Kurihara, T. Kaino, K. Kubota, and S. Sasaki, J. Am. Chem. Soc., 1996, 118, 10389; CrossRef d) H. Son, W. Han, D. Yoo, K. Min, S. Kwon, J. Ko, and S. O. Kang, J. Org. Chem., 2009, 74, 3175. CrossRef
7. a) M. T. S. Ritonga, H. Sakurai, and T. Hirao, Tetrahedron Lett., 2002, 43, 9009; CrossRef b) H. Sakurai, M. T. S. Ritonga, H. Shibatani, and T. Hirao, J. Org. Chem., 2005, 70, 2754; CrossRef c) M. T. S. Ritonga, H. Shibatani, H. Sakurai, T. Moriuchi, and T. Hirao, Heterocycles, 2006, 68, 829. CrossRef
8. a) A. R. Muci and S. L. Buchwald, Top. Curr. Chem., 2002, 219, 131; CrossRef b) J. F. Hartwig, Acc. Chem. Res., 2008, 41, 1534. CrossRef
9. X-phos: 2-Dicyclohexylphosphino-2',4',6'-triisopropylbiphenyl. X-phos is an effective ligand for a C-N coupling reaction of heteroaromatic compounds. J. Kuwabara, H. Mori, T. Teratani, M. Akita, and T. Kanbara, Macromol. Chem. Rapid Commun., 2009, 30, 997. CrossRef
10. Xantphos: 4,5-Bis(diphenylphosphino)-9,9-dimethylxanthene.
11. D. Maiti, H. Paul, N. Chanda, S. Chakraborty, B. Mondal, V. G. Puranik, and G. K. Lahiri, Polyhedron, 2004, 23, 831. CrossRef